Abstract
Growth of cultivated Vaccinium species on soils with a pH above 6.0 is inhibited and production can be extended to these types of soils only after extensive soil modifications. V. arboreum is a wild species that grows on soils with higher pH values. The aim of the present study was to characterize differences in nitrate and iron assimilation in cultivated vs. wild Vaccinium. Genotypes from V. corymbosum and V. arboreum were grown hydroponically with different nitrate and iron concentrations. Nitrate and iron uptake were significantly greater in V. arboreum compared with V. corymbosum. This was correlated with increased nitrate reductase and ferric chelate reductase activities and increased tissue concentrations of reduced nitrogen and iron in V. arboreum. The wild species, V. arboreum, clearly exhibits an increased ability to assimilate nitrate and iron compared with V. corymbosum. This greater capacity for assimilation may play a significant role in this species' broader soil adaptation.
INTRODUCTION
Production of cultivated Vaccinium species is restricted to very narrow soil types; specifically, soils with acidic pH and high organic matter, readily available iron, and nitrogen in the form of ammonium rather than nitrate (CitationRosen et al., 1990; CitationWilliamson and Lyrene, 1998). Such soils are limited and are often located in or adjacent to wetlands and blueberry growers have expressed concern over wetland regulatory policies that affect development of potential production sites (CitationStrik and Yarborough, 2005).
Growth and yield of cultivated Vaccinium on more typical agricultural soils—i.e., drier, higher pH, lower organic matter, decreased iron availability, and nitrogen primarily in the nitrate form—is markedly reduced. This may be related to decreased iron availability at higher pH and low rates of nitrate uptake in Vaccinium species (CitationMerhaut and Darnell, 1995; CitationPoonnachit and Darnell, 2004; CitationRosen et al., 1990). Production can be extended to these types of soils only after extensive and costly soil modifications, such as mulching, incorporation of peat or pine bark, and soil acidification. Additionally, frequent irrigation is required, since most cultivated Vaccinium species have shallow, fibrous root systems and cannot tolerate dry soils (CitationWilliamson and Miller, 2009). Without such soil modifications, iron and/or nitrogen deficiency symptoms are exhibited (CitationBrown and Draper, 1980; CitationKorcak et al., 1982). However, soil amendments and mulches may immobilize nitrogen, requiring nitrogen fertilization rates in excess of 3-fold higher than those recommended for traditional soil culture for optimum productivity (CitationWilliamson and Miller, 2009). Thus, cultivation in these soil amendments is expensive—even cost-prohibitive in some cases—and is not ecologically sound.
There are several species of wild Vaccinium that appear to have increased tolerance to more typical agricultural soils compared with cultivated Vaccinium. Most notable is V. arboreum Marsh., the sparkleberry, which is drought resistant and more tolerant of higher pH (6.0–6.5) and lower organic matter than most Vaccinium species (CitationBallington, 1998; CitationLyrene, 1997). V. arboreum also thrives on sandy-clay hills in the southeastern U.S., which are typically low in nutrients, and is commonly the only Vaccinium species found in association with Liquidambar styraciflua, Magnolia grandiflora, and Carya glabra, trees not found on highly-acid soils (CitationCamp, 1945).
The ability of V. arboreum to tolerate dryer, higher pH soils may be related to increased efficiency for nitrate and/or iron assimilation compared with cultivated Vaccinium. Nitrate uptake and assimilation is regulated by nitrate reductase (NR) activity (CitationTouraine et al., 2001), while the rate limiting enzyme for iron uptake and assimilation is considered to be iron chelate redutase (CitationGrusak et al., 1993). Our previous work indicated that V. arboreum consistently exhibits higher rates of root NR activity and greater nitrate uptake than does V. corymbosum, one of the cultivated species (CitationDarnell and Hiss, 2006; CitationPoonnachit and Darnell, 2004). Neither species exhibits measurable leaf NR activity, indicating that root activity is the primary regulation point for nitrate assimilation in these Vaccinium species. Iron uptake is also greater in V. arboreum than in V. corymbosum and is correlated with higher root ferric chelate reductase (FCR) activity (CitationDarnell and Hiss, 2006).
