ABSTRACT
The early twentieth-century industrialization of food production and processing generated large volumes of processing waste. Following the fate of waste products cast off from the new food commodities, this article describes the logics and practices of large-scale waste reuse as animal feed and microbial nutrient medium in industrial chemical production in the United States. A “chemical gaze” on matter recast disparate burdensome byproducts such as beet pulp, cottonseed meal, or arsenic trioxide in terms of resources to be fed forward through microbial and animal metabolism. Focused on feed efficiency and growth promotion, this chemical gaze was selectively attuned to growth yet blind to the emerging infrastructure of waste-as-feed as a massive circulatory system for both nutrients and the toxicants introduced by growth promoters. Following metabolic pathways of materials over time, this history is at once an account of animal feed systems in twentieth-century America, and a tracing of the externalities of food commodities as they pass through different chemical forms.
The turn of the twentieth century was a time of rapid innovation in food commodities. Foodstuffs became more standardized, and innovations in canning, drying, packaging, pasteurizing, refrigeration, and refining meant that they traveled further, lasted longer, and reached more consumers regardless of season or origin (Levenstein Citation2003). Processes of grinding, fermenting, butchering, flavoring, preserving or churning were relocated from the home kitchen (or the gut) to the manufacturers and distributors of food and drink commodities, while new foodstuffs that had not before traveled long distances came into more diets than ever before (Bauch Citation2011). Histories of fresh milk, sugar, tea, canning, cereal, meat, soft drinks and many more speak to this profound transformation of food preparation, commerce, and consumption.
While the single commodity has provided many useful and narratively tractable routes for tracing the transformation of food over time, this article looks to waste and discard studies, departing from any one foodstuff to examine what was left behind in production as the scale of manufacturing grew precipitously: distiller’s waste, mash left after plant oil is extracted, husks and cobs and pulp and bones and sawdust and fish heads and rinds and bran, or, somewhat less obviously related to food, arsenic waste generated in copper smelting, and pharmaceutical waste (Zimring and Rathje Citation2012). I do not follow the purified inside of the industrial food product (canned peaches, pork chops, white sugar, corn flakes) but rather focus on interlinked cycles of use and reuse changed by the residues of their purification. In particular, I recount the remaking of nutritive and toxic relations between plants, animals, microbes, minerals and humans occasioned by the feeding forward of manufacturing wastes into animal husbandry, and the feeding forward of food or agricultural waste into chemical manufacturing.
The story of how food animals were chemically remade by waste-as-feed is both a specific story of the transformation of meat, milk and egg production, and a general demonstration of the value of including waste processes in the empirical work of food studies, particularly when analyzing large-scale production. Wastes are by their nature often left out of the picture, indeed a great deal of technological work is put into making them disappear. Yet the analysis below demonstrates that the heterogeneous trajectories of waste make the homogenous food commodity possible, both materially and economically. I show that a distinctive approach to manufacturing and agricultural waste emerged in early twentieth century America, one that I characterize as a “chemical gaze.”Footnote1 Where the annoyed passerby might see a riot of smelly detritus – beet pulp, fish heads, corncobs – the chemical gaze perceived carbohydrate and nitrogen. Where the naturalist might see species and kingdoms – plants, microbes, animals – the chemical gaze traced a metabolic map of enzymatic and energetic conversions between different kinds of matter connecting one body to another across taxonomic boundaries.
The lineaments of the chemical gaze were simultaneously economic and scientific, native to the practical character of agricultural and industrial chemistry and microbiology. Here I follow the lead of historian Emma Spary, who argues in Feeding France that between 1760 and 1815 it was an industrial chemistry, “perhaps more than any other single science, which most transformed daily life and material culture in the 19th century” (Citation2014). In twentieth-century America, there is an important but only partially mapped set of relations between industrial chemistry, agriculture and eating that not only transformed daily life and material culture, but also ecologies, bodies, and the material interdependencies between different kinds of organisms. Modern chemical production is not just as an origin point for toxins and pollutants (although it is also that) but is a constitutive history of contemporary bodies, “always already present in everything we do, as literally part of us” (Romero et al. Citation2017, 159; Murphy Citation2017) My approach is consonant with recent arguments for “thinking chemically” in cultural geography and anthropology, where the terms “chemo-ethnography” and “chemical geographies” have been mobilized to describe a growing body of literature that pays “sustained attention to the inherently chemical nature of the world and the chemicalized nature of twenty-first century life” (Romero et al. Citation2017, 159; Shapiro and Kirksey Citation2017).
If the first aim of this article is to give a historical account of the chemical gaze in the transformation of food animals, the second is to reckon the consequences of its applications. Dissecting the logic of the chemical gaze shows how nutrition and toxicity came to travel together in animal feeding. The drive to reuse waste as cheap fodder came hand in hand with new adjuvants intended to correct for the nutrient deficiencies, non-palatability, or disease susceptibilities engendered by new feeding regimes. As animal feed was increasingly manufactured rather than locally grown, these adjuvants – growth promoters such as antibiotics or arsenicals – began to circulate through animal and human bodies at scale, and through the soil, water and air. Although biologically potent, the growth promoters and supplements keeping the system going were deployed as if they simply disappeared upon use. Therefore, at stake in the history of animal feed are the environmental and public health consequences of a massive redistribution system of nutrients and toxicants built in the name of feed efficiency.
Of course, a comprehensive history of all manufacturing wastes is well beyond the scope of the present article, and my aim is not waste per se, but the specific system of circuits built to move it through microbial, plant, animal, and human metabolisms. In what follows, I develop a series of examples that illustrate how manufacturing waste from food processing, mining, and pharmaceutical industries came to provide an important input to animal husbandry in the United States in the early twentieth century, which itself generated waste products subsequently cycled back into production. I then assess why these processes were not frictionless, and how the system of circulation became one of both nutrients and toxins. I conclude by discussing historiographical implications of following cycles of the biochemical transformation of by-products instead of genetic lineages or single commodities.
1. From beet pulp to cornstalks: the outsides of commodities
Practices of animal and plant breeding moved into a new alignment with the commodity form in Europe and America beginning in the late nineteenth century. The consistency and stability over time of food or pharmaceutical-producing living things was a central concern for plant and animal scientists as well as microbiologists and vaccine makers (Mendelsohn Citation2016). Crop plants such as beets were bred both for high sugar content and low variability in sugar content (Bonneuil Citation2016). This is the history of the purified inside: what was pushed to the outside? Beets provide a convenient entrée to this question. Even as their industrial usage expanded in the production of sugar and alcohol at the end of the nineteenth century, the processes allowing for the extraction and purification of sugar generated copious beet pulp, which promptly became an urban hazard.
According to a rather obscure but useful little book, The Golden Anniversary of Scientific Feeding, published in 1947, “in the early days of the beet sugar industry, the beet pulp was not dried but was allowed to accumulate near the factories where it quickly became a great mass of decaying vegetable matter that was extremely offensive to the people in the community. The factories tried to get the farmers to use the wet pulp, even offering it free of charge, but the number of farmers within hauling distance … was not sufficient to use the material” (Wherry Citation1947, 37). In 1911–1912, the US production of beet sugar – 543,860 metric tons – presented a considerable challenge in terms of residue generation (Pearl Citation1920, 47). Some producers turned to keeping cattle or sheep to eat the pulp, but this was generally impracticable; beet sugar factories were not farms. No matter how genetically stable and how standardized the sugar content of the beet, processing generated mountains of beet pulp, because one could not get to beet sugar without going through the beet first. The “body” of the beet, that metabolic thing which made sugar but was unwanted after its singular productive function was exhausted, was still there.
Sugar producers were under considerable pressure to dispose of their odiferous byproduct, as urban smells were a source of health concerns (Kiechle Citation2017). Drying technologies imported from Europe helped convert wet pulp into dry, and after an enterprising businessman took interest in a distress-lot of dried beet pulp, it appeared about 1910 in America as “the feeding sensation among dairymen” (Wherry Citation1947, 37). In 1926, approximately 200,000 metric tons of dried beet pulp was being fed to animals, expanding to half a million tons by 1954 (King Citation1958). Thus, dried beet pulp took its place amongst other repurposed processing wastes as a cheap source of animal feed, in a period when farmers were admonished to always calculate “feed efficiency.” This meant using feeds that increased both production of animal goods and profit gained, reducing the cost of feed and labor in relation to sale prices of meat, milk, eggs, or wool.
Such waste economies are an underappreciated element of the late nineteenth century establishment of the corporate form in the United States. The American Cotton Oil Company was founded in 1889 as a New Jersey holding company, and became a legally organized corporation listed on major stock exchanges, one of the first industrial stocks on markets previously dominated by railroad and government finance (Roy Citation1999, 199). Cottonseed oil had a long road to value, beginning as a nuisance waste of cotton ginning. A history of the industry reports that “many an antebellum cotton gin was setup on the bank of a stream so that the seed could be washed away,” spurring states to introduce measures such as Mississippi’s fine for dumping cottonseed “in any stream used for drinking or fishing purposes,” or for allowing it to accumulate within half a mile of a city or town (Nixon Citation1930, 73).
One way to dispose of cottonseed waste was to extract oil from it, initially a product in search of a use. After an idiosyncratic itinerary as an illegal adulterant to hog lard and cheap alternative to animal fats in soap-making in the nineteenth century, it came into its own as a household culinary item with the development of deodorized Wesson cooking oil in 1899 and Proctor & Gamble’s Crisco shortening in 1911 (Pendleton Citation1999). In making oil from cottonseed, cottonseed meal is left as a waste product. The meal was discarded or shipped to Europe to be used as animal fodder. American uptake of German agricultural nutrition science and its recognition of seeds as protein-rich nutritional supplements eventually led to the reframing of cottonseed meal as a valuable national resource (Matz Citation2015; Finlay Citation2015). Oil-stripped mash was high in protein, and thus a good source of nitrogen. Cottonseed meal was thereby repurposed as a protein supplement in animal feed, and as fertilizer. In 1921, 1,044,6000 tons of cottonseed meal went to animal feed, doubling over the next 30 years (King Citation1958).
The National Linseed Oil Company was capitalized at 18 million dollars in 1899.Footnote2 The National Starch Manufacturing Company and the American Sugar Refining Company were the first “food” industry players to adopt corporate form in the late 1890s. The American Distilling & Cattle Feed Company likewise was among the first 12 stocks on the original Dow list in 1896, having entered the cattle feed business by virtue of needing to dispose of whisky mash, and then into the fertilizer business by virtue of needing to dispose of the cow manure, after an order from the Illinois river and harbor commissioner “made it imperative that some means of taking care of the refuse had to be made other than dumping it into the river” (Anonymous Citation1906, 38). Meanwhile, corn gluten, a byproduct of starch and corn syrup manufacture, was “disposed of by pouring it in the Hamburg Canal in Buffalo, much to the disgust of inhabitants past whose back doors the canal flowed” (Wherry Citation1947, 2). In other industries, increasingly popular fresh milk and dairy goods generated buttermilk and milk solid byproducts (Dupuis Citation2002). Canning and meat packing industries generated fish and meat offal, blood, and “tankage” (residue from carcass rendering), all of which demanded disposal in places other than the river or the back lot. “Great competition and the lowering of prices,” wrote an observer in 1919, “have made it essential for factories to utilize or dispose of all of their waste material, and processes that leave large margins for waste have not much chance of success” (Shelford Citation1919, 102).
Thus, according to The Golden Anniversary, “men of vision realized that a new era in poultry and livestock production could be built on the efficient utilization of those precious proteins that were wasted in an earlier day. And so it was that the golden age of better feeding began” (Wherry Citation1947, 1). However, it was not inevitable that the nation’s farmers accepted processing wastes instead of their own fodder crops. A considerable technical infrastructure and the expansion of scientific and accounting advice in American farming underpinned the practice (Fitzgerald Citation2003). A manufactured feed industry emerged between 1890 and 1910 alongside the national mandate that agricultural science foster farm production, supported by a technical literature declaring that scientific feeding was necessary to avoid waste and access profit. Feeding animals more than they needed, insufficient protein, or the wrong feed for growth stage were all forms of loss of profitability (Armsby and Moulton Citation1925). Feed efficiency arose as a central value in science and agriculture concomitant with the spread of efficiency values and practices in management and economics. For example, Harrington Emerson’s 12 Principles of Efficiency overtly compared organization to organisms, in turn casting organisms as organizations to which those principles could be applied (Citation1912).
Perhaps the quirkiest story of efficiency ideals applied to leftovers comes from corn. Harvey Wiley, a chemist and later Chief of the Bureau of Chemistry in the Department of Agriculture, was retained by the Marsden Company of Philadelphia to develop uses for byproducts of the “Marsden process,” in which the pith of cornstalks was ground to fine powder, then mixed with molasses to make bricks. The bricks were built into three-foot thick hull linings for battleships, designed to be self-sealing, as incoming water would make the material swell in the event of being punctured by a shell. Even the processing of corncob pith generated byproducts. “Being of a thrifty nature,” Wiley used these cornstalk remnants as feeds for cattle, but “methods of warfare changed, and the ships abandoned the use of the protective cellulose lining. So the Marsden Company was left only with a by-product process for making molasses feeds” (Wherry Citation1947, 14). In other words, the byproduct of battleship lining became the main business.
This story points to the key role of molasses, a waste product of sugar refining. Molasses made feeds composed of cheap agricultural byproducts more nutritious and more palatable to animals. Sucrene Oil Meal, for example, combined linseed oil meal, “midds” (wheat middlings), and molasses ().
Figure 1. 1918 Advertisement for Sucrene Hog Meal Feed containing molasses and linseed meal, showing macronutrient breakdown and promising more pork per pound, in less time. From The Country Gentleman. Public domain.
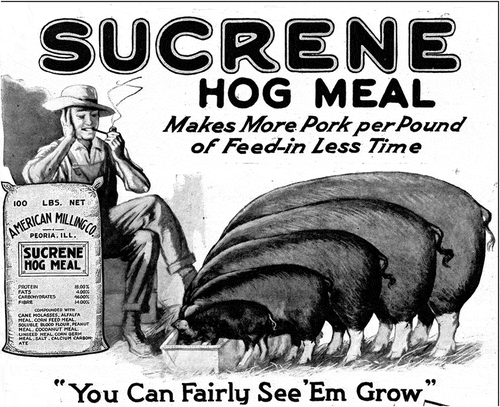
Molasses was difficult to work with and depended on a racist economy of cheap labor. The apparent nutritional benefit of molasses to animals was offset by difficulty in handling, the description of which shows the mid-twentieth century view on the primitivism of the substance: “It was common practice to spread the dry ingredients on the floors of the facility, sprinkle on molasses from a sprinkling can” (Wherry Citation1947, 15). A crew of bare-footed African America workers would then “with shovels, mix it into soft masses the size of croquet balls and dry these in open burlap pans in a hot-air room” (Wherry Citation1947, 15).
Molasses thus began as a nuisance byproduct of less value than the cost of its transport. Yet its manifest usefulness in disposing of other waste by making even sawdust a candidate for animal food by adding nutrients and palatability led to a demand so large that the United States began importing supplies from Cuba in 1914. Discovery of numerous B vitamins between 1930 and 1950 and increasing appreciation of the role of essential trace nutrients such as manganese altered the value of molasses; the process of making refined sugar stripped all these nutrients away, concentrating them in the byproduct.
Smell was one problem; stickiness was another. In feeding wastes onward, one cannot underestimate the importance of the chemistry of drying technologies. Chickens were often fed dairy waste, particularly before vitamin concentrates came available in the 1930s. Milk drying technology made transport and mixing feasible, but created the next problem: chicken manure high in casein, a milk protein excreted undigested by the chickens, which “has the sticky consistency and ammoniacal odor of partially ripened cheese.” As observed in a 1927 patent filed by the American By-Product Company concerning new chemical treatment of fattening-pen manure, “this manure possesses valuable fertilizing properties, but efforts to reduce it to a dry condition suitable for use on a commercial basis and without material loss of fertilizing qualities have failed. When heated it melts into a sticky mass which cannot be dried by ordinary treatments” (Maclachlan Citation1929). This problem could be solved with appropriate chemistry: heating with acid salt granulated the casein. Further heating resulted in a hard cake that could be pulverized. The patent notes that the addition of poultry feathers or whole bird corpses and sodium sulfide further facilitated the drying process and increased the nitrogen content of the fertilizer. In this way chicken manure made it back onto the crop fields, perhaps fostering the growth of beets to make sugar, or cotton to make oil.
2. The chemical gaze
In an inverse way, the leftover bodies – seed hulls and pulps, undigested casein, fish heads – continue to drive forward the commodities plumbed from their centers or essences by ensuring that processing can continue, not buried under its own weight. Yet it seems deceptively straightforward to depict this as simple re-use, as if occurring without work. In the example of protein, a theory of chemical unity and inter-convertibility was needed to open the conduit from cotton to meat; in the example of chicken manure, the chemistry of sticky substances and the engineering of drying machines was necessary to make the substance useable. And, as seen in almost every example proffered above, it took social or economic sanction to divert these matters from the nation’s waterways and drive the capitalization of their repurposing.
The recasting of individual materials in terms of their common chemical constituents or properties rendered the motley collection of byproducts described above legible as an economy of nutrients, energy, and elements. The flow of these elements was brought into the foreground, while the individual body, the species, the particular waste in question, and the specificities of geography and community receded in importance. The statistician Raymond Pearl (better known for later work on longevity) detailed the national economy of protein, carbohydrate, fat, and calories in 1920 in The Nation’s Food: A Statistical Study of a Physiological and Social Problem (Pearl Citation1920). The study, commissioned in the depths of war, sought to answer basic questions of the nation’s food supply and ability to export foods to war-torn Europe. Pearl argued it was necessary to go beyond crop statistics to parse out what proportion of agricultural products were consumed by animals, and what by humans, and to know “in nutritive units” how the balance sheet came out. For example, when counting by “nutritive unit” it was evident that a very few commodities contributed the majority of the nation’s protein, with wheat, hogs and dairy heading the list ().
Figure 2. Thinking in protein and animal units. This USDA graph from 1958 shows the increase in protein feeding and use of oilseed meal over the first half of the twentieth century. Public domain. (King Citation1958).
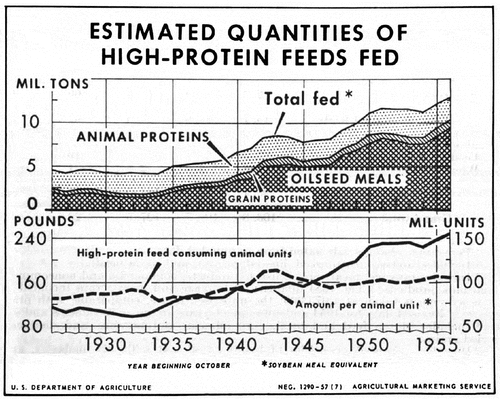
One didn’t want humans and animals competing for the same food, so sources of protein edible to animals but inedible to humans were needed to make the protein economy solvent.
Such calculations characterized the nation’s animals as suffering from “protein hunger.” The feed industry was charged with using materials that would otherwise be wasted, to educate the feeder, and to supply economical feeds to fill the gap:
There always has been “protein hunger” among the common flocks and herds in this country. Our nation can nearly double the use of protein concentrates before the saturation point is reached. It is generally agreed that for efficient production of meat, milk and eggs, high protein concentrates should be fed with carbohydrate feeds in the ratio of about 1 to 6. Yet we have never had enough protein available to feed at a ratio greater than 1 to 10 or 1 to 12 (Wherry Citation1947, 106).
The chemical gaze on matter and its cultivation in the name of productivity was particularly evident in the 1920s American “chemurgy” movement (named by combining “chemi” and “ergon,” Greek for work). Historian Mark Finlay has analyzed this movement’s concern with the oversupply of American farm products in the wake of World War I, when demand for them fell sharply upon recovery of European farming and protectionist tariff policies. William Hale, a leader of the movement, “argued that an emergent chemurgic revolution meant that farmers should discard the cultivation of old-fashioned crops with ‘time-worn nicknames’ like corn and wheat and instead view their job as the cultivation of products with real meaning: cellulose, starch, lignin, levulose, and the like”(Citation2003, 37). Nonfood uses of farm products included the development of a soybean-based plastic trunk lid for Ford cars, and a solvents industry that employed sugar beets, Jerusalem artichokes and surplus grain as raw material for bacterial fermentation (Finlay Citation1990). The imperative to develop a chemical approach to farm waste was pursued in academic settings and in US Department of Agriculture research laboratories (Marcus and Lokensgard Citation1986).
Making building insulation or trunk lids out of wastes were however much less successful than feeding them onward into other metabolisms. As ecologist Victor Shelford put it in an argument for protecting fish stocks from pollution via increased economy in waste, “If one can find no other use for a waste material, he can probably work it off either as a cattle food or as a fertilizer” (Citation1919, 102). Shelford could well have added nutritive medium for microbes to this statement. World War I transformed the perception of microbes as locations of chemical power and creativity. While origins of such a perspective lie in the view of microbes as “living workers” in making fermented food products, disruption of flow of materials between countries in World War I drove increased repurposing of manufacturing waste as nutritive media for industrial fermentation (Lee Citation2015; Fruton Citation2006).
The acetone-butanol process, by which bacteria metabolize starch to produce acetone and butanol, changed the course of World War I (Bud Citation1994). Acetone was previously derived from destructive distillation of wood and calcium acetate, but a blockaded Britain was short of materials. Acetone was used in smokeless powder, ensuring warships did not reveal their locations when firing guns. Distilleries in Toronto and Terre Haute were converted to microbial acetone production. Albert Kluyver, a Dutch microbiologist noted for his theories of the chemical unity of life through investigations of bacterial metabolism commented that,
at the time of armistice, 5,000 tons of acetone and twice that amount of butanol had been produced in the U.S.A. alone, and this owing to the activity of a microbe that twenty years ago had probably never been considered worthy of human attention. In this connexion I may point out that the thousands of cultures employed in these industrial fermentations may well have originated from one bacterial cell whose dimensions … are very small indeed. If we further realize that in a relatively short time that single, minute cell has given rise to the construction of many imposing factories in various parts of the world, then it is unavoidable not to be impressed by the enormous powers that reside even in the smallest living entities (Kluyver Citation1959, 173–74).
Speaking in 1922, Kluyver commented that Bacillus acetoethylicum usefully produced both acetone and butanol, and moreover was “not particular as far as its nutrition is concerned … this opens up a means for economically disposing of corn cobs, now representing a waste product which, in the U.S.A., amounts to 20 million tons annually” (Citation1959, 173). The Terre Haute facility was bought from the Allied War Board by the Commercial Solvents Corporation (CSC), which continued the production of acetone after 1918 and found a use for the butanol byproduct. Butanol became a solvent for quick-drying car lacquers, and in 1923 CSC opened a second plant in Peoria, Illinois. By 1925 they were producing 100 tons of solvents per day, with corn and molasses as a nutrient base (Jones and Woods Citation1986). In the 1950s, competition from petroleum chemistry and changes in the economics of molasses due to increased use in cattle feed led to the decline of this fermentation-based production. Meanwhile, CSC entered the animal nutrition market in the 1930s with the microbial production of vitamins, and then penicillin (Kelly Citation1936). All of these were, in Kluyver’s words, “the macro-achievement of a micro-organism” (Citation1959, 170).
Efficiency as fundamental to national prosperity, and a corollary view of waste and unused resources as political vices inimical to both country and economy were not new ideas. Emma Spary shows how earlier versions of these values were prominent in the work of chemists and agronomists and the relationship between “governmentality and consumption” in France between 1760 and 1815 (Citation2014, 16). Twentieth-century agricultural chemistry and agronomy were certainly shaped by this inheritance, yet by then, the chemical gaze on the circulation of matter was informed by intermediary metabolism and the realization that all cells, including microbes, were chemical consumers and producers. The biochemical unity of metabolism across species was operationalized and scaled up in linking the metabolic abilities of different kinds of organisms into novel chains of production for the economic re-use of farm surpluses and manufacturing waste.
3. Enter growth promoters
There is nothing wrong with re-use. This history of refiguring nutrient flows could be one of laudatory efficiency, perhaps of values and techniques that we might wish to recall in these times of carbon excesses and water shortages. In general, it seems economically and environmentally sound to find productive uses for things that otherwise end up in waterways or landfills. Observing that “waste is an inherently ambiguous linguistic signifier,” Myra Hird writes “anything and everything can become waste, and things can simultaneously be and not be waste, depending on the perceiver” (Citation2012, 454). Certainly, from the point of view outlined above, in which a chemical gaze parsed matter into potential streams through other metabolisms, it would be possible to argue that there was no outside to this system of coping with the externalities of commodities: no waste, just substrate and product moving through the paces of enzymatic inter-conversion.
However, given that one aim of this analysis is to show how waste re-feeding constituted a biochemical transformation of everyday life, re-use is only part of the story. The material flows of industrial metabolism became as much a novel landscape of toxicity and dysbiosis as one of nutrition and recirculation. There are several reasons why this was not a perpetual motion machine of feeding and re-feeding proceeding without residues (Boudia et al. Citation2018). Each re-fed substance enters into other conduits of consumption, production, and excretion. While changes in breeding and transport are important to the intensification of animal agriculture, developments in feeding practices such as protein supplementation, microbial fermentation of vitamin B12, and the development of growth promoters transcended key bottlenecks to the industry’s expansion. Facilitation of the intensification of animal agriculture has resulted in extraordinary amounts of animal waste, with costs displaced on neighboring communities and ecosystems (Blanchette Citation2019).
More specific to the history described above, manufacturing wastes were nutrient poor, nutritionally unbalanced, or unpalatable. Their profitable use was made possible only by a wide array of adjuvants that balanced amino acid ratios, improved feed efficiency parameters, or enhanced appetite. Exactly because chemical adjuvants of waste-as-feed were designed as metabolic supplements, they were effectively distributed through bodies and environments. Whether via intentional mobilization of endocrine disruption through hormone growth promoters, or unintentional distribution of toxic substances accidentally mixed in feed, the animal feed system is a means by which small quantities of biologically potent substances are mixed at trace amounts into large volumes of other materials, and fed through billions of bodies. Today, these former “outsides” of commodities are increasingly visible on the “inside” of things, in human tissues or bacterial genomes or food commodities.
How then does the story of waste re-feeding become a massive circulatory system for trace heavy metals, endocrine disruptors, and antibiotics? A growth promoter is any substance facilitating the growth of plants or animals. Between 1945 and 1955 the term took on new meaning, referring to a novel range of substances able to change the ratio between food input and animal product output, such that less food over less time was necessary to bring an animal to market weight or to laying or milking maturity. The three major classes of growth promoters introduced in this period were arsenical drugs, antibiotics, and hormones, which I will discuss here in turn.
While antibiotics and hormones are better known in terms of their use as animal growth promoters, arsenical medications were introduced earlier. Arsenic was itself a waste product of copper smelting. “Until the early twentieth century, post-smelting arsenic residues and arsenic in smelter smoke were generally regarded as useless or objectionable. Neighbors downwind of copper smelters often complained that stack gas and smoke particulates, settling on farmland, injured crops and grazing livestock” (Tepper and Tepper Citation2013, 65).Footnote3 Yet eventually this nuisance byproduct became a profitable commodity in its own right, first as lead arsenate pesticides used in the American South against boll weevil in the cotton fields and in the Northwest to control apple codling moth. In 1944, arsenical medications were introduced for the control of intestinal parasites in chickens, a growing problem plaguing intensified conditions of year-round indoor poultry raising (Frost Citation1967). Investigators were delighted to find that arsenicals increased weight gain, brought chickens to maturity faster and had the desirable quality of making the meat pinker, all independent of its anti-parasitical activity. These drugs were fed to chickens until 2012 and turkeys until 2015 in the United States (Nachman et al. Citation2017).Footnote4
Antibiotics followed hard on the heels of arsenicals. As described above, the industrial mobilization of microbial metabolism for chemical production reshaped the waste economy in a number of ways. Waste could supply nutrient medium for growing microbes, such as using corncobs for acetone production or corn steep liquor from corn starch manufacturing as a growth medium for Penicillium mold in the making of penicillin during World War II (Finlay Citation1990). Microbially produced vitamins were inexpensive supplements to nutrient-poor fodders. Fermentation, in turn, produced wastes; after a population of microbes had converted its nutritive medium into a desired metabolite such as a vitamin and the product was harvested, a vat of sludge composed of live and dead microbes and their excreta remained. The feeding of vat sludge from antibiotic production to animals initiated the use of low-dose antibiotics as animal growth promoters (Anonymous Citation1952; Finlay Citation2004).
Finally, the story of the first hormonal growth promoter is part of this landscape. Early research on what today is called the microbiome was driven by this waste economy. Wise Burroughs of the Ohio Agricultural Research Station began “digestion experiments” on the ability of cattle to process “poor quality roughages” by inventing an artificial rumen in the late 1940s (Arias et al. Citation1951). He asked if microbes living in the rumen might be harnessed to enable cows to digest poorer quality feeds with the same efficiency as grass or hay. Burroughs concocted feed from ground corn cob, molasses, and urea, a synthetic nitrogen compound made by DuPont, observing how digestion of rough cellulose products was influenced by rumen bacteria (Marcus Citation1993). Such work was later overshadowed by Burroughs’ design of a synthetic estrogen diethylstilbestrol (DES) growth-promoting diet, but the pertinent point is that the discovery of DES happened in the context of aiming to get animals to metabolize cheap agricultural waste (Langston Citation2010).
Arsenicals as growth promoters have increased the arsenic content of foods such as chicken and rice (Silbergeld and Nachman Citation2008). Continued use of antibiotics in animal agriculture means increased flow of antibiotic residues, antibiotic-resistant bacteria, and genetic antibiotic resistance determinants into consumers and the environment on meat, or via water, air and soil (McEachran et al. Citation2015). DES was used in cattle from 1954–1979, during which time approximately 80–95% of feed-lot cattle received the drug, and in broiler chickens from 1947 to 1966 (Langston Citation2010). It was given to pregnant women to prevent miscarriage, its pharmaceutical use discontinued in the United States only in 1971 after the discovery of a cancer cluster in young women exposed in utero. DES changes the epigenome of exposed organisms, particularly during early development, and resulting alterations in gene expression cause multigenerational reproductive abnormalities and cancer risks in descendents of women who took DES (Al Jishi and Sergi Citation2017).
These are examples of intentional distribution, with regulatory approval, of substances for improving the economy of gain in animal husbandry. In many cases, the actual amounts and impacts of these substances remain unknown. For example, what we know about DES derives from tracing the women who took the drug rather than the general population impact of low-dose exposures from food or the environment. There are also unintentional stories, such as the mix-up of Nutrimaster and Firemaster at the Michigan Chemical Corporation in 1973. Nutrimaster was a magnesium oxide supplement for dairy cattle feed; Firemaster was a fire retardant, a polybrominated bisphenol (PBB). Both were white and crumbly and came in brown paper bags. It took eight months to realize that approximately 1000 pounds of Firemaster were accidentally milled into cattle feed in 1973 (Carter Citation1976).
Initially, it was assumed that only people who lived on farms would ingest PBBs by eating meat or milk from affected animals (Reich Citation1983). Yet by 1978, 96% of breast milk samples taken from women in the general population in Michigan’s lower peninsula showed detectable amounts of PBB (Brilliant et al. Citation1978). Scientists had few tools to assess how PBBs in cattle feed became ubiquitous in the region’s inhabitants. PBB, like DES, is an endocrine disruptor, and intergenerational health consequences continue to unfold, such as increased risk of genito-urinary defects and adult infertility in individuals exposed in utero (Small et al. Citation2011).
Commenting on the Michigan disaster in Science, Luther Carter wrote, “Some of these episodes fail to attract national attention … and when the injury is not so much to human health as it is to farm animals the incidents may be little noted outside the regions where they occur” (Citation1976, 240). Indeed, what this story shows is the very un-remarkability of the chemical remaking of animal life through feed. In 1973 (as today), there was nothing abnormal about bags of crumbly white stuff manufactured by a chemical company traveling on a truck to a state-run food mill selling composite feedstuffs in bags to farmers, who assume the bag contains a scientifically verified mixture of nutrients, energy, medicaments, and metabolic adjuvants designed to maximize growth and/or productivity. Manganese oxide is a sweetening agent, which improves the palatability of grass-supplementing composed feed intended to improve milk production. A second reason for its use is the grass itself: fast-growing grass fertilized with high-nitrogen and potassium fertilizer, such as produced from chicken manure, is low in manganese (Grunes and Mayland Citation1976).
4. Conclusion
This article goes in circles. Commodities such as refined sugar spin-off beet pulp repurposed as dairy cow feed. Dairy waste is fed to chickens and chicken waste fertilizes fields, generating crops from which seed mash, pith, and pulp feeds cows and pigs and chickens. Cornstarch liquor nourishes microbes that metabolize vitamins and antibiotics that promote animal growth on such diets. Copper smelting waste makes the meat pinker but flows back into the environment to be sequestered by plant seeds, moving on to other eaters, in and out of bodies of all kinds. Molasses appears at multiple junctures, enhancing palatability and passage. Chicken manure fertilizer changes the nutrient profile of grass, contributing to the need for dietary supplements for dairy cattle.
Both the content and the form of this metabolic history are important to understanding the transformation of industrial animal nutrition in the twentieth century. First, in content, this history has focused on biochemistry and not breeding, in the spirit of what Angela Creager has called a “chemical reaction to the historiography of biology” (Creager Citation2017). Excellent histories of genetics are foundational to our comprehension of the technoscience of foodstuffs, the remaking of pigs, corn, and cows and the erection of property regimes around genotypes (Fitzgerald Citation1990; Bugos and Kevles Citation1992; Allen Citation2014; White Citation2011). And yet the ontologies of inside and outside evinced by historical actors have been overly powerful in setting the parameters of inquiry, unintentionally reifying heredity and genetics as separate from “environment,” keeping our eye trained on descent lines of entities (organisms or commodities made from them) presumed to be internally coherent and self-same over time.
This brings me to form: metabolism as methodological prompt. While maintaining a suitably reflexive awareness of the concept’s European origins in the industrial nineteenth century, and prior modes of its employment in social science, it nonetheless seems that along with a different object of inquiry (biochemical reactions rather than genes), the very nature of the processes under scrutiny distributes historical attention differently (Mackert Citation2016; Wachsmuth Citation2012). Thinking about metabolic relations, their intentional deployment to human economic or engineering ends, and consequences for the chemical landscape in which life proceeds, draws empirical attention toward systems of interlinked processes rather than things (Nicholson and Dupré Citation2018).
Thinking about metabolism diverges from the genealogical bent in scholarship inspired by Foucault aimed at tracing lineages from which things descend and the conditions for the replicative or mutational histories by which they come to pass in their current form (Koopman Citation2013). Metabolic history is not (directly) about the poles of reproduction or death but the axis of eating and growing of somatic life that connects them.Footnote5 In terms of the study of food, this metabolic methodological orientation implies a distinctive perspective, in that the topic under investigation is not limited to humans and what they eat, but captures some small part of a ceaselessly churning scene of incorporation and excretion in which machines, bodies, microbes, enzymes, toxins, and nutrients are moving and converting matter into different forms.
The chemical vision of the twentieth century could look utopic, such as the tree of re-use drawn by ecologist Victor Shelford, in which pollutants were directed away from fish stocks into useful ends ().
Figure 3. Victor Shelford’s tree of re-use showing how industrial wastes could be turned to useful purposes rather than dumped in waterways. (Shelford Citation1919). Public domain.
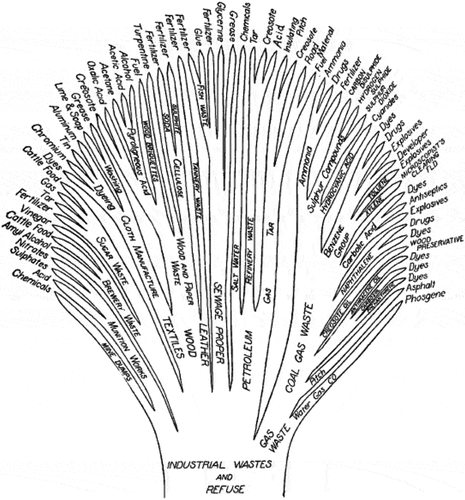
It was a deeply hopeful inventive enterprise, for a prosperous and well-fed nation. Yet what the circularities traced from the stories of the outsides of commodities show us is that not all residues can be continually re-valued, and their continued metabolism is not always possible or desirable. Agents brought into action in the twentieth century to facilitate the re-feeding of manufacturing waste through animal, plant and microbial metabolism, from arsenicals to antibiotics, were treated as though they would simply disappear once they had done their work. Care was not taken with their potency, or their capacity to change and endure.
We are left in the twenty-first century to inhabit the altered metabolisms of the wasteland of the twentieth-century commodity, in which “waste as strategy” continues to render processing profitable (Liboiron Citation2013). It is no longer possible to think that wastes left to the outside of things will stay there or even that there remains a possibility for their exclusion, from food, from the genome, from the gut, from our ova, futures, rivers (Murphy Citation2017). For decades, arsenicals fed to animals were excreted into waste lagoons and spread on fields, where they were taken up into wheat and rice, plants that sequester these elements in their kernels, making their way into the cereals, brown rice syrup, and crackers packaged in boxes festooned with images of rural plenty (Consumer Reports Citation2012). Endocrine disruptors past and present are active participants in the epigenetic topology of gene expression, reshaping physiology and fertility of humans and animals. Antibiotics and heavy metals have irrevocably reshaped bacterial genetics such that antibiotic resistance genes are found not just in pathogens but in the microbes in starter cultures for fermented foods and probiotics (Aarts and Margolles Citation2015).
Not that commodities or animals were ever pure (Latour Citation2012). The historical object in this light loses electrons or amino groups, turns inside out, becomes feedstock for the next move, shows up as a nutrient imbalance in the grass of fertilized fields, inhabits genomes, is a useful accelerant in one cycle and a metabolic disruptor in another. The presence of cycles does not guarantee repetition. It has never been more important to cultivate knowledge of what has fed, and what will feed food.
Acknowledgments
I would like to thank Lieba Faier, Rachel Lee, Sarah Stein, Christine Chism, Barbara Fuchs, Sarah Tracy, and Rachel Vaughn for their input on earlier drafts of this manuscript.
Disclosure statement
No potential conflict of interest was reported by the author.
Additional information
Funding
Notes on contributors
Hannah Landecker
Hannah Landecker is a historian and sociologist of the modern life sciences. She holds a joint appointment across the Divisions of Life Science and Social Science at UCLA, where she is a Professor in the Sociology Department and the Director of the Institute for Society and Genetics.
Notes
1. “Chemical gaze” is used here in intentional paraphrase of Foucault’s concept of the clinical gaze, which he characterizes as a new perceptual regime and structure of knowledge that organized how the doctor saw patients, bodies, and diseases in nineteenth-century medicine. With transformations in the discourse of medicine in the late eighteenth century, the clinical gaze was organized around the lesion or diseased organ, and the systematic imputation of the underlying cause. However, the concept of the gaze itself drew heavily on the figure of nineteenth-century analytic techniques of combustion chemistry, in which complex substances were incinerated and the products separately examined, thereby split into their elements: “the clinician’s gaze becomes the functional equivalent of fire in chemical combustion; it is through it that the essential purity of phenomena can emerge; it is the separating agent of truths … the clinical gaze is a gaze that burns things to their furthest truth” (Foucault Citation2002, 120). Therefore, it seems apt to use the concept to describe how observers trained to see proteins and fats rather than the diversity of foodstuffs, or microbial enzymatic processes rather than mold or putrefaction would have a different view on the world – and put substances to other uses – than other observers.
2. Linseed oil was used in paints, varnishes, enamels, oilcloth, printing inks, shoe polish, patent leather, and as a starting material for further chemical splitting to make glycerine and fatty acids. Its use as a foodstuff was comparatively minor in the early twentieth century. European farmers turned to linseed mash as high protein animal feed earlier than American farmers and it was not until World War I depressed the European market for linseed mash that it saw uptake as animal feed in the US (Eastman Citation1968).
3. The capture of arsenic from smelting smoke became a technological feature of smelters built in the late nineteenth century, such as the ASARCO smelter in Tacoma, Washington. Constructed after the Northern Pacific Railroad completed its route to Tacoma, ASARCO was the destination for mining ores from Washington State and British Columbia. After 1905 it was turned almost exclusively to copper by the Guggenheim brothers, known at the time as the “Kings of Copper” (Tepper and Tepper Citation2013, 67).
4. The history of arsenicals as growth promoters is treated in greater detail in “Trace Amounts at Industrial Scale: Arsenicals, Medicated Feed, and the ‘Western Diet’” (Landecker Citationforthcoming).
5. I owe this formulation to Alex Blanchette.
References
- Aarts, H., and A. Margolles. 2015. “Antibiotic Resistance Genes in Food and Gut (non-pathogenic) Bacteria. Bad Genes in Good Bugs.” Frontiers in Microbiology 5. doi:10.3389/fmicb.2014.00754.
- Al Jishi, T., and C. Sergi. 2017. “Current Perspective of Diethylstilbestrol (DES) Exposure in Mothers and Offspring.” Reproductive Toxicology 71 (August): 71–77. doi:10.1016/j.reprotox.2017.04.009.
- Allen, G. 2014. “Origins of the Classical Gene Concept, 1900–1950: Genetics, Mechanistic, Philosophy, and the Capitalization of Agriculture.” Perspectives in Biology and Medicine 57 (1): 8–39. doi:10.1353/pbm.2014.0003.
- Anonymous. 1906. “Distillery Refuse.” The American Fertilizer 25 (1): 38.
- Anonymous. 1952. “Antibiotics in the Barnyard.” Fortune, March.
- Arias, C., W. Burroughs, P. Gerlaugh, and R. M. Bethke. 1951. “The Influence of Different Amounts and Sources of Energy upon in Vitro Urea Utilization by Rumen Microorganisms.” Journal of Animal Science 10 (3): 683–692. doi:10.2527/jas1951.103683x.
- Armsby, H., and C. Moulton. 1925. The Animal as A Converter of Matter and Energy: A Study of the Role of Live Stock in Food Production. New York: Book Department, The Chemical Catalog Company, Incorporated.
- Bauch, N. 2011. “The Extensible Digestive System: Biotechnology at the Battle Creek Sanitarium, 1890–1900.” Cultural Geographies 18 (2): 209–229. doi:10.1177/1474474010393646.
- Blanchette, A. 2019. “Living Waste and the Labor of Toxic Health on American Factory Farms.” Medical Anthropology Quarterly 1–21. doi:10.1111/maq.12491.
- Bonneuil, C. 2016. “Pure Lines as Industrial Simulacra: A Cultural History of Genetics from Darwin to Johannsen.” In Heredity Explored: Between Public Domain and Experimental Science, 1850–1930, edited by S. Müller-Wille and C. Brandt, 213–242. Cambridge, Mass: MIT Press.
- Boudia, S., A. Creager, S. Frickel, E. Henry, N. Jas, C. Reinhardt, and J. A. Roberts. 2018. “Residues: Rethinking Chemical Environments.” Engaging Science, Technology, and Society 4: 165–178. doi:10.17351/ests2018.245.
- Brilliant, L., G. Van Amburg, J. Isbister, H. Humphrey, K. Wilcox, J. Eyster, A. Bloomer, and H. Price. 1978. “Breast-Milk Monitoring to Measure Michigan’s Contamination with Polybrominated Biphenyls.” The Lancet 312 (8091): 643–646. doi:10.1016/S0140-6736(78)92758-7.
- Bud, R. 1994. The Uses of Life: A History of Biotechnology. Cambridge: Cambridge University Press.
- Bugos, G., and D. Kevles. 1992. “Plants as Intellectual Property: American Practice, Law, and Policy in World Context.” Osiris 7 (1): 74–104. doi:10.1086/368706.
- Carter, L. 1976. “Michigan’s PBB Incident: Chemical Mix-Up Leads to Disaster.” Science 192 (4236): 240–243. doi:10.1126/science.192.4236.240.
- Consumer Reports. 2012. “Arsenic In Your Food Investigated - Consumer Reports.” https://www.consumerreports.org/cro/magazine/2012/11/arsenic-in-your-food/index.htm
- Creager, A. 2017. “A Chemical Reaction to the Historiography of Biology.” Ambix 64 (4): 343–359. doi:10.1080/00026980.2017.1412136.
- Dupuis, E. M. 2002. Nature’s Perfect Food: How Milk Became America’s Drink. New York: NYU Press.
- Eastman, W. 1968. The History of the Linseed Oil Industry in the United States. Minneapolis: T. S. Denison.
- Emerson, H. 1912. The Twelve Principles of Efficiency. New York: Engineering Magazine.
- Finlay, M. 1990. “The Industrial Utilization of Farm Products and By-Products: The USDA Regional Research Laboratories.” Agricultural History 64 (2): 41–52.
- Finlay, M. 2003. “Old Efforts at New Uses: A Brief History of Chemurgy and the American Search for Biobased Materials.” Journal of Industrial Ecology 7 (3‐4): 33–46. doi:10.1162/108819803323059389.
- Finlay, M. 2004. “Hogs, Antibiotics, and the Industrial Environments of Postwar Agriculture.” In Industrializing Organisms: Introducing Evolutionary History, edited by S. Schrepfer and P. Scranton, 237–260. New York: Routledge.
- Finlay, M. 2015. “Science, Promotion, and Scandal: Soil Bacteriology, Legume Inoculation, and the American Campaign for Soil Improvement in the Progressive Era.” In New Perspectives on the History of Life Sciences and Agriculture, edited by D. Phillips and S. Kingsland, 205–229. Dordrecht: Springer.
- Fitzgerald, D. 1990. The Business of Breeding: Hybrid Corn in Illinois, 1890–1940. Ithaca: Cornell University Press.
- Fitzgerald, D. 2003. Every Farm A Factory: The Industrial Ideal in American Agriculture. New Haven: Yale University Press.
- Foucault, M. 2002. The Birth of the Clinic. Translated by R.D. Laing. New York: Routledge.
- Frost, D. 1967. “Arsenicals in Biology: Retrospect and Prospect.” Federal Proceedings (United States) 26: 1. https://www.osti.gov/biblio/6409871
- Fruton, J. S. 2006. Fermentation: Vital Or Chemical Process? Leiden: Brill.
- Grunes, D. L., and H. F. Mayland. 1976. “Grass Tetany … How to Recognize It, Prevent It, and Treat It.” Rangeman’s Journal 3 (6): 180–181.
- Hird, M. 2012. “Knowing Waste: Towards an Inhuman Epistemology.” Social Epistemology 26 (3–4): 453–469. doi:10.1080/02691728.2012.727195.
- Jones, D. T., and D. R. Woods. 1986. “Acetone-Butanol Fermentation Revisited.” Microbiological Reviews 50 (4): 484–524.
- Kelly, F. 1936. One Thing Leads to Another: The Growth of an Industry. New York: Houghton Mifflin.
- Kiechle, M. 2017. Smell Detectives: An Olfactory History of Nineteenth-Century Urban America. Seattle: University of Washington Press.
- King, G. 1958. “The Demand and Price Structure for Byproduct Feeds.” Technical Bulletin 1183. Washington, DC: United States Department of Agriculture.
- Kluyver, A. 1959. “Microbes and Industry.” In Albert Jan Kluyver: His Life and Work, edited by A. F. Kamp, J. W. M. La Rivière, and W. Verhoeven, 165–185. New York: Interscience Publishers.
- Koopman, C. 2013. Genealogy as Critique: Foucault and the Problems of Modernity. Bloomington: Indiana University Press.
- Landecker, H. forthcoming. “Trace Amounts at Industrial Scale: Arsenicals, Medicated Feed, and the ‘western Diet.’.” In Risk at the Table, edited by A. Creager and J.-P. Gaudillière.
- Langston, N. 2010. Toxic Bodies: Hormone Disruptors and the Legacy of DES. New Haven: Yale University Press.
- Latour, B. 2012. We Have Never Been Modern. Cambridge, Mass: Harvard University Press.
- Lee, V. 2015. “Mold Cultures: Traditional Industry and Microbial Studies in Early Twentieth-Century Japan.” In New Perspectives on the History of Life Sciences and Agriculture, edited by D. Phillips and S. Kingsland, 231–252. Archimedes. Cham: Springer International Publishing. doi:10.1007/978-3-319-12185-7_12.
- Levenstein, H. 2003. Revolution at the Table: The Transformation of the American Diet. Berkeley: University of California Press.
- Liboiron, M. 2013. “Modern Waste as Strategy.” Lo Squaderno: Explorations in Space and Society 29: 9–12.
- Mackert, N. 2016. “Feeding Productive Bodies: Calories, Nutritional Values, and Ability in the Progressive-Era US.” In Histories of Productivity: Genealogical Perspectives on the Body and Modern Economy, edited by P.-P. Bänziger and M. Suter, 125–143, New York: Routledge.
- Maclachlan, A. 1929. “Process for Producing Fertilizer and the Resulting Product.” United States US1718297A, filed 1927 1927, and issued 1929. https://patents.google.com/patent/US1718297/en
- Marcus, A. 1993. “The Newest Knowledge of Nutrition: Wise Burroughs, DES, and Modern Meat.” Agricultural History 67 (3): 66–85.
- Marcus, A., and E. Lokensgard. 1986. “The Chemical Engineers of Iowa State College.” The Annals of Iowa 48 (3): 177–205. doi:10.17077/0003-4827.9151.
- Matz, B. 2015. “Nutrition Science and the Practice of Animal Feeding in Germany, 1850–1880.” In New Perspectives on the History of Life Sciences and Agriculture, edited by D. Phillips and S. Kingsland, Vol. 40, 163–181. Archimedes: New Studies in the History and Philosophy of Science and Technology. Dordrecht: Springer.
- McEachran, A., J. Brett Blackwell, D. Hanson, K. Wooten, G. Mayer, S. Cox, and P. Smith. 2015. “Antibiotics, Bacteria, and Antibiotic Resistance Genes: Aerial Transport from Cattle Feed Yards via Particulate Matter.” Environmental Health Perspectives 123 (4): 337–343. doi:10.1289/ehp.1408555.
- Mendelsohn, A. 2016. “Message in a Bottle: Vaccines and the Nature of Heredity after 1880.” In Heredity Explored: Between Public Domain and Experimental Science, 1850–1930, edited by C. Brandt and S. Müller-Wille, 243–264. Cambridge, Mass: MIT Press.
- Murphy, M. 2017. “Alterlife and Decolonial Chemical Relations.” Cultural Anthropology 32 (4): 494–503. doi:10.14506/ca32.4.02.
- Nachman, K., G. Ginsberg, M. Miller, C. Murray, A. Nigra, and C. Pendergrast. 2017. “Mitigating Dietary Arsenic Exposure: Current Status in the United States and Recommendations for an Improved Path Forward.” Science of the Total Environment 581–582 (March): 221–236. doi:10.1016/j.scitotenv.2016.12.112.
- Nicholson, D., and J. Dupré, eds. 2018. Everything Flows: Towards a Processual Philosophy of Biology. Oxford: Oxford University Press.
- Nixon, H. C. 1930. “The Rise of the American Cottonseed Oil Industry.” Journal of Political Economy 38 (1): 73–85. doi:10.1086/254082.
- Pearl, R. 1920. The Nation’s Food: A Statistical Study of A Physiological and Social Problem. Philadelphia: W. B. Saunders.
- Pendleton, L. 1999. “Man’s Most Important Food Is Fat: The Use of Persuasive Techniques in Proctor & Gamble’s Public Relations Campaign to Introduce Crisco, 1911–1913.” Public Relations Quarterly 44 (1): 6–14.
- Reich, M. 1983. “Environmental Politics and Science: The Case of PBB Contamination in Michigan.” American Journal of Public Health 73 (3): 302–313. doi:10.2105/AJPH.73.3.302.
- Romero, A., J. Guthman, R. Galt, M. Huber, B. Mansfield, and S. Sawyer. 2017. “Chemical Geographies.” GeoHumanities 3 (1): 158–177. doi:10.1080/2373566X.2017.1298972.
- Roy, W. 1999. Socializing Capital: The Rise of the Large Industrial Corporation in America. Princeton, N.J: Princeton University Press.
- Shapiro, N., and E. Kirksey. 2017. “Chemo-Ethnography: An Introduction.” Cultural Anthropology 32 (4): 481–493. doi:10.14506/ca32.4.01.
- Shelford, V. 1919. “Fortunes in Wastes and Fortunes in Fish.” The Scientific Monthly 9 (2): 97–124.
- Silbergeld, E., and K. Nachman. 2008. “The Environmental and Public Health Risks Associated with Arsenical Use in Animal Feeds.” Annals of the New York Academy of Sciences 1140 (1): 346–357. doi:10.1196/annals.1454.049.
- Small, C., D. Murray, M. Terrell, and M. Marcus. 2011. “Reproductive Outcomes among Women Exposed to a Brominated Flame Retardant in Utero.” Archives of Environmental & Occupational Health 66 (4): 201–208. doi:10.1080/19338244.2010.539640.
- Spary, E. 2014. Feeding France: New Sciences of Food, 1760–1815. Cambridge: Cambridge University Press.
- Tepper, L., and J. Tepper. 2013. “The Rise and Fall of the Tacoma Arsenic Industry.” IA. The Journal of the Society for Industrial Archeology 39 (1/2): 65–78.
- Wachsmuth, D. 2012. “Three Ecologies: Urban Metabolism and the Society-Nature Opposition.” The Sociological Quarterly 53 (4): 506–523. doi:10.1111/j.1533-8525.2012.01247.x.
- Wherry, L. 1947. The Golden Anniversary of Scientific Feeding. Chicago: Business Press.
- White, S. 2011. “From Globalized Pig Breeds to Capitalist Pigs: A Study in Animal Cultures and Evolutionary History.” Environmental History 16 (1): 94–120. doi:10.1093/envhis/emq143.
- Zimring, C., and W. Rathje, eds. 2012. Encyclopedia of Consumption and Waste. Thousand Oaks, CA: Sage Publications. https://us.sagepub.com/en-us/nam/encyclopedia-of-consumption-and-waste/book235186