Abstract
Supplemental irrigation can be a viable means of increasing lowbush blueberry (Vaccinium angustifolium) crop yields in humid environments. To best utilize this management technique, Maine growers require accurate knowledge of regional crop water demands. Meteorological data and weighing lysimeters were used to determine Penman–Montieth grass reference evapotranspiration (ETo) rates and actual evapotranspiration rates of the lowbush blueberry at five irrigated and two nonirrigated fields of varying distance from the Maine coast. Mean daily values of air temperature, relative humidity range, and solar flux were all found to increase with increasing distance from the coast. The frequency of growing season fog hours increased from 3.7% of all hours at a distance of 22.5 km from the coast, to 11.1% of all hours at a distance of 0.8 km from the coast. Water equivalent inputs from fog per growing season at these two sites averaged 7.2 cm and 18.3 cm, respectively. These geographic climatic differences were reflected in the calculated evapotranspirationo rates, which also generally increased with distance from the coast. Four-year mean weekly ETo rates for July, the month of greatest potential water demand, were 2.76 cm/week, 3.21 cm/week, 3.52 cm/week, and 4.23 cm/week for sites located 0.8 km, 6.4 km, 22.5 km, and 48 km, respectively, from the coast. Actual ET rates for both crop and prune rotations followed a similar pattern of increasing magnitude with distance from the coast. For July, mean crop and prune year pooled weekly ET rates at 0.8 km, 6.4 km, and 22.5 km from the coast were: 1.69 cm/week (0.85), 2.28 cm/week (0.73), and 2.40 cm/week (0.83), respectively. Standard condition crop coefficient (Kc) values for both rotations during the months of May through September were calculated to be 0.68 for sites 6.4 and 22.5 km from the coast. Thus, it would appear that the same Kc value can be used universally within the typical growing range of the Maine lowbush blueberry. These findings should substantially contribute to improved water use efficiency for the irrigated lowbush blueberry in Maine.
INTRODUCTION
Recent research has indicated that the consumption of lowbush blueberries may provide more protection against cancer, heart disease, and the debilitating effects of aging than any other single fruit (CitationJoseph et al., 2003; CitationKalt et al., 2008; CitationSchmidt et al., 2004). Lowbush blueberry production is an integral part of Maine's agricultural heritage and is an important contributor to the state's economy, generating in excess of 80 million dollars in direct revenue in 2007 alone (CitationUniversity of Maine, 2009). As with most agricultural industries, profitability in the blueberry industry is inexorably linked with innovative crop management techniques that best utilize limited resources in a sustainable manner. Expanded irrigation implementation and enhancements in irrigation practices represent important opportunities for improving the profitability of Maine's lowbush blueberry industry. It has been estimated that as a whole, Maine agriculture sustained approximately 32 million dollars in losses during the period of 2001–2002 due to insufficient irrigation (CitationHandley and Hutton, 2007). Yet, the question of whether or not to irrigate in a state such as Maine is a complex one. In arid regions, where rainfall is typically insufficient to meet crop water demands, irrigation is obligatory. However, in humid regions such as Maine, the need for irrigation during a given growing season depends primarily upon the frequency and quantity of rainfall during critical periods of the season (CitationDalton et al., 2004). The feasibility of such supplemental irrigation, which is useful during certain dry periods but often inessential for farm profitability, hinges upon both economic and environmental considerations specific to individual growers. However, before accurate analyses of such factors are possible, the water requirements of the lowbush blueberry must be well understood.
Efficient irrigation management begins with knowledge of the amount of water used by lowbush blueberries during the process of evapotranspiration (ET). In a previous study, CitationHunt et al. (2008) used weighing lysimeters and meteorological data collected over 3 years to determine both actual ET and reference evapotranspiration (ETo) rates for the lowbush blueberry at two sites located within 8 km of the coast in Washington County, Maine. Using pooled data from the sites, they found mean weekly ET rates (with standard deviations) for the months of June through August to be 2.13 (±0.71), 2.39 (±1.05), and 2.19 (±0.97) cm/week, respectively. These rates were close to, but slightly less than, the 2.54 cm/week rate generally considered by growers as the minimum rainfall necessary for optimum crop growth (CitationStarr et al., 2004). Still, the large standard deviations associated with each mean reveal the inherent differences in day-to-day ET demand, indicating that growers need a method for monitoring crop ET in real-time. One method for calculating crop water demand involves multiplication of crop coefficients (Kc) by ETo values determined from coincident, locally collected weather data. Thus, CitationHunt et al. (2008) determined a May through September standard condition crop coefficient (Kc) value for the lowbush blueberry of ∼0.69. One limitation of these results, however, was that these data were collected from a limited geographic area and, thus, may not be indicative of conditions at other locations within the state. Although the lowbush blueberry in Maine is grown primarily within 50 km from the coast, important climatic differences exist within this region that may impact plant water requirements. The objective of this study was to provide Maine growers with information on the water requirements of the lowbush blueberry throughout its commonly occurring growth range, with the overall goal of improving water use efficiency for the entire blueberry industry in Maine. Toward this end, we evaluated the effects of coastal proximity on lowbush blueberry ET rate, ETo rate, and Kc value, as described in the following.
MATERIALS AND METHODS
Research Sites
Research on water demand by the lowbush blueberry was conducted at seven study sites () located at five different locations, varying in distance to the Maine coast from 0.8 km (∼0.5 miles) to 25.7 km (∼16 miles). One of the sites, Blueberry Hill Farm located in Jonesboro, ME, is an experimental farm operated by the University of Maine. Management practices at this farm, such as irrigation, pruning, and pesticide application, were consistent throughout the study period. The four sites located in Jonesport, Deblois, Northfield, and Addison were all privately owned and, thus, subject to the management practices of their owners. The Deblois and Jonesboro sites each contained two weighing lysimeter stations, while the Jonesport, Northfield, and Addison sites had one weighing lysimeter station each. Each weighing lysimeter station consisted of four weighing lysimeters installed several meters apart in a rectangular orientation around a central data logger station (CitationStarr and Yarborough, 2006). Sites at Jonesport, Jonesboro, and Deblois also contained weather stations to measure meteorological data parameters such as air temperature, relative humidity, solar radiation flux, wind speed, precipitation, and visibility. These meteorological data were used to calculate daily Penman–Montieth ETo rates (CitationAllen et al., 1998). Tensiometers were used at all stations to monitor soil water matric potential both inside and outside of selected lysimeters. Soil volumetric water content was measured at Jonesboro in 2006 and 2007 using time domain reflectometry (TDR). Data from an additional weather station located at a research farm in Newport, ME, approximately 48 km from the coast, was also examined to provide weather data more characteristic of sites farther from the coast than Deblois. Although the Newport farm did not grow the lowbush blueberry, its distance from the coast is representative of the inland limit for the majority of blueberries grown in Maine.
TABLE 1 Site Characteristics of Seven Maine Lowbush Blueberry Study Sites
The stations at Jonesboro, Deblois, and Northfield were irrigated using raised sprinkler heads atop movable piping. Irrigation at the experimental farm in Jonesboro was applied when the average soil matric potential of the outside tensiometers decreased below –20 kPa, while irrigation at growers' farms in Deblois and Northfield was based upon proprietary scheduling. No irrigation was applied to the Addison or Jonesport stations during the study period.
Soils varied at the five sites, with root zone soil textures ranging from silty clay to loamy sand. The coarsest soil was found in Jonesport, the site closest to the coast (∼0.8 km). Soils there are mapped as an Adams–Croghan complex (sandy, isotic, frigid Typic Haplorthods and sandy, isotic, frigid Aquic Haplorthods), with a lowbush blueberry root zone consisting of loamy sand for the upper 10 cm that is underlain by sand. Soils at the two Jonesboro stations (∼6.4 km from the coast) are mapped as Colton gravelly sandy loams (sandy skeletal, isotic, frigid Typic Haplorthods) with 0% to 3% slopes. These soils are excessively drained and have a depth to the water table of greater than 203 cm (80 in). Soils at the Addison site (∼8 km from the coast) are a complex of Buxton silty clay loam (fine, illitic, frigid Aquic Dystric Eutrudept) and Lamoine silty clay (fine, illitic, nonacid, frigid Aeric Epiaquept) formed from glaciolacustrine and fine glaciomarine deposits. The Addison root zones are less well drained and have lower hydraulic conductivity than those at the other six sites. Soil at the two Deblois sites (∼14 and 15 km from coast) is classified as Marsardis sandy loam (sandy-skeletal, isotic, frigid Typic Haplorthods) and is considered to be somewhat excessively drained with moderately high permeability. Soils at the Northfield site, approximately 25.7 km from the coast, are classified as a complex of Hermon sandy loams (sandy-skeletal, isotic, frigid Typic Haplorthods) and Monadnock fine sandy loams (coarse-loamy over sandy or sandy-skeletal, isotic, frigid Typic Haplorthods). These soils were subject to occasional flooding due to their proximity to a nearby lake. The finer textured soils at Addison and Northfield were chosen to offer a contrast from the coarser soils at Jonesboro, Deblois, and Jonesport. Information on all stations is summarized in .
Weighing Lysimeters
The 28 weighing lysimeters used in this study were constructed as detailed by CitationStarr et al. (2004) and CitationStorlie and Eck (1996). Briefly, the lysimeter design featured a rectangular inner chamber constructed from 1.91-cm (0.75-in) thick treated plywood inside an outer chamber frame constructed from 3.18-cm (1.25-in) thick treated plywood. The inner chamber, containing soil and blueberry plants, rested upon a single ball-bearing that was centered atop a temperature-compensated weighing load cell. Outside surfaces of the inner chamber were treated with fiberglass cloth and resin. The inner chamber of each lysimeter had dimensions of 46 cm × 46 cm (18 in × 18 in) with a surface area of 0.21 m2 (2.25 ft2) and a depth of 44.5 cm (17.5 in), giving a total internal volume of 0.94 m3 (3.28 ft3). Four small, rigid springs were positioned between the inner and outer chambers to prevent contact between the two chambers. A drainage collection system, consisting of perforated piping, was run from the bottom of the inner chamber to a carboy positioned in an access port adjacent to the outer chamber. Drainage was periodically measured to assess and monitor the water-holding capacity of each lysimeter. Lysimeter accuracy was tested periodically during each growing season using calibration weights, and errors in mass measurements were generally less than 2 %.
Data Analysis
The 10-minute changes in weight for each lysimeter were summed into hourly changes. Hourly changes in lysimeter weight at a particular site were then eliminated if the rain gauge at the site indicated any precipitation for that hour, or alternatively, records indicated the application of irrigation for that hour. Elimination of these “rain hours,” rather than entire “rain days,” allowed the use of a much larger data set. In addition, including all days eliminated the bias of using only “dry” days of generally greater solar flux. As in all lysimeter studies, data associated with excessive drainage, site flooding, animal activity, or similar perturbations to the experimental conditions were eliminated from consideration. In total, 3.59% of the 115,228 total hours of lysimeter data from the Jonesboro stations, 4.11% of the 99,882 total hours at the Deblois stations, 7.83% of the 60,844 total hours from the Addison station, 7.85% of the 45,861 total hours from the Jonesport station, and 7.36% of the 15,007 total hours from the Northfield station were discarded based on these quality control criteria. Hourly data not eliminated due to the above considerations were summed and averaged for the 24 hours of each day. If the difference between the sum and the average for any given day exceeded 15%, then the ET rate for that day was eliminated from consideration. This quality control criterion was followed to avoid using data with too many missing hours, which would result in calculation of an inaccurate daily ET rate.
Daily and weekly crop coefficients were calculated by dividing the lysimeter determined ET rate for a particular time period by the climate coupled ETo rate for the same period. As blueberry plants generally covered the entire surface area of the lysimeters during the months of June through September, the land area used to calculate Kc values was 0.21 m2. Student's T-tests and analysis of variance with Tukey's mean separation analysis were utilized to test the statistical significance of weather variables, ET rates, ETo, rates, and Kc values between stations. Pearson correlations were used to examine the relationship between ET and ETo rates within the same station, as well as the relationship between water equivalent inputs and fog incidence.
RESULTS AND DISCUSSION
Climate Differences
Selected 4-year mean growing season climatic characteristics recorded at lowbush blueberry farms in Jonesport, Jonesboro, and Deblois, ME, are given in . In addition, climatic data from a more inland weather station located in Newport, ME, is also provided in to more completely illustrate the effect of coastal proximity on weather conditions. Analysis of variance with mean separation showed that mean air temperature, mean daily temperature range, mean daily relative humidity range, mean daily solar flux, and mean hourly wind speed were generally greater for sites farther from the coast. For example, mean daily air temperature was 12.3°C at coastal Jonesport, 14.0°C at Jonesboro, 15.9°C at Deblois, and 17.4°C at the most inland Newport site. These results are consistent with the work of CitationLeighly (1947) who reported similar geographic temperature gradients approximately perpendicular to the Maine coast during July. Mean daily air temperature fluctuations at Jonesport, Jonesboro, Deblois, and Newport for the 4-year period were 8.1°C, 11.1°C, 13.6°C, and 10.7°C, respectively. With the exception of the Newport site, the mean daily air temperature range increased with greater distance to the coast. Similarly, CitationLeighly (1947) reported July mean daily air temperature fluctuations for the coastal cities of Portland and Eastport of 8.9°C and 9.3°C, respectively. Mean daily air temperature and mean daily air temperature-range varied along a geographic continuum to the coast that was likely the result of the ocean's cooling and moderating effect during the summer months. The lower than expected mean daily air temperature range found for the most inland Newport site may have been due in part to the moderating effect of two nearby fresh water lakes.
TABLE 2 Selected May Through August Weather Variables at Four Study Sites of Varying Distance to the Maine Coast
Mean daily solar flux values rose significantly from 17.2 MJ/m2 at the most coastal site to a high of 21.9 MJ/m2 at the most inland site, likely reflecting a decrease in cloud cover with distance from the coast. Over this same geographic gradient, mean daily wind speed increased from 1.85 m/s at Jonesport, to 2.23 m/s at Jonesboro, and to 2.46 m/s at Deblois. This pattern was somewhat counterintuitive as wind speeds would generally be expected to be greater closer to the coast. Many microscale climatic factors, such as surrounding tree height and instrument orientation, influence wind speed at 2 m in elevation and may have played a role in these findings. Although annual precipitation is typically greater at the coast, maritime suppression of summer thunderstorm activity often results in coastal areas receiving similar or even slightly less precipitation than inland areas during the Maine growing season (CitationDavis, 1966). This was likely reflected in the statistically similar mean precipitation amounts received at the different sites during the study period ().
ETo
gives the mean weekly Penman–Montieth ETo rates calculated for each month of the growing season from data collected at Jonesport, Jonesboro, Deblois, and Newport. The high standard deviation values associated with each mean illustrates the variability in daily weather for each month. Despite the high variability, analysis of variance, and Tukey's mean separation revealed many significant differences among weekly mean ETo rates. The two most coastally proximate sites had mean weekly ETo rates for May and June that were statistically similar. For May, mean weekly ETo rates (along with standard deviations) at Jonesport and Jonesboro were 2.16 cm/week (0.85 in/week) and 2.31 cm/week (0.91 in/week) and, for June, were 2.47 cm/week (0.97 in/week) and 2.71 cm/week (1.07 in/week), respectively. For July and August, the mean weekly ETo rates at Jonesport were significantly lower than those at all other sites. Conversely, the farthest inland site at Newport had the highest mean weekly ETo rates for the months of June (3.42 cm/week [1.35 in/week]), July (4.23 cm/week [1.67 in/week]), and August (3.51 cm/week [1.38 in/week]). For the month of May, Newport's mean weekly ETo rate of 2.77 cm/week (1.06 in/week) was similar to the mean weekly rate of 2.81 cm/week (1.11 in/week) at Deblois, the next most inland site. Disparities in mean ETo rates between sites generally increased later in the growing season, when air temperatures and solar flux differences between coastal and inland sites would be expected to be greatest. The highest mean ETo rate for all stations was recorded during the month of July. These results clearly indicate that potential water demand increased with increasing distance from the Maine coast.
TABLE 3 Mean Monthly ETo Rates (cm/week) at Varying Distances to the Maine Coast (2005–2008)Footnote z
Fog Occurrence and Deposition
Fog and mist are surface clouds that are generally classified according to how much they act to reduce outdoor visibility. By international agreement, visibility during a “fog” event is less than 1 km; however, the limit of visibility during a “mist” event is less well established. In this study, we considered mist to be defined as visibility greater than 1 km but less than 10 km. Along the Maine coast, south winds are prevalent in summer, while east winds typically dominate in the winter (CitationKimball et al., 1988). As a result, advection fog and mist are common occurrences along the immediate Maine coast during the summer months as warm air masses from the south and southwest pass over the cold waters of the Gulf of Maine (CitationDavis, 1966). illustrates the monthly distribution of fog hours for the Jonesport, Jonesboro, and Deblois research stations during the growing seasons of 2005 through 2008. The monthly distribution of fog was similar for all stations, with a greater frequency in the summer, and a peak during the month of July.
FIGURE 1 Monthly frequency distribution of fog (visibility <1 km) hours at three Maine lowbush blueberry sites (visibility data not available for Deblois during the 2006 growing season).
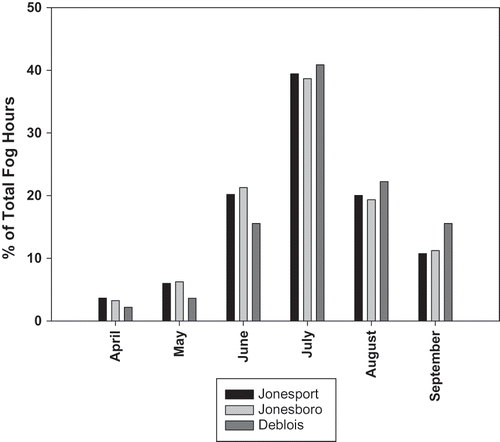
During the 4-year period given in , the most coastal research station at Jonesport recorded fog an average of 11.1% of the time. In contrast, fog was recorded at the most inland Deblois station approximately 3.7% of the time and, at the intermediate Jonesboro station, about 7.0% of the time. This pattern of decreased fog incidence with increasing distance to the coast is consistent with the expectation that advection fog dissipates rapidly with distance from the source. CitationYin and Arp (1994) analyzed meteorological data from a number of studies conducted within the southern Canadian Maritime provinces, an area affected by the same advection fog source as the Down East coast of Maine. Using multiple regression analysis, they found that distance from the coast, month, and air temperature explained 87% of the variance in fog/mist occurrence. Employing the same mist criterion as used in our study, they determined that fog comprised about 40% of all total fog and mist occurrences in the southern Canadian Maritime provinces. That finding was consistent with results observed at the Jonesboro research station, where fog (visibility <1 km) comprised on average about 40% of all instances of visibility less than 10 km. In contrast, at coastal Jonesport and inland Deblois, fog comprised about 57% and 32%, respectively, of all such episodes of low visibility. Thus, in the present study, hours of restricted visibility were more likely to be classified as fog events closer to the coast and mist events farther from the coast.
TABLE 4 Percentage of Fog and Mist Hours at Three Blueberry Fields of Varying Distance to the Maine Coast (2005–2008)
It is difficult to accurately determine the amount of water contributed by fog to particular ecosystems. Artificial fog catchers have been employed for such purposes; however, because of the unique nature of a plant species' leaf surface and canopy structure, such measurements often yield only estimates of potential water capture. Alternative methods, such as eddy covariance measuring systems (CitationVermeulen, et al., 1997), stable isotope analysis (CitationIngraham and Matthews, 1995), and throughfall measurements (CitationLlyod et al., 1988), have all met with varying degrees of success but require specialized, dedicated equipment and/or significant assumptions with respect to the surface area or canopy structure of a particular plant system. On the other hand, weighing lysimeters used in conjunction with visibility measurements allow direct quantification of water input and output during periods of fog and mist. With these tools, the water balance of a lysimeter plant/soil system can accurately be tracked during fog events, thereby eliminating the need for assumptions and/or complex modeling. Still, the quantitatively small input from each individual fog event and the confounding effect of the simultaneously occurring process of ET make direct calculation of actual water deposition during single fog events difficult. However, the overall seasonal effect of fog on the simultaneous deposition of water and suppression of ET during non–rain hours can be approximated through a comparison of lysimeter weight changes in the presence and absence of fog. Results from such calculations are best expressed in water equivalent amounts, defined here as the magnitude of positive water balance during foggy conditions relative to non–foggy conditions. This approach recognizes the impact of fog on ecosystems beyond direct water deposition, such as fog's role in reducing ET through reduced air temperature and solar radiation input (CitationEugster et al., 2006; CitationRitter et al., 2008).
Water equivalent inputs to the coastal Jonesport and intermediate Jonesboro sites averaged 18.3 cm and 13.8 cm per growing season, respectively, for the 4-year period, 2005–2008 (). CitationStarr and Yarborough (2006) calculated an average daily water input rate of 0.075 cm/day from vapor deposition at the Jonesboro station from June 11 through October 8, 2003. When prorated for the 183-day annual growing season, the resulting total water input of 13.7 cm from fog reported by CitationStarr and Yarborough (2006) agrees well with the 4-year average growing season water equivalence of 13.8 cm calculated for Jonesboro in this study ().
FIGURE 2 Water equivalent contributed to weighing lysimeters during episodes of fog (visibility <1 km). (Water equivalent was not calculated for the Deblois research station for the 2005–2006 growing seasons due to insufficient precipitation (2005) and visibility (2006) data.)
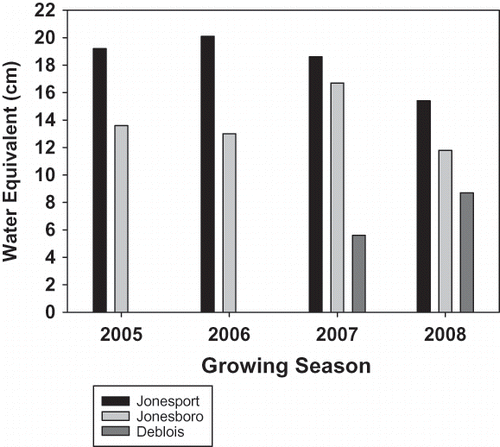
Growing season water equivalent inputs to the inland site at Deblois averaged 7.2 cm for the 2 years of available data (). Water equivalence and fog incidence were well correlated (Pearson r = 0.860). The average ratio of water equivalence to percent fog incidence for Jonesport, Jonesboro, and Deblois was 1.65, 1.97, and 1.93, respectively. Thus, for sites in Down East Maine, it may be possible to estimate fog water equivalence inputs by multiplying the percentage of fog hours by the region-appropriate ratio given above. Interestingly, when ET rates during hours of mist were compared with ET rates during hours of unlimited visibility, no significant differences were found. This suggests that mist alone has a limited effect on the water budget of the Maine lowbush blueberry.
Fog water equivalence during the 2005–2008 growing seasons represented 18.6% and 29.3% of precipitation input at Jonesboro and Jonesport, respectively. Previous estimates of fog's percentage contribution to the water budgets of various fog-affected ecosystems around the world vary widely with environment and experimental methodology. CitationChang et al. (2006) calculated fog deposition to a mountainous forest in northern Taiwan as 10% of annual precipitation, while fog deposition to high-altitude Norway Spruce stands in Germany were estimated to vary between 22% and 28% of annual precipitation (CitationZimmerman and Zimmerman, 2002). A 3-year isotopic study by CitationDawson (1998) determined that fog-drip off redwood trees contributed about 34% of the annual water input to northern coastal redwood forest soils. In a more analogous study, CitationYin and Arp (1994) calculated fog deposition in the southern Canadian Maritimes as only 5%–8% of recorded annual precipitation. The discrepancy in the estimates of fog's relative importance to the water budget in that study and the current study may be due to a number of factors. First, Yin and Arp's results were based on annual data, whereas those of this study were based upon growing season data only—a period when precipitation is lowest and fog occurrence is greatest. Second, those authors considered the effect of fog on ET reduction (up to 6%) separately, while ET suppression and water deposition are quantitatively combined in this study. It is clear from the results of this study that advection fog adds to the coastal proximity differences observed in the potential water demand of the lowbush blueberry.
Additional Considerations
In addition to lower climatic water demand and greater fog occurrence during the growing season, ocean-spray salt deposition is another consequence of immediate coastal proximity. No studies have been done on the effect of growing season sea salt deposition on the health of lowbush blueberry plants. However, CitationEaton et al. (2004) found that salt spray deposition during winter months reduced the yield of exposed lowbush blueberries by damaging buds. Those authors found fruit yield reductions to be variable and highly dependent upon location and degree of crop sheltering through natural barriers such as trees and snow cover. It is likely that the effects of growing season salt deposition on crop health are also strongly dependent on local microclimatic conditions.
As previously described, the general effect of fog in Down East, Maine, is to increase available soil water through both direct deposition and ET suppression. An often overlooked consequence of fog, however, is its effect upon soil and plant health. The chemistry of fog, and occult precipitation in general, has been found to be significantly more acidic and higher in atmospheric pollutants than rain (CitationWaldman et al., 1982). A 1988 study by Kimball et al. found that Maine coastal fog, corrected for airborne marine salts, was on average three times higher in concentrations of [H+] and [NO3 −] ions than mountain fog from Maine and New Hampshire. According to that study, atmospheric pollutants originating from industrial areas to the south and southwest react with advection fog within the Gulf of Maine, sometimes even acting as fog condensation nuclei. As a result, Maine coastal fog is typically higher in nitrates and sulfates, and lower in pH, than fog found in other nonindustrial regions of the continental United States. In addition, a recent study has shown that contrary to expectations and conditions found in most other places within the continental United States, soils of central New England and Maine continue to acidify (CitationWarby et al., 2009). These concerns may be of less importance to acid-tolerant species such as the lowbush blueberry, but need to be mentioned in any discussion of the effects of fog on plant growth.
Actual ET
summarizes the mean weekly actual ET rates for the months of May through August at seven lysimeter stations of varying distances from the Maine coast. The results, determined from weighing lysimeter data recorded during hours of no rain or irrigation, are presented on a crop and prune-year rotation basis. There were 4 months where the mean weekly ET rates for crop-year plants were statistically greater than those for prune-year plants at the same station. Despite the generally numerically higher ET rates of crop plants relative to prune plants during the months of May through August, in most cases, the differences between the rotation cycles were not statistically significant. Not surprisingly, due to the severe nature of pruning, significant differences in mean weekly ET rates between rotation cycles were observed during the month of April (data not shown) for most stations. However, because April in Maine is typically characterized by high rainfall, low climatic water demand, and high soil moisture content, rotational differences in plant water needs during that month do not typically affect irrigation management.
TABLE 5 Mean Monthly Actual ET Rates (cm/week) at Varying Distances to the Maine Coast (2005–2008)
As with the ETo rates given in , ET rate generally increased with distance from the coast (). For crop years, mean weekly ET rate was higher at the inland lysimeter stations of Northfield and Deblois than at the coastal Jonesport station for all months, while the weekly ET rate at Addison was higher than at Jonesport from June through August. Even the two Jonesboro lysimeter stations, located less than 6 km farther inland, had higher crop ET rates than those recorded for the Jonesport station in July and August ().
In analyzing these data, we determined that there were numerous individual days at each station for which a daily ET rate could not be calculated. Comparing ET rates between sites using nonidentical days, as shown in , does not control for daily climatic demand variability and, thus, reduces the overall sensitivity of the data. Restricting comparisons between stations to days in which each station has a calculated daily ET rate (“days in common” analysis) eliminates this large source of variation and allows for a better analysis of the effect of distance from the coast on ET rate. illustrates the mean weekly ET rates for the five sites using 84 days in common during the months of May through August, 2008. Data from the two sites at both Jonesboro and Deblois were pooled for each site. When viewed in this manner, the lysimeter stations at Jonesboro, Addison, and Deblois all had similar mean overall ET rates of 1.83 cm/week, 1.83 cm/week, and 1.98 cm/week, respectively, while the station at coastal Jonesport (∼0.8 km) had the lowest mean ET rate of 1.61 cm/week, and the inland station at Northfield (∼25.7 km) had the highest mean rate of 2.32 cm/week. Thus, for 2008, three distinct ET regimes existed, and the difference in mean ET rate between the most coastal and the most inland site was 0.71 cm/week. Although the mean ET rate at Deblois was 0.15 cm/week greater than that at the intermediate coastal sites of Jonesboro and Addison, the sample size was too small and the deviations from the means too great for these numerical differences to be statistically significant.
FIGURE 3 Overall mean actual weekly ET rates for lowbush blueberry at five Maine field sites for 84 “days in common” during the period of May through August, 2008.
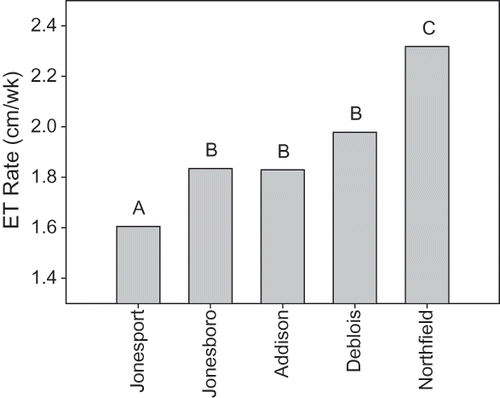
Similarly, if the limitation of using only 2008 data is removed (the comparison therefore excludes Northfield), additional statistical differences between the remaining sites can be discerned. The mean ET rates of four sites using an expanded sample set consisting of 138 May through August days in common from the growing seasons of 2006 through 2008 are shown in . In this larger data set, the difference in mean ET rate between the Jonesport station and the pooled Deblois sites was 0.60 cm/week. This difference is similar to the 0.71 cm/week difference between the Jonesport and Northfield sites observed using 84 days of data. Significant differences in mean ET rate between the pooled Jonesboro and Deblois sites are also delineated. For the 138 days in common, the ET rate at Jonesboro averaged 2.10 cm/week while the average Deblois rate was statistically greater at 2.28 cm/week. Thus, not only were comparisons based on days in common data effective in probing the relationship between ET rate and costal proximity; increasing the number of such comparisons increased the resolution of the analysis.
FIGURE 4 Overall mean actual weekly ET rates for lowbush blueberry at five Maine field sites for 138 “days in common” during the period of May through August, 2006–2008.
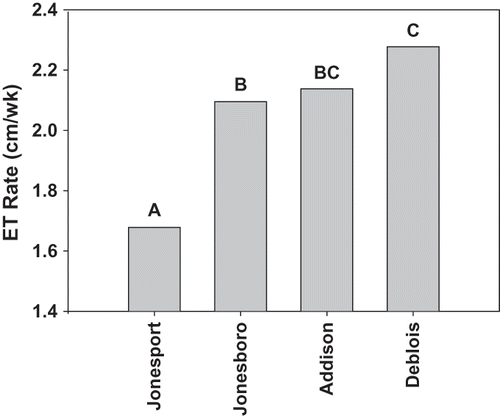
The use of irrigation might intuitively be expected to increase the amount of water available for plant use, and this, in turn, would likely be reflected in greater ET rates for irrigated sites. Although this study was not specifically designed to compare ET rates between irrigated and unirrigated fields, our data showed no evidence of an irrigation effect. As and and demonstrate, mean ET rates at the proximal Jonesboro and Addison stations were similar, despite the former being irrigated and the latter not being irrigated. Both stations have usable data extending back to 2005, which allows for a more comprehensive comparison of the two sites. In utilizing over 310 days in common for the months of May through August, 2005–2008, the mean ET rates for Jonesboro and Addison were found to be 1.98 cm/week and 1.94 cm/week, respectively. Thus, despite the application of a growing season average of 3.8 cm and 2.6 cm of irrigation water to Jonesboro crop and prune fields, respectively, mean ET rates at the proximal Jonesboro and Addison sites were nearly identical over a 4-year period.
In contrast, an expanded comparison, using 244 days from the months of May through August 2006–2008 of the more geographically distant Jonesboro and Deblois sites, revealed significant differences in the mean ET rates between the sites despite the fact that both were irrigated. During the 2008 growing season, tensiometers installed at all seven sites generally indicated similar water potentials at the sites, except during brief periods of drought when the irrigated fields had greater potentials. Because of the number of variables involved, it is not possible to quantitatively separate the effects of lowered water availability during these times with calculated ET rates. In addition, as shows, differences in soil texture, organic matter content (upper 10 cm), and pruning method varied among the sites. Nevertheless, the results of this study show small but statistically significant differences in ET rate between sites that appear primarily related to coastal proximity. A study will be conducted during the 2009 growing season at these same sites to quantitatively ascertain: (a) the relationship between soil water potential and ET rate and (b) the effects of mulching on atmospheric water retention and soil water availability.
Crop Coefficients
Previous research using 3 years of data from Jonesboro determined a growing season, lowbush blueberry crop coefficient (standard condition) of ∼0.69 (CitationHunt et al., 2008). The current research provides results based on both a 4th year of data from Jonesboro, as well as 3 years of data from a more inland site. For Jonesboro alone, the 4-year May through September standard condition crop coefficient was found to average 0.68 (99% confidence interval: 0.65–0.71), a result consistent with that of the previous study. Similarly, a 3-year May through September standard condition crop coefficient value for inland Deblois was determined to be 0.68 (99% confidence interval: 0.63–0.73). Thus, for these two stations, the effects of coastal proximity on ETo and actual ET appear to balance, resulting in similar crop coefficient values for the two locations. This suggests that for the irrigated lowbush blueberry, a standard condition, single-value crop coefficient of ∼0.68 can be used throughout the growing region in Eastern Maine.
For the coastal Jonesport station, the results from 4 years of data gave a lower crop coefficient of 0.63 (99% confidence interval: 0.58 to 0.68). However, because irrigation was absent from this site, no assurances can be made that sufficient soil water was consistently available there to meet plant ET demands—a crucial assumption in the development of standard condition crop coefficients. Therefore, this crop coefficient value for extreme coastal environments should be used with caution. Additional research will be conducted in 2009 to quantitatively determine the effect of soil moisture on weighing lysimeter-determined ET rates and crop coefficients.
SUMMARY AND CONCLUSIONS
A 4-year study was conducted to compare both climatic demand and actual lowbush blueberry ET rates using weighing lysimeters at four irrigated sites and three nonirrigated sites of varying distance from the Maine coast. Mean daily air temperature, relative humidity, and solar flux values were all found to increase with distance from the coast. Penman–Montieth grass ETo results reflected these differences, as sites farther from the coast had significantly higher ETo rates than those closer to the coast. The effects of summer advection fog on ET suppression were also determined using both weighing lysimeter and visibility data. Fog was found to occur more often near the coast and to contribute more to the water budgets at coastal sites than at inland sites. Water equivalent input (water deposition/ET suppression) at the most coastal site was more than 2.5 times greater than that determined for a site just over 20 km farther inland. Actual ET rates at all sites were generally found to be similar for prune and crop fields from May through August. As with climatic water demand, higher ET rates were found with increasing distance from the coast. The results of this study indicate that fog and proximity to the coast play an important role by reducing water demand of the Maine lowbush blueberry.
By lowering the rates of both ETo and ET simultaneously, the extent of this geography related ET suppression is reflected in the calculated Kc values. The May through August standard condition Kc value for two irrigated sites located 6.4 km and 22.5 km from the coast was found to be 0.68. This Kc value can be used in conjunction with ETo data to provide Maine lowbush blueberry growers with an enhanced ability to predict crop water demand (CitationHunt et al., 2008). Additional research is currently being conducted to determine how organic mulching and soil properties affect ET rates and Kc values of the Maine lowbush blueberry.
ACKNOWLEDGMENT
We thank the Wild Blueberry Commission of Maine for their financial support; and we thank Ben Lagasse, Eric Lowell, and Peggy Pinette for their technical assistance in conducting this research.
Notes
G. Starr was formerly with USDA-ARS New England Plant, Soil, and Water Laboratory; Orono, Maine, USA.
LITERATURE CITED
- Allen , R.G. , Jenson , M.E. , Wright , J.L. and Burman , R.B. 1998 . Operational estimates of reference evapotranspiration . Agron. J. , 81 : 650 – 662 .
- Chang , S.C. , Yeh , C.F. , Wu , M.J. , Hsia , Y.J. and Wu , J.T. 2006 . Quantifying fog water deposition by in situ exposure experiments in a mountainous coniferous forest in Taiwan . Forest Ecol. Mgt. , 224 : 11 – 18 . DOI: 10.1016/j.foreco.2005.12.004
- Dalton , T.J. , Files , A. and Yarborough , D. 2002 . “ Investment, ownership, and operating costs of supplemental irrigation systems for Maine wild blueberries ” . In Maine Agr. Forest Expt. Sta. Technol. Bul. 183 Orono, ME
- Davis , R.B. 1966 . Spruce-fir forests of the coast of Maine . Ecol. Monogr. , 36 : 80 – 94 .
- Dawson , T.E. 1998 . Fog in the California redwood forest: Ecosystem inputs and use by plants . Oecologia , 117 : 476 – 485 . DOI: 10.1007/s004420050683
- Eaton , L.J. , Sanderson , K.R. and Hoyle , J. 2004 . Effects of salt deposition from salt water spray on lowbush blueberry shoots . Small Fruits Rev. , 3 : 95 – 103 . DOI: 10.1300/J301v03n01_10
- Eugster , W. , Burkard , R. , Holwerda , F. , Scatena , F.N. and Bruijnzeel , L.A. 2006 . Characteristics of fog and fogwater fluxes in a Puerto Rican elfin cloud forest . Agr. For. Meteorol. , 139 : 288 – 306 .
- Handley, D.T. and M.G. Hutton. 2007. University of Maine cooperative extension plan of work issue area needs assessment 2007–2011. http:www.umext.maine.edu/POW/POW2007/issuepapers/Agriculture-Crop%20Production.doc
- Hunt , J.F. , Honeycutt , C.W. , Starr , G. and Yarborough , D. 2008 . Evapotranspiration rates and crop coefficients for lowbush blueberry (Vaccinium angustifolium) . Intl. J. Fruit Sci. , 8 : 282 – 298 . DOI: 10.1080/15538360802597549
- Ingraham , N.L. and Matthews , R.A. 1995 . The importance of fog-drip water to vegetation: Point Reyes Peninsula, California . J. Hydrology , 164 : 269 – 285 . DOI: 10.1016/0022-1694(94)02538-M
- Joseph , J.A. , Denisova , N.A. , Arendash , G. , Gordon , M. , Diamond , D. , Shukitt-Hale , B. and Morgan , D. 2003 . Blueberry supplementation enhances signaling and prevents behavioral deficits in an Alzheimer disease model . Nutr. Neuroscience , 6 : 153 – 162 .
- Kalt , W. , Foote , K. , Fillmore , S.A. , Lyon , M. , Van Lunen , T.A. and McRae , K.B. 2008 . Effect of blueberry feeding on plasma lipids in pigs . British J. Nutr. , 100 : 70 – 78 .
- Kimball , K.D. , Jagels , R. , Gordon , G.A. , Weathers , K.C. and Carlisle , J. 1988 . Differences between New England coastal fog and mountain cloud water chemistry . Water Air Soil Pollut. , 39 : 383 – 393 .
- Leighly , J.B. 1947 . Profiles of air temperatures normal to coast lines . Ann. Assoc. Amer. Geograph. , 37 : 75 – 86 .
- Lloyd , C.R. , Gash , J.H.C. , Shuttleworth , W.J.U. , Marques , F. and De , O.F. 1988 . The measurement and modeling of rainfall interception by Amazonian rain forest . Agr. For. Meteorol. , 43 : 277 – 294 .
- Ritter , A. , Regalado , C.M. and Aschan , G. 2008 . Fog water collection in a subtropical elfin laurel forest of the Garajonay National Park (Canary Islands): A combined approach using artificial fog catchers and a physically based impaction model . J. Hydrometerol. , 9 : 920 – 935 . DOI: 10.1175/2008JHM992.1
- Schmidt , B.M. , Howell , A.B. , McEniry , B. , Knight , C.T. , Seigler , D. , Erdman , J.W. and Lila , M.A. 2004 . Effective separation of potent antiproliferation and antiadhesion components from wild blueberry (Vaccinium angustifolium Ait.) fruits . J. Agr. Food Chem. , 52 : 6433 – 6442 .
- Starr , G. , Seymour , R.M. , Olday , F. and Yarborough , D.E. 2004 . Determination of evapotranspiration and drainage in lowbush blueberries (Vaccinium angustifolium) using weighing lysimeters . Small Fruits Rev. , 3 : 273 – 283 . DOI: 10.1300/J301v03n03_04
- Starr , G.C. and Yarborough , D.E. 2006 . Influence of vapor deposition on lowbush blueberry water requirements in a humid coastal climate . Acta Hort. , 715 : 323 – 328 .
- Storlie , C.A. and Eck , P. 1996 . Lysimeter-based crop coefficients for young highbush blueberries . HortScience , 31 ( 5 ) : 819 – 822 .
- University of Maine. 2009. Wild blueberry fact sheet. http:www.wildblueberries.maine.edu/PDF/Miscellaneous/CropStatistics.pdf
- Vermeulen , A.T. , Wyers , G.P. , ömer , F.G. R , Van Leeuwen , N.F.M. , Draaijers , G.P.J. and Erisman , J.W. 1997 . Fog deposition on a coniferous forest in The Netherlands . Atmos. Environ. , 31 : 375 – 386 .
- Waldman , J.M. , Munger , J.W. , Jacob , D.J. , Flagan , R.C. , Morgan , J.J. and Hoffmann , M.R. 1982 . Chemical composition of acid fog . Science , 218 : 677 – 680 .
- Warby , R.A.F. , Johnson , C.E. and Driscoll , C.T. 2009 . Organic soils continue to acidify despite reduction in acidic deposition . Soil Sci. Soc. Amer. , 73 : 274 – 284 . DOI: 10.2136/sssaj2007.0016
- Yin , X. and Arp , P.A. 1994 . Fog contributions to the water budget of forested watersheds in the Canadian Maritime provinces: A generalized algorithm for low elevations . Atmosphere Ocean , 32 ( 3 ) : 553 – 566 .
- Zimmermann , L. and Zimmermann , F. 2002 . Fog deposition to Norway Spruce stands at high elevation sites in eastern Erzgebirge (Germany) . J. Hydrol. , 256 : 166 – 175 .