Although root FCR activity is often well correlated with iron uptake, iron concentration in leaves is not always correlated with development of leaf chlorosis. In fact, leaves of many iron deficient plants have total iron concentration similar to those of iron sufficient leaves, but the internal transport steps of iron appear to be impaired by iron deficiency (CitationGonzalez-Vallejo et al., 1998). This phenomenon has been referred to as “chlorosis paradox” (CitationRomheld, 2000) and it has been suggested that a leaf iron reductase plays a critical role in iron availability by allowing reduction and subsequent transport of iron from the leaf apoplast into the cell (CitationMengel, 1994). Iron chelate reductase activity has been documented in several plant species and recently, expression of a leaf plasmamembrane FCR has been observed (as cited in CitationJeong and Connolly, 2009). However, no work has examined leaf FCR activities in Vaccinium species, which often exhibit the “chlorosis paradox.”
It was hypothesized that V. arboreum is more efficient at both iron and nitrate uptake and assimilation than is V. corymbosum, and this allows V. arboreum to successfully establish on typical agricultural soils where the higher pH may limit iron acquisition and also increase nitrification (CitationMengel, 1994), resulting in higher levels of soil nitrate. The objectives of the present study were to determine rhizosphere nitrate and iron concentration effects on root NR activity and root and leaf FCR activity, nitrate and iron uptake, and tissue concentrations of nitrogen and iron in the cultivated V. corymbosum and the wild V. arboreum.
MATERIALS AND METHODS
Seeds of the wild species, V. arboreum, were collected from a natural habitat of distinct plants (most likely representing a single genotype) at Jennings Forest, FL in winter 2006 and germinated on the surface of Canadian peat with intermittent mist under 60% shade in a greenhouse, with average daily temperatures of 25°C and photosynthetic photon flux (PPF) of 545 μmol m−2 s−1. Propagation by seed was required since previous work indicated very low success rate with propagation by stem cuttings. In spring 2007, shoot cuttings of V. corymbosum interspecific hybrid ‘Springwide’ were rooted in peat:perlite (1:1 by volume) medium with intermittent mist under 60% shade in a greenhouse with average temperatures of 25°C and PPF of 545 μmol m−2 s−1. As germination and rooting proceeded, plants of both species were transplanted to 1-L pots containing pine bark: peat (3:1) and maintained in a greenhouse with average temperatures of 22°C and PPF of 1700 μmol m−2 s−1. In November 2007, all plants were root pruned (approx. 25%) and repotted in1-L pots containing pine bark: peat (3:1). In mid-January 2008, 20 plants of each species were selected and whole plant fresh weights determined. Initial whole plant fresh weights averaged 21.1 g and 92.6 g for V. arboreum and V. corymbosum, respectively. Plants were blocked by size and transferred into 2-L plastic bottles filled with a complete nutrient solution. The bottles were wrapped with aluminum foil to eliminate light infiltration. The nutrient solution contained (mM): 0.5 K2HPO4, 1.0 MgSO4, 0.5 CaCl2, 0.045 H3BO3, 0.01 MnSO4, and 0.01 ZnSO4, with 0.3 μM CuSO4 and 0.2 μM Na2MoO4. Iron was supplied as Fe-diethylenetriaminopentaacetic acid (Fe-DTPA) at 10 or 90 μM. Nitrogen was supplied as KNO3 at either 1.0 or 0.5 mM. For the 0.5 mM KNO3 treatment, 0.5 mM KCl was added to balance K+ in both nutrient solution treatments. The nutrient solutions were buffered at pH 5.5 with 5 mM 2-(4-morpholino)-ethane sulfonic acid (MES). This pH was chosen since it is in the optimum range for both species and pH was not a variable in this experiment. Aeration (1 L min−1) was provided to each bottle by an aquarium pump (Elite 801, Rolf C. Hagen, Mansfield, MA, USA), connected by tygon tubing. Nutrient solutions were changed weekly and whole plant fresh weights were taken at every other solution change throughout the experiment in order to calculate nitrate and iron uptake on a g fresh weight basis.
Nitrate and iron uptake were determined weekly by measuring depletion from the nutrient solutions. For nitrate uptake, 10 μL of the nutrient solution was taken from each sample bottle before the solutions were changed each week and diluted with 1.5 ml of distilled water. Concentrated HCl (15 μL of 12.1 N) was added and samples were vortexed before reading spectrophotometrically (Shimadzu UV-160, Kyoto, Japan) in quartz cuvettes at 210 nm. Iron uptake was determined using an atomic absorption spectrophotometer (Perkin Elmer 3030B, Norwalk, CT, USA) with a hallow cathode lamp and air-acetylene flame.
Root NR activity was quantified four times at three-week intervals during the course of the 14-week experiment, starting five weeks after the beginning of treatments. Root tips (1 cm long) were cut, placed in a foil wrapped beaker filled with ice water, and transferred to the lab. Samples were blotted dry, weighed, and about 100 mg fresh weight of tissue was placed in a test tube (two tubes per replicate per treatment). Two mL of assay solution, comprised of 100 mM KH2PO4 (pH 7.5), 30 mM KNO3, and 2% 1-propanol, were added to each test tube. One tube per replicate per treatment was immediately filtered through Whatman No. 2 paper and used as the time 0 control. Samples were vacuum-infiltrated for 5 min, then incubated in a shaking water bath at 31°C for 1 hr in the dark. After incubation, the assay solution containing tissue samples was filtered and a 1 mL aliquot from each sample was removed to a new tube. One mL sulfanilamide (1% w/v in 1.5 N HCl) and 1 mL N-(1-naphthyl)-ethylenediaminedihydrochloride (0.02% w/v in 0.2 N HCl) were added. The samples were incubated at room temperature for 30 min and the absorbance of nitrite at 540 nm was determined spectrophotometrically (Shimadzu UV-160, Kyoto, Japan) (modified from CitationJaworski, 1971).
Root and leaf FCR activities were also quantified four times during the experiment at three-week intervals, beginning 4 weeks after the beginning of treatments. Root tips (1 cm long) or leaf disks (∼4 mm diameter, cut from the most recently matured leaves, avoiding major vascular bundles) were cut, placed in a foil-wrapped beaker filled with ice water, and transferred to the lab. Samples were handled as above and about 100 mg fresh weight of tissue was placed in a test tube. Tissues were rinsed in 0.2 mM CaSO4 for 10 min before transferring to 2 mL assay solution, containing 10 mM CaSO4, 5 mM MES (pH 5.5), 0.1 mM Fe-EDTA, and 0.3 mM sodiumbathophenanthroline disulfonic acid (Na2-BPDS). Leaf tissues were vacuum infiltrated with an assay solution for 10 min. Test tubes containing 2 mL of assay solution without tissue were used as controls. Samples and control tubes were incubated for 1 hr in a shaking water bath at 23°C in the dark. After incubation, a 1-mL aliquot from each tube was transferred into a cuvette and read spectrophotometrically at 535 nm (Shimadzu UV-160, Kyoto, Japan). The concentration of Fe(II)-BPDS produced was calculated using the molar extinction coefficient of 22.14 mM cm−1 (CitationChaney et al., 1972).
After 14 weeks, plants were harvested and root, stem, and leaf fresh weights were determined. Dry weight was determined after oven drying at 70°C to constant weight. Removal of root tips and leaf slices for FCR and NR activity measurements did not appear to have any impact on root or plant growth, as this represented a small fraction (<0.05%) of the total root or leaf fresh weight. Dried samples were ground through a 20-mesh screen in a Wiley Mill (Thomas Scientific, Swedesboro, NJ, USA) and were analyzed for total nitrogen, total iron, and active iron. Active iron is the fraction of iron that is extracted with dilute acids or ferrous chelates and is considered to be a better indicator of tissue iron nutritional status than total iron (CitationSmith and Cheng, 2005). A second sample of dried tissue was ground through a 40-mesh screen and analyzed for nitrate-N.
Total tissue nitrogen was analyzed by combustion (Leco TruSpec CN, St. Joseph, MI, USA). Tissue nitrate was analyzed by the automated cadmium reduction method using a rapid flow analyzer (Flow Solution IV, O-I Analytical, Astoria, OR, USA) at 540 nm (CitationAnderson and Case, 1999). Reduced nitrogen was calculated as the difference between total nitrogen and nitrate.
Total tissue iron was determined using an open vessel-wet digestion procedure. Samples were digested for 20 min in equal volumes of 12 M HNO3 and 14.2 M H2O2. Following digestion, 25 to 50 mL dH2O was added and iron concentration was determined by ICP spectrophotometry (SPECTRO Analytical Instruments, Kleve, Germany). Active iron was determined based on a protocol developed by CitationSmith and Cheng (2006). Briefly, 50 mg of dried tissue was placed in a centrifuge tube with 1 ml 0.1 M HCl, mixed well, and placed on an orbital shaker for 24 hr at 2.0 hertz. Samples were then centrifuged at 10,000 × g for 5 min and the supernatant was drawn into a 1-ml plastic syringe and filtered through a 0.45-um PVDF syringe filter (diameter, 13 mm). A 400-μL aliquot was mixed with 250 μL of fresh 500 mM ascorbic acid and 250 μL 1 mM bathophenanthroline (BP) and allowed to stand for 5 min. Sample pH was raised by adding 400 μL 2.5 M decontaminated sodium acetate (pH 4.5) and mixing vigorously before allowing to stand for 5 min. Five hundred μL of 1-octanol was then added, vortexed, and allowed to stand for 30 min to allow the Fe(II)-BP complex to move into the 1-octanol upper phase. The sample was then vortexed before centrifuging at 1,000 g for 5 min to promote phase separation. An aliquot of the upper phase (containing Fe(II)-BP in 1-octanol) was removed and measured spectrophotometrically at 537 nm (Shimadzu UV1800, Kyoto, Japan). Iron concentration was determined from a standard curve using iron atomic absorption standards (Aldrich Chemical) in 0.1 M HCl.
Treatments were arranged in a 2 × 2 × 2 factorial (species × nitrate concentration × iron concentration) in a randomized complete block design with five replications, using a single plant per replicate. Data were analyzed using SAS Proc GLM (SAS Institute, Cary, NC, USA). Mean separation was performed using Least Square Means (LSM) or t test. Final plant fresh weight and dry weight were analyzed using initial plant fresh weight as a covariate to normalize for differences in initial plant size.
RESULTS
Specific nitrate uptake rates were significantly greater in V. arboreum compared with V. corymbosum starting two weeks after treatments began and continuing throughout the experiment (). Specific uptake rates ranged from 2.0 to 3.7 μmol NO3 per g fresh weight per day for V. arboreum, while rates for V. corymbosum ranged from 0.8 to 1.5 μmol NO3 per g fresh weight per day. There was no significant effect of external iron concentration on nitrate uptake. Increasing external nitrate concentration did not significantly increase specific nitrate uptake rates for all sampling dates, although there was a trend towards increased uptake rates at the higher external nitrate concentration. Because of the increased trend, cumulative nitrate taken up during the experiment was significantly greater at 1.0 compared with 0.5 mM external nitrate (15.7 vs. 9.1 mmol nitrate, respectively).
FIGURE 1 Species effect on specific nitrate uptake rate. External iron and nitrate concentrations had no significant effect on uptake rate, thus data are pooled. Asterisks indicate significant differences in uptake rate, p ≤ 0.05 (n = 20).
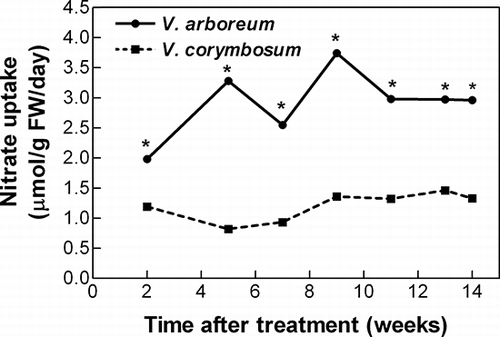
Marked differences in NR activities reflected the differences observed between species in nitrate uptake rates—significantly higher NR activity was found in roots of V. arboreum compared to roots of V. corymbosum throughout the experiment (). Root NR activity in V. arboreum averaged ∼275 nmol NO2 − per g fresh weight per hour, while activity in V. corymbosum roots averaged ∼95 nmol NO2 − per g fresh weight per hour. There were no consistent differences in root NR activity in either species at the two different external nitrate concentrations, although there was a clear trend towards increased activity at the higher external concentration ().
FIGURE 2 Species (A) and external nitrate concentration (B) effects on root nitrate reductase (NR) activity. External iron concentration had no significant effect on NR activity, thus data were pooled across iron concentration and across external nitrate concentration (A) or species (B). Mean separation within time after treatment by t-test, p ≤ 0.05 (n = 20).
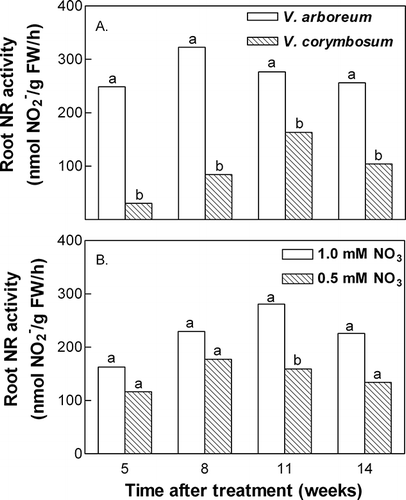
Specific iron uptake was also significantly higher in V. arboreum compared with V. corymbosum throughout the experiment (). Uptake rates in V. arboreum were variable, ranging from 0.02 to 0.04 μmol per g fresh weight per day, while uptake rates in V. corymbosum were relatively stable throughout the experiment, averaging 0.01 μmol per g fresh weight per day. Except for one sampling time (5 weeks after treatments began), specific uptake rates were significantly higher at external iron concentrations of 90 compared with 10 μM (). There was no effect of external nitrate concentration on specific iron uptake rates, nor were there consistent species/external iron concentration interactions on uptake rates.
FIGURE 3 Species (A) and external iron concentration (B) effects on iron uptake. External nitrate concentration had no significant effect on uptake rate, thus data were pooled across nitrate concentration and across external iron concentration (A) or species (B). Asterisks indicate significant differences in uptake rate, p ≤ 0.05 (n = 20).
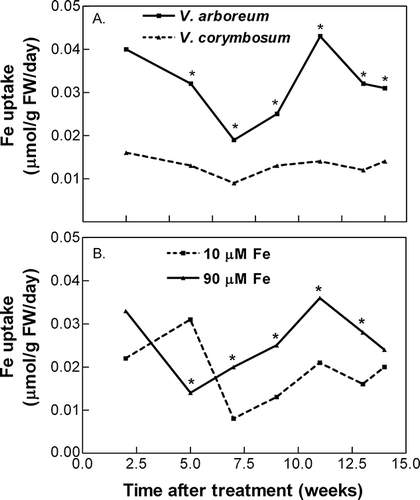
As with nitrate uptake, differences in iron uptake rates were reflected in differences in activities of both root and leaf FCR. Root FCR activity was significantly greater in V. arboreum compared with V. corymbosum by 7 weeks after treatment (), while increased activity of leaf FCR in V. arboreum compared with V. corymbosum was observed by 4 weeks after treatment (). Root FCR activities ranged from ∼160 to 275 nmol Fe2+ per g fresh weight per hour in V. arboreum and from ∼100 to 320 nmol Fe2+ per g fresh weight per hour in V. corymbosum. Leaf FCR activities in both species were considerably lower, averaging 25 to 30% of the activity found in roots.
FIGURE 4 Species effect on ferric chelate reductase (FCR) activity in roots (A) and leaves (B) of V. arboreum and V. corymbosum. External nitrate and iron concentrations had no significant effect on FCR activity, thus data are pooled. Mean separation within time after treatment by t-test, p ≤ 0.05 (n = 20).
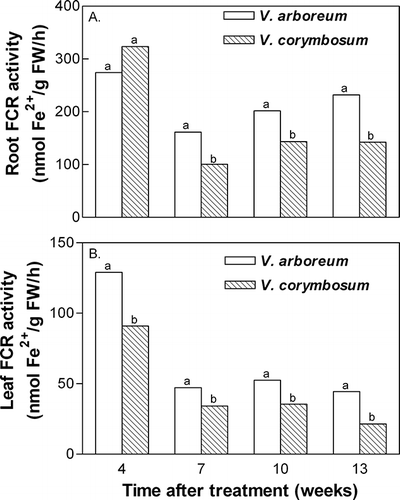
There was a significant interaction between external nitrate concentration and species on plant dry weight (). V. arboreum grown at 1.0 mM external nitrate concentration had the greatest leaf and whole plant dry weights compared to the same species grown at 0.5 mM nitrate, or compared to V. corymbosum grown at either external nitrate concentration. Root dry weights were greater in V. arboreum compared with V. corymbosum regardless of external nitrate concentration, while stem dry weights were lowest in V. arboreum at 0.5 mM nitrate compared with the other three treatments.
TABLE 1 External Nitrate Concentration Effects on Leaf, Root, Stem, and Whole-Plant Dry Weight in V. arboreum and V. corymbosum
Reduced nitrogen concentrations were significantly greater in roots of V. arboreum compared with V. corymbosum at both external nitrate concentrations, while leaf concentrations were greater in V. corymbosum (). There was a significant interaction between external nitrate concentration and species on stem and whole-plant reduced nitrogen concentrations. Stem and whole-plant concentrations in V. arboreum were the highest and were not affected by external nitrate concentration. In contrast, stem and whole-plant reduced nitrogen concentration in V. corymbosum were significantly lower at 0.5 mM compared with 1.0 mM external nitrate. Overall, the whole-plant reduced nitrogen concentration was significantly higher in V. arboreum compared with V. corymbosum.
TABLE 2 External Nitrogen Concentration Effects on Leaf, Root, Stem, and Whole-Plant Reduced Nitrogen and Nitrate-Nitrogen in V. arboreum and V. corymbosum
Organ nitrate concentrations were less affected by external nitrate or species. Stem nitrate concentrations were significantly lower in V. arboreum at 1.0 mM external nitrate compared with the other three treatments, while nitrate concentrations in other organs were similar between species and not affected by external nitrate concentration. There was no effect of external iron concentration on organ nitrogen concentrations.
Total organ iron concentrations were similar between the two species, except in roots, where iron concentration was significantly higher in V. arboreum compared with V. corymbosum (). External iron concentration did not affect leaf or stem iron concentrations, but root iron concentrations were significantly higher at 90 μM compared with 10 μM external iron concentration. There were no significant interactions between species and external iron concentration on total iron concentration in any organ, nor were there any effects of external nitrate concentration on organ iron levels.
TABLE 3 Species and External Iron Concentration Effects on Total and Active Iron Concentration in Roots, Leaves, and Stems
Active iron represented between 10 and 20% of the total iron concentration, depending on organ. Concentrations of active iron were significantly higher in roots, leaves, and stems of V. arboreum compared with V. corymbosum (). External iron concentrations did not affect active iron concentrations in any organ.
DISCUSSION
The ability to assimilate nