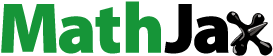
ABSTRACT
Background
Caffeine, widely recognized as an ergogenic aid, has undergone extensive research, demonstrating its effectiveness to enhance endurance performance. However, there remains a significant gap in systematically evaluating its effects on time trial (TT) performance in cyclists.
Purpose
This meta-analysis aimed to determine the efficacy of caffeine ingestion to increase cycling TT performance in cyclists and to evaluate the optimal dosage range for maximum effect.
Methods
A search of four databases was completed on 1 December 2023. The selected studies comprised crossover, placebo-controlled investigations into the effects of caffeine ingestion on cycling TT performance. Completion time (Time) and mean power output (MPO) were used as performance measures for TT. Meta-analyses were performed using a random-effects model to assess the standardized mean differences (SMD) in individual studies.
Results
Fifteen studies met the inclusion criteria for the meta-analyses. Subgroup analysis showed that moderate doses of caffeine intake (4–6 mg/kg) significantly improved cycling performance (SMD Time = -0.55, 95% confidence interval (CI) = -0.84 ~ -0.26, p < 0.01, I2 = 35%; SMD MPO = 0.44, 95% CI = 0.09 ~ 0.79, p < 0.05, I2 = 39%), while the effects of low doses (1–3 mg/kg) of caffeine were not significant (SMD Time = -0.34, 95% CI = -0.84 ~ 0.17, p = 0.19, I2 = 0%; SMD MPO = 0.31, 95% CI = -0.02 ~ 0.65, p = 0.07, I2 = 0%).
Conclusion
A moderate dosage (4–6 mg/kg) of caffeine, identified as the optimal dose range, can significantly improve the time trial performance of cyclists, while a low dose (1–3 mg/kg) does not yield improvement. In addition, the improvements in completion time and mean power output resulting from a moderate dose of caffeine are essentially the same in cycling time trails.
1. Introduction
A mere 1% change in average speed can significant impact medal rankings in highly competitive Olympic endurance events [Citation1]. For instance, during the 2016 Athens Olympics, the champion and runner-up in the team pursuit cycling were separated by just 0.88% in average speed [Citation1]. In the men’s individual road race, the top three athletes exhibited a performance gap of less than 0.01% [Citation2]. Given the growing competitiveness in cycling, athletes and coaches continuously seek advantages to maximize their chances of winning by optimizing training methods and/or incorporating sports supplements.
Caffeine, with its convenience and rapid metabolism, has become one of the most popular supplements among athletes [Citation3–5]. Previous meta-analyses have explored the effects of caffeine ingestion on multiple types of exercise performance, such as endurance exercise [Citation1,Citation6–9], team sports [Citation10,Citation11], and resistance exercise [Citation12,Citation13]. The general consensus from these studies is that caffeine can improve endurance exercise performance [Citation1,Citation6–9,Citation14–20].
The meta-analysis exploring the effects of caffeine on endurance performance primarily focuses on two testing protocols, namely time trial (TT) and time to exhaustion (TTE) [Citation6–9,Citation20]. Nevertheless, being a “closed-loop” performance test, TT allows athletes to self-pace and adjust their efforts, simulating the real conditions and characteristics of cycling races unlike “open-loop” tests such as TTE [Citation21]. Additionally, the inclusion criteria in the aforementioned meta-analysis addressing the effect of caffeine on endurance TT performance were often not sufficiently rigorous, resulting in several issues including the inclusion of (1) multiple sports events (i.e. swimming, cycling, rowing, running) [Citation7,Citation8], athletes (i.e. swimmers, cyclists, rowers, runners) [Citation7,Citation8], and forms of caffeine intake (i.e. gum, capsules, liquid) [Citation20], leading to significant research heterogeneity; and (2) co-ingestion of other substances [Citation9], making it difficult to determine the independent effects of caffeine. Furthermore, numerous recent studies have investigated the effect of caffeine intake on TT performance in cyclists, with inconsistent findings [Citation22–26]. Therefore, a meta-analysis is needed to explore the effect of caffeine intake on cyclists’ TT performance and provide clarity on the optimal caffeine dosage range.
This meta-analysis aims to review recent studies that investigated caffeine’s ergogenic effects on TT performance in cyclists. Our intention is to quantitatively analyze these studies to gauge the extent of caffeine’s ergogenic influence on cycling TT performance and identify the optimal dosage. Consequently, we will offer practical guidance for cyclists and coaches seeking to enhance athletic performance through caffeine consumption.
2. Materials and methods
This meta-analysis was conducted following the recommendations of the Preferred Reporting Items for Systematic Reviews and Meta-Analyses (PRISMA) and has been registered in the International System Review Prospective Register (PROSPERO) (CRD42022358446) [Citation27].
2.1. Literature search
We conducted a literature search in PubMed, Web of Science, EMBASE and CNKI (China National Knowledge Infrastructure) databases using the following keywords: caffeine, coffee, caffeinated, cycling, cyclist, exercise, performance, time trial. We employed Boolean search syntax (“AND,” “OR,” “NOT”) and tailored our search strategies to each database’s characteristics. Considering the sustained interest in caffeine and exercise performance over the past two decades, relevant studies from January 2002 to December 2023 were retrieved. When necessary, we contacted the corresponding authors via e-mail to obtain missing data. Finally, to avoid missing any relevant literature, manual searches were conducted using Google Scholar and Scopus.
2.2. Selection criteria
Inclusion criteria were based on PICOS (participants, interventions, comparators, outcomes, and study design) and included studies that: (1) involved cyclists as participants; (2) examined the effects of isolated caffeine ingestion on TT performance; (3) included a placebo group as a comparator; (4) used completion time (Time) and mean power output (MPO) as evaluation indices of TT performance; (5) utilized a single or double-blind placebo-controlled crossover design. Exclusion criteria were applied to studies that: (1) involved caffeine consumption during the exercise protocol; (2) grouped participants by genotype with different caffeine sensitivity; (3) utilized energy drinks during exercise; (4) conducted studies in extreme environments (high altitude, high or low temperature); (5) consumed caffeine in the form of gum due to its faster absorption rate (5–10 vs. 45–60 min) and differing bioavailability compared to capsules and liquid [Citation28]. Two investigators (B.C. and L.D.) independently selected eligible articles based on the title, abstract and full paper, adhering to the inclusion criteria. For the included studies, the same reviewers independently collected data for use in the meta-analysis.
2.3. Study coding and data extraction
Data extraction was performed to obtain information about participants, interventions, comparisons, outcomes, and study findings. Specifically, the following data were extracted from the included studies: (1) author(s), title, and year of publication; (2) participants’ characteristics [e.g. age, maximal oxygen uptake (O2max)], habitual caffeine intake); (3) caffeine dose and timing of ingestion; (4) TT performance protocol; (5) main study findings.
2.4. Assessment of methodological quality and statistical analyses
The methodological quality and risk of bias in all eligible studies were assessed according to the criteria outlined in the Cochrane guideline [Citation29]. The Cochrane risk of bias assessment tool evaluates studies for biases, including: (1) random sequence generation; (2) allocation concealment; (3) blinding of participants and personnel; (4) blinding of outcome assessment; (5) incomplete outcome data; (6) selective reporting; (7) other bias. Ratings for each of category are denoted as either “low risk” (“+”), “high risk” (“-”) or “unclear risk” (“?”). Two reviewers (D.L. and C.B.) independently conducted these assessments using Review Manager 5.4 software (Copenhagen: The Nordic Cochrane Center, The Cochrane Collaboration, 2014). Any disagreements regarding quality and risk of bias were resolved through discussion or by a third reviewer (C.Y.).
To gauge the effect of caffeine intake on TT performance, we employed a random-effects model that assigned weights to the selected studies based on their standard deviations, which allowed us to calculate the weighted-mean standardized mean differences (SMD). TT performance was measured in two ways: MPO within a set duration completion time for a specific athletic event. We conducted a meta-analysis utilizing Review Manager 5.4 software. Results are presented as P-value, SMD, and 95% confidence interval (CI). An ergogenic effect was considered when the time to complete a set performance decreased with caffeine compared to placebo, or when MPO in a set TT increased with caffeine compared to placebo. Effect sizes were classified as trivial (SMD <0.20), small (0.20 ≤ SMD < 0.50), moderate (0.50 ≤ SMD < 0.80), or large (SMD ≥0.80) according to established criteria [Citation30].
We assessed heterogeneity among the included studies using the I2 statistic as recommended by the Cochrane Collaboration. Heterogeneity levels were categorized as low (I2 <25%), moderate (25% ≤ I2 ≤50%), and high (I2 >50%) [Citation31]. Subgroup analyses were conducted when there were at least three studies to explore potential sources of heterogeneity [Citation30]. Meta-regression analysis was conducted using Stata 14.0 software to examine how factors such as O2max, age, and exercise duration affected performance outcomes in TT events for both caffeine and placebo. Funnel plots, Egger’s regression, and trim-and-fill tests were employed to assess publication bias.
3. Results
3.1. Study characteristics
Out of 1,749 initially identified studies, we removed duplicates and conducted title and abstract screening (). After applying our criteria, we selected 15 articles with a total of 35 effect sizes for quantitative analysis [Citation22–26,Citation32–41].
presents the characteristics of all the included studies. A total of 14 studies investigated the effects of caffeine on Time, comprising 18 trials [Citation22–24,Citation26,Citation32–41], while 13 studies examined the effects of caffeine on MPO, comprising 17 trials [Citation22–26,Citation33–37,Citation39–41]. The studies that provided caffeine doses relative to body weight had doses ranging from 1–6 mg/kg. One study used an absolute dose of 200 mg, which is equivalent to a relative dose of 2.5 mg/kg [Citation26]. Fourteen studies administered caffeine supplementation 60 minutes prior to exercise [Citation22–26,Citation32–41], while one study had a scheme of caffeine ingestion 90 minutes before exercise [Citation37]. One study initiated exercise following individual peak serum caffeine concentrations [Citation36]. None of the studies reported commercial sponsorship.
Table 1. General characteristics of the studies included.
3.2. Quality of study methods
The risk of bias assessment was conducted for the 15 included placebo-controlled crossover trials (). Three trials had a low risk of bias, while 12 were deemed to have a moderate risk of bias. Firstly, a slight publication bias may exist in terms of performance outcomes in TT (Time and MPO), as indicated by the funnel plots (). However, funnel plots are subjective inspection methods, and further analysis is required by combining Egger’s regression intercept test. Secondly, based on the numerical values of Egger’s regression intercept, the Egger’s linear regression results for performance outcomes in TT showed a significant difference from zero (p < 0.05), suggesting the presence of publication bias. Finally, after conducting trim and fill analysis to assess the impact of publication bias, the overall effects for performance outcomes in TT remained significant, indicating that the study results are not significantly influenced by publication bias and can be considered reliable ().
Figure 3. Funnel plot of standard mean difference against standard error for completion time. SE (SMD) Standard error of the mean difference, SMD Standard mean difference.
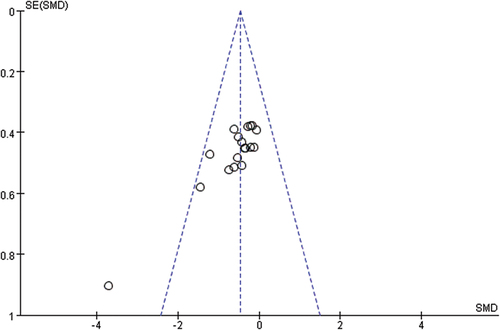
Figure 4. Funnel plot of standard mean difference against standard error for mean power output. SE (SMD) Standard error of the mean difference, SMD Standard mean difference.
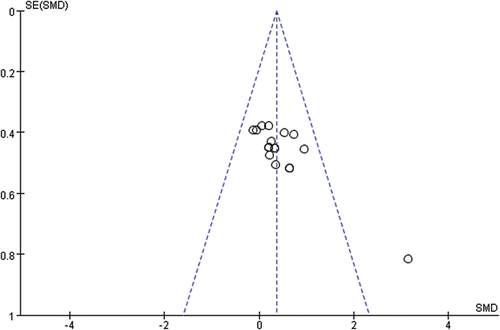
Table 2. Duval and Tweedie’s trim and fill random effects of time trial results.
3.3. Meta-analysis results
Fourteen placebo-controlled crossover studies, encompassing 18 trials, examined the effect of caffeine on Time () [Citation22–24,Citation26,Citation32–41]. There was a significant effect of caffeine ingestion (1–6 mg/kg) on Time compared to placebo (SMD = −0.50, 95% CI = −0.74 ~ −0.26, p < 0.001, I2 = 23%). Three trials involving 62 participants (31 in the caffeine group and 31 in the placebo group) assessed the effect of a low dose (1–3 mg/kg) of caffeine on Time [Citation26,Citation33,Citation37], while 15 trials with 322 participants (161 in the caffeine group and 161 in the placebo group) evaluated the effect of a moderate dose (4–6 mg/kg) of caffeine on Time [Citation22–24,Citation32,Citation34–36,Citation38–41] (). Subgroup analysis indicated that moderate dose of caffeine ingestion (4–6 mg/kg) resulted in a significant improvement in Time (SMD = −0.55, 95% CI = −0.84 ~ −0.26, p < 0.01, I2 = 35%) (). However, the improvement in Time due to caffeine was not significant when a low dose caffeine (1–3 mg/kg) was administered (SMD = −0.34, 95% CI = −0.84 ~ 0.17, p > 0.05, I2 = 0%) ().
Figure 5. Subgroup analysis of the effects of different caffeine doses intake on time trial performance (completion time) of cyclists. “a”, “b,” “c” represents the number of trials of the same study. Filled green square represents study-specific estimates, and filled diamond represents pooled estimates of random-effects. Std Mean Difference Standard mean difference, CI confidence interval, SD standard deviation.
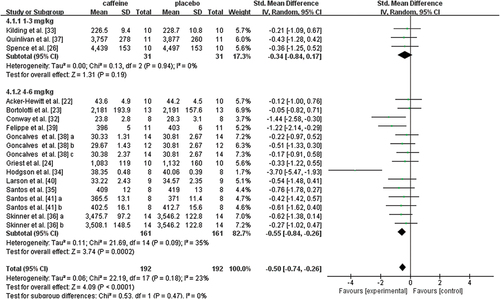
Thirteen placebo-controlled crossover studies, encompassing 17 trials, examined the effect of caffeine on MPO [Citation22–26,Citation33–37,Citation39–41] (). There was also a significant effect of caffeine ingestion (1–6 mg/kg) on MPO compared to placebo (SMD = 0.37, 95% CI = 0.14 ~ 0.60, p = 0.002, I2 = 16%). Six trials, involving 140 participants (70 in the caffeine group and 70 in the placebo group), assessed the effect of a low dose (1–3 mg/kg) of caffeine on MPO [Citation25,Citation26,Citation33,Citation37]. Additionally, 11 trials with 226 participants (113 in the caffeine group and 113 in the placebo group) evaluated the effect of a moderate dose (4–6 mg/kg) of caffeine on MPO [Citation22–24,Citation34–36,Citation39–41] (). Subgroup analysis showed that moderate doses of caffeine intake resulted in a significant improvement in MPO (SMD = 0.44, 95% CI = 0.09 ~ 0.79, p < 0.05, I2 = 39%) (). However, the improvement of caffeine on MPO due to caffeine was not significant when a low dose was administered (SMD = 0.31, 95% CI = −0.02 ~ 0.65, p = 0.07, I2 = 0%) ().
Figure 6. Subgroup analysis of the effects of different caffeine doses intake on time trial performance (mean power output) of cyclists. “a”, “b,” “c” represents the number of trials of the same study. Filled green square represents study-specific estimates, and filled diamond represents pooled estimates of random-effects. Std Mean Difference Standard mean difference, CI confidence interval, SD standard deviation.
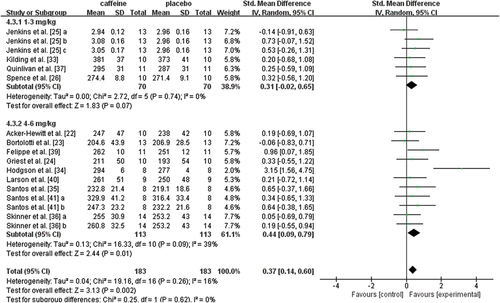
Meta-regression analysis showed no significant relationship between the effect size of both Time and MPO, and the independent variables: O2max (P MPO = 0.523; P Time = 0.266), age (P MPO = 0.199; P Time = 0.263), and exercise duration (P MPO = 0.932; P Time = 0.535) ().
Table 3. Meta-regression analysis of time trial results.
4. Discussion
The aim of this systematic review and meta-analysis was to evaluate the effect of caffeine intake on TT performance in cyclists and determine the optimal dosage range for most pronounced ergogenic effect. We found that a moderate dose (4–6 mg/kg) of caffeine intake significantly improves the TT performance of cyclists (SMD Time = −0.55, p < 0.01; SMD MPO = 0.44, p < 0.05). Conversely, lower doses (1–3 mg/kg) of caffeine do not result in performance improvement (SMD Time = −0.34, p = 0.19; SMD MPO = 0.31, p = 0.07).
4.1. Effects of low to moderate dose of caffeine intake on cycling TT performance
Our results indicate that a moderate caffeine dose can improve the TT performance of cyclists (). Our results are consistent with a recent meta-analysis that incorporated various endurance sports (i.e. swimming, cycling, running, double poling and triathlon), which also suggested that a moderate caffeine dose significantly enhances TT performance [Citation8]. However, the overall effect sizes in their study were slightly lower than what we observed (SMD Time: 0.41 vs. 0.55; SMD MPO: 0.24 vs. 0.44). This difference could be attributed to the inclusion of different endurance events and exercise in high-temperature environments (~30℃) in their meta-analysis [Citation8,Citation42], potentially reducing the overall effect size. Considering the previously mentioned study conducted under high-temperature conditions, it is noteworthy that caffeine failed to enhance TT performance, as indicated by SMD = −0.24 [Citation42]. Additionally, a systematic review examining the effect of caffeine on TT performance in rowing, cycling, running, swimming and skiing, also suggested that a moderate caffeine dose is likely to offer the greatest improvement in TT performance compared to low or high doses of caffeine [Citation15]. Our study reaffirms this conclusion, suggesting that compared to a low dose (1–3 mg/kg) a moderate dose of caffeine (4–6 mg/kg) enhances cyclists’ TT performance (). This is evident in the greater effect size and mean improvement (SMD Time: 0.55 vs. 0.34; SMD MPO: 0.44 vs. 0.31) (Time: 3.2 ± 3.72% vs. 1.78 ± 1.15%; MPO: 4.33 ± 1.67% vs. 2.08 ± 1.67%). However, a meta-analysis focusing on the effect of caffeine on endurance performance found that the ergogenic effect of caffeine is not significantly dose-dependent [Citation9]. This discrepancy could be due to the low dosage (1–3 mg/kg) of caffeine included in that meta-analysis and the administration of caffeine through energy drinks, which may contain other substances that might interfere with the effects of caffeine.
The larger ergogenic effect of moderate-dose caffeine, compared to low-dose caffeine, on TT performance may be attributed to an increased number of adenosine receptors in cyclists. Caffeine’s antagonistic effect on adenosine receptors is a well-established mechanism through which it influences endurance performance [Citation43–47]. Firstly, recent research indicates that endurance athletes who engage in long-term training exhibit adaptive changes to cope with exercise-induced stress, thereby increasing the number of adenosine receptors [Citation48]. Secondly, we found that participants in both the low-dose group (167 ± 70 mg/day) and the moderate-dose group (221 ± 112 mg/day) had a moderate caffeine consumption habit (75–300 mg/day) [Citation49]. Habitual caffeine intake can also enhance athletes’ tolerance to caffeine by increasing the number of adenosine receptors [Citation50,Citation51], thereby reducing the ergogenic effect of caffeine on athletic performance [Citation52,Citation53]. Overall, a moderate dosage above 3 mg/kg may be required to effectively block the increased number of adenosine receptors in cyclists, thereby improving TT performance.
4.2. Effects of moderate caffeine dose intake on time and MPO in cycling TT
We observed that both Time and MPO, which are commonly used indicators in cycling TT, showed comparable improvements compared to placebo group (Time: +3.2 ± 3.7%, MPO: +4.3 ± 1.7%). These results are consistent with a meta-analysis that investigated the effects of moderate-dose caffeine on Time and MPO among athletes participating in various endurance events (Time: +2.2 ± 2.6%, MPO: +2.9 ± 2.2%). The comparable improvements in Time and MPO due to caffeine in our study align with research suggesting that a 1% change in power output roughly corresponds to a 1% change in Time [Citation54]. However, another meta-analysis found a more substantial improvement in endurance performance (+22.3% ± 13.3%) in cycling tests compared to placebo trials, which is substantially larger than our observed effects [Citation6]. This discrepancy could be attributed to their inclusion of TTE measurement protocols, where a 1% change in output power leads to a 15% change in Time [Citation54].
4.3. Heterogeneity analysis of moderate-dose caffeine effects on cycling TT performance
Heterogeneity analysis of moderate caffeine dosage on Time and MPO in the included studies showed that I2 were 35% and 39%, respectively. These values indicate a moderate level of internal heterogeneity in the research results. In contrast, a meta-analysis focusing on the ergogenic effects of moderate-dose caffeine on endurance TT performance revealed a larger level of heterogeneity within the studies (I2 = 63%) [Citation8]. This disparity may be attributed to the broader inclusion of different endurance events (i.e. swimming, cycling, running, double poling and triathlon) in the aforementioned meta-analysis [Citation8]. To explore potential sources of heterogeneity, we conducted a meta-regression analysis. The results are consistent with other meta-analyses, indicating that age (24–41 years), O2max (49–72 ml/min/kg), and exercise duration (3.8–72 min) do not significantly influence performance improvement observed between caffeine and placebo [Citation6–8]. However, given that the studies included in our analysis shared similar characteristics in terms of these variables, future research should consider broadening the age range, incorporating world-class athletes, and including longer competition durations to further elucidate the impact of these variables on the ergogenic effects of caffeine. Additionally, genetic variations have been found to influence the ergogenic effects of caffeine on athletic performance, which could contribute to the observed heterogeneity in our research [Citation8,Citation55]. Research indicates that individuals carrying the AA genotype exhibit faster caffeine metabolism and higher sensitivity to caffeine compared to individuals with the CC or AC genotypes [Citation56–59]. Finally, other factors such as gender, cigarette smoking, alcohol intake, and the use of hormonal contraceptives may also serve as potential sources of heterogeneity that warrant further exploration [Citation60–62].
5. Practical implications
Our result indicates that a moderate caffeine dosage (4–6 mg/kg) leads to a 2% higher improvement in TT performance compared to a low dosage (1–3 mg/kg). This 2% advantage holds practical relevance, as it could potentially elevate an 8th place finisher’s time (57:18) in the men’s cycling TT at the 2021 Tokyo Olympics to a top-three finish (56:08) [Citation2]. Existing research has demonstrated that a moderate caffeine dose generally does not produce significant side effects such as gastrointestinal discomfort, lack of concentration, or accelerated fluid loss [Citation18,Citation63,Citation64]. In addition, individuals exhibit variability in their response to caffeine`s ergogenic effects [Citation15,Citation18,Citation19], and an athlete’s specific caffeine needs entail a careful balance between maximizing the dose for a meaningful physiological stimulus and preserving overall well-being by avoiding potential side effects. Hence, we recommend that practitioners focus on identifying the optimal caffeine dose for each individual athletes through a series of trials conducted before official competitions. This personalized approach can help ensure that athletes harness the performance-enhancing benefits of caffeine without compromising their health and comfort during competition events.
6. Limitations and future considerations
Although this systematic review rigorously examined the existing literature and strictly adhered to the PRISMA guidelines, there are certain limitations that should be considered [Citation27]. First, our analysis did not include factors such as dietary habit (alcohol and cigarettes), gender, and genetic type, which have been proven to influence the metabolic effects of caffeine [Citation18,Citation60,Citation61]. Future research should take these variables into account to better understand the effects of caffeine on cyclists. Second, our study primarily focused on the effects of caffeine on MPO and Time in cycling TT, omitting investigations into various physiological and psychological indicators such as blood lactate concentration, heart rate, and ratings of perceived fatigue. Incorporating these additional metrics in future studies could provide valuable insights into the ergogenic effects of caffeine among cyclists. Third, cyclists often consume caffeine in combination with other substances during competitions [Citation1]. Therefore, future research should explore the differences in effects resulting from different co-intake strategies. Last, the small number of studies included in the low-dose group analyses may limit our ability to draw definitive conclusions from the obtained outcomes.
7. Conclusion
This systematic review and meta-analysis showed that a moderate dosage (4–6 mg/kg) of caffeine, identified as the optimal dose range, significantly improves the time trial performance of cyclists. Contrastingly, a low dose (1–3 mg/kg) does not yield the same improvement. In addition, the improvements in completion time and mean power output due to a moderate dose of caffeine are essentially equivalent in cycling time trials. These findings could provide a basis for cyclists and coaches to devise more effective caffeine supplementation strategies. However, when tailoring personalized supplement regimens, it is essential to consider individual athlete differences (i.e. gender, dietary habits, and genetic types) to maximize caffeine’s ergogenic effects.
Author contributions
Original idea: Y.C.; Development and formulation of concept: Y.C., B.C., L.D., T.L., Q.Q., & O.G.; Draft: L.D., B.C., Y.C.; Critical revision: Y.C., T.L., & O.G. All authors read and approved the final version of the manuscript.
Acknowledgments
The authors express their gratitude to all participants. This study would not have been possible without the unwavering commitment, valuable time, and diligent effort of our participants.
Disclosure statement
No potential conflict of interest was reported by the author(s).
Data availability statement
Data are available on request from the authors
Additional information
Funding
References
- Christensen PM, Shirai Y, Ritz C, et al. Caffeine and bicarbonate for speed. A meta-analysis of legal supplements potential for improving intense endurance exercise performance. Front Physiol. [2017 May 9];8. doi: 10.3389/fphys.2017.00240
- Olympic.org. Rio 2016 cycling road 2023. Available from: https://olympics.com/
- Caraballo I, Dominguez R, Guerra-Hernandez EJ, et al. Analysis of sports supplements consumption in young Spanish elite dinghy sailors. Nutrients. 2020 Apr;12(4). doi: 10.3390/nu12040993
- Sanchez-Oliver AJ, Grimaldi-Puyana M, Dominguez R. Evaluation and behavior of Spanish bodybuilders: doping and sports supplements. Biomolecules. 2019 Apr;9(4). doi: 10.3390/biom9040122
- Comes AV, Sanchez-Oliver AJ, Martinez-Sanz JM, et al. Analysis of nutritional supplements consumption by squash players. Nutrients. 2018 Oct;10(10). doi: 10.3390/nu10101341
- Doherty M, Smith PM. Effects of caffeine ingestion on exercise testing: a meta-analysis. Int J Sport Nutr Exerc Metab. 2004 Dec;14(6):626–18. doi: 10.1123/ijsnem.14.6.626
- Shen JG, Brooks MB, Cincotta J, et al. Establishing a relationship between the effect of caffeine and duration of endurance athletic time trial events: a systematic review and meta-analysis. J Sci Med Sport. 2019 Feb;22(2):232–238. doi: 10.1016/j.jsams.2018.07.022
- Southward K, Rutherfurd-Markwick KJ, Ali A. The effect of acute caffeine ingestion on endurance performance: a systematic review and meta-analysis (vol 48, pg 1913, 2018). Sports Med. 2018 Oct;48(10):2425–2441. doi: 10.1007/s40279-018-0967-4
- Souza DB, Del Coso J, Casonatto J, et al. Acute effects of caffeine-containing energy drinks on physical performance: a systematic review and meta-analysis. Eur J Nutr. 2017 Feb;56(1):13–27. doi: 10.1007/s00394-016-1331-9
- Salinero JJ, Lara B, Del Coso J. Effects of acute ingestion of caffeine on team sports performance: a systematic review and meta-analysis. Res Sports Med. 2019 Apr-Jun;27(2):238–256. doi: 10.1080/15438627.2018.1552146
- José J, Salinero B, Del Coso J. Effects of acute ingestion of caffeine on team sports performance: a systematic review and meta-analysis. Res Sports Med. 2019;27(2):238–256. doi: 10.1080/15438627.2018.1552146
- Grgic J, Del Coso J. Ergogenic effects of acute caffeine intake on muscular endurance and muscular strength in women: a meta-analysis. Int J Environ Res Public Health. [2021 May 27];18(11):5773. doi: 10.3390/ijerph18115773
- Grgic J, Trexler ET, Lazinica B, et al. Effects of caffeine intake on muscle strength and power: a systematic review and meta-analysis. J Int Soc Sports Nutr. 2018;15(1):11. doi: 10.1186/s12970-018-0216-0
- Desbrow B, Biddulph C, Devlin B, et al. The effects of different doses of caffeine on endurance cycling time trial performance. J Sports Sci. 2012;30(2):115–120. doi: 10.1080/02640414.2011.632431
- Ganio MS, Klau JF, Casa DJ, et al. Effect of caffeine on sport-specific endurance performance: a systematic review. J Strength Cond Res. 2009 Jan;23(1):315–324. doi: 10.1519/JSC.0b013e31818b979a
- Graham-Paulson T, Perret C, Goosey-Tolfrey V. Improvements in cycling but not handcycling 10 km time trial performance in habitual caffeine users. Nutrients. 2016 Jul;8(7). doi: 10.3390/nu8070393
- Grgic J, Diaz-Lara FJ, Del Coso J, et al. The effects of caffeine ingestion on measures of rowing performance: a systematic review and meta-analysis. Nutrients. 2020 Feb;12(2). doi: 10.3390/nu12020434
- Guest NS, VanDusseldorp TA, Nelson MT, et al. International society of sports nutrition position stand: caffeine and exercise performance. J Int Soc Sports Nutr. [2021 Jan 2];18(1):1. doi: 10.1186/s12970-020-00383-4
- Higgins S, Straight CR, Lewis RD. The effects of preexercise caffeinated coffee ingestion on endurance performance: an evidence-based review. Int J Sport Nutr Exerc Metab. 2016 Jun;26(3):221–239. doi: 10.1123/ijsnem.2015-0147
- Wang Z, Qiu B, Gao J, et al. Effects of caffeine intake on endurance running performance and time to exhaustion: a systematic review and meta-analysis. Nutrients. [2022 Dec 28];15(1):148. doi: 10.3390/nu15010148
- Laursen PB, Francis GT, Abbiss CR, et al. Reliability of time-to-exhaustion versus time-trial running tests in runners. Med Sci Sports Exerc. 2007 Aug;39(8):1374–1379. doi: 10.1249/mss.0b013e31806010f5
- Acker-Hewitt TL, Shafer BM, Saunders MJ, et al. Independent and combined effects of carbohydrate and caffeine ingestion on aerobic cycling performance in the fed state. Appl Physiol Nutr Metab. 2012 Apr;37(2):276–283. doi: 10.1139/h11-160
- Bortolotti H, Altimari LR, Vitor-Costa M, et al. Performance during a 20-km cycling time-trial after caffeine ingestion. J Int Soc Sports Nutr. 2014;11(1):45. doi: 10.1186/s12970-014-0045-8
- Griest TD, Saunders MJ, Womack CJ, et al. Caffeine enhances 10-km cycling performance in habitual users only when preceded by caffeine abstinence. Int J Sports Physiol Perform. 2023 Aug;18(8):805–812. doi: 10.1123/ijspp.2022-0350
- Jenkins DG, Trilk JL, Singhal A. Ergogenic effects of low doses of caffeine on cycling performance. Int J Sport Nutr Exercise Metab. 2008;18(3):328–342. doi: 10.1123/ijsnem.18.3.328
- Spence AL, Sim M, Landers G, et al. A comparison of caffeine versus pseudoephedrine on cycling time-trial performance. Int J Sport Nutr Exercise Metab. 2013 Oct;23(5):507–512. doi: 10.1123/ijsnem.23.5.507
- Moher D, Shamseer L, Clarke M, et al. Preferred reporting items for systematic review and meta-analysis protocols (PRISMA-P) 2015 statement. Syst Rev. [2015 Jan 1];4(1):1. doi: 10.1186/2046-4053-4-1
- Kamimori GH, Karyekar CS, Otterstetter R, et al. The rate of absorption and relative bioavailability of caffeine administered in chewing gum versus capsules to normal healthy volunteers. Int J Pharm. [2002 Mar 2];234(1–2):159–167. doi: 10.1016/S0378-5173(01)00958-9
- Cohen J. Statistical power analysis for the behavioral sciences. Burlington: Elsevier Science; 2013. English.
- Cumpston M, Li T, Page MJ, et al. Updated guidance for trusted systematic reviews: a new edition of the Cochrane handbook for systematic reviews of interventions. Cochrane Database Syst Rev. [2019 Oct 3];10(10):ED000142. doi: 10.1002/14651858.ED000142
- Higgins JP, Thompson SG. Quantifying heterogeneity in a meta-analysis. Stat Med. [2002 Jun 15];21(11):1539–1558. doi: 10.1002/sim.1186
- Conway KJ, Orr R, Stannard SR. Effect of a divided caffeine dose on endurance cycling performance, postexercise urinary caffeine concentration, and plasma paraxanthine. J Appl Physiol (1985). 2003 Apr;94(4):1557–1562. doi: 10.1152/japplphysiol.00911.2002
- Kilding AE, Overton C, Gleave J. Effects of caffeine, sodium bicarbonate, and their combined ingestion on high-intensity cycling performance. Int J Sport Nutr Exercise Metab. 2012 Jun;22(3):175–183. doi: 10.1123/ijsnem.22.3.175
- Hodgson AB, Randell RK, Jeukendrup AE. The metabolic and performance effects of caffeine compared to coffee during endurance exercise. PLoS One. 2013;8(4):e59561. doi: 10.1371/journal.pone.0059561
- Santos Rde A, Kiss MA, Silva-Cavalcante MD, et al. Caffeine alters anaerobic distribution and pacing during a 4000-m cycling time trial. PLoS One. 2013;8(9):e75399. doi: 10.1371/journal.pone.0075399
- Skinner TL, Jenkins DG, Taaffe DR, et al. Coinciding exercise with peak serum caffeine does not improve cycling performance. J Sci Med Sport. 2013 Jan;16(1):54–59. doi: 10.1016/j.jsams.2012.04.004
- Quinlivan A, Irwin C, Grant GD, et al. The effects of red bull energy drink compared with caffeine on cyclinG time-trial performance. Int J Sports Physiol Perform. 2015 Oct;10(7):897–901. doi: 10.1123/ijspp.2014-0481
- Goncalves LD, Painelli VD, Yamaguchi G, et al. Dispelling the myth that habitual caffeine consumption influences the performance response to acute caffeine supplementation. J Appl Physiol. 2017 Jul;123(1):213–220. doi: 10.1152/japplphysiol.00260.2017
- Felippe LC, Ferreira GA, Learsi SK, et al. Caffeine increases both total work performed above critical power and peripheral fatigue during a 4-km cycling time trial. J Appl Physiol (1985). 2018 Jun 1;124(6):1491–1501. doi: 10.1152/japplphysiol.00930.2017
- Larson EM, Oliver JT, Stickford JL, et al. Exercise performance and perception of breathlessness after caffeine ingestion in trained cyclists: 1951 board #212 May 31 3. Med Sci Sports Exerc. 2018;50(5S):471. doi: 10.1249/01.mss.0000536630.97197.fb
- Santos PS, Felippe LC, Ferreira GA, et al. Caffeine increases peripheral fatigue in low- but not in high-performing cyclists. Appl Physiol Nutr Metab. 2020 Nov;45(11):1208–1215. doi: 10.1139/apnm-2019-0992
- Roelands B, Buyse L, Pauwels F, et al. No effect of caffeine on exercise performance in high ambient temperature. Eur J Appl Physiol. 2011 Dec;111(12):3089–3095. doi: 10.1007/s00421-011-1945-9
- Mesquita RNO, Cronin NJ, Kyrolainen H, et al. Effects of caffeine on neuromuscular function in a non-fatigued state and during fatiguing exercise. Exp Physiol. 2020 Apr;105(4):690–706. doi: 10.1113/EP088265
- Meeusen R, Roelands B, Spriet LL. Caffeine, exercise and the brain. Nestle Nutr Inst Workshop Ser. 2013;76:1–12.
- Davis JM, Zhao Z, Stock HS, et al. Central nervous system effects of caffeine and adenosine on fatigue. Am J Physiol Regul Integr Comp Physiol. 2003 Feb;284(2):R399–404. doi: 10.1152/ajpregu.00386.2002
- Fredholm BB, Bättig K, Holmén J, et al. Actions of caffeine in the brain with special reference to factors that contribute to its widespread use. Pharmacol Rev. 1999;51(1):83.
- Kalmar JM. The influence of caffeine on voluntary muscle activation. Med Sci Sports Exerc. 2005 Dec;37(12):2113–2119. doi: 10.1249/01.mss.0000178219.18086.9e
- Mizuno M, Kimura Y, Tokizawa K, et al. Greater adenosine A(2A) receptor densities in cardiac and skeletal muscle in endurance-trained men: a [11C]TMSX PET study. Nucl Med Biol. 2005 Nov;32(8):831–836. doi: 10.1016/j.nucmedbio.2005.07.003
- Dodd SL, Brooks E, Powers SK, et al. The effects of caffeine on graded exercise performance in caffeine naive versus habituated subjects. Eur J Appl Physiol Occup Physiol. 1991;62(6):424–429. doi: 10.1007/BF00626615
- Fredholm BB. Adenosine actions and adenosine receptors after 1 week treatment with caffeine. Acta Physiol Scand. 1982 Jun;115(2):283–286. doi: 10.1111/j.1748-1716.1982.tb07078.x
- Nikodijević O, Jacobson KA, Daly JW. Locomotor activity in mice during chronic treatment with caffeine and withdrawal. Pharmacol Biochem Behav. 1993 Jan;44(1):199–216. doi: 10.1016/0091-3057(93)90299-9
- Lara B, Ruiz-Moreno C, Salinero JJ, et al. Time course of tolerance to the performance benefits of caffeine. PLoS One. 2019;14(1):e0210275. doi: 10.1371/journal.pone.0210275
- Beaumont R, Cordery P, Funnell M, et al. Chronic ingestion of a low dose of caffeine induces tolerance to the performance benefits of caffeine. J Sports Sci. 2017;35(19):1920–1927. doi: 10.1080/02640414.2016.1241421
- Hopkins WG. How to interpret changes in an athletic performance test. Sports Sci. 2004 Jan 1;8:1–7.
- Pickering C, Grgic J. Caffeine and exercise: what next? Sports Med. 2019 Jul;49(7):1007–1030. doi: 10.1007/s40279-019-01101-0
- Yang A, Palmer AA, Wit HD. Genetics of caffeine consumption and responses to caffeine. Psychopharmacology. 2010;211(3):245–257. doi: 10.1007/s00213-010-1900-1
- Roza G, Christensen, Magnus M, et al. Comparisons of CYP1A2 genetic polymorphisms, enzyme activity and the genotype-phenotype relationship in Swedes and Koreans. Eur J Clin Pharmacol. 2007;63(6):537–546. doi: 10.1007/s00228-007-0288-2
- Sachse C, Brockmöller J, Bauer S, et al. Functional significance of a C→A polymorphism in intron 1 of the cytochrome P450 CYP1A2 gene tested with caffeine. Brit J Clinical Pharma. 47, 445-449. British Journal of Clinical Pharmacology. 1999;47(4):445–449. doi: 10.1046/j.1365-2125.1999.00898.x
- Butler MA, Iwasaki M, Guengerich FP, et al. Human cytochrome P-450PA (P-450IA2), the phenacetin O-deethylase, is primarily responsible for the hepatic 3-demethylation of caffeine and N-oxidation of carcinogenic arylamines. Proc Natl Acad Sci USA. 1989 Oct;86(20):7696–7700. doi: 10.1073/pnas.86.20.7696
- Magkos F, Kavouras SA. Caffeine use in sports, pharmacokinetics in man, and cellular mechanisms of action. Crit Rev Food Sci Nutr. 2005;45(7–8):535–562. doi: 10.1080/1040-830491379245
- Abernethy DR, Todd EL. Impairment of caffeine clearance by chronic use of low-dose oestrogen-containing oral contraceptives. Eur J Clin Pharmacol. 1985;28(4):425–428. doi: 10.1007/BF00544361
- Perera V, Gross AS, McLachlan AJ. Influence of environmental and genetic factors on CYP1A2 activity in individuals of South Asian and European ancestry. Clin Pharmacol Ther. 2012 Oct;92(4):511–519. doi: 10.1038/clpt.2012.139
- Maughan RJ, Griffin J. Caffeine ingestion and fluid balance: a review. J Hum Nutr Diet. 2003;16(6):411–420. doi: 10.1046/j.1365-277X.2003.00477.x
- Greer F. Myth buster: caffeine does not exhibit a diuretic effect during exercise performance. Indian J Med Res. 2010 Jul;132:11–13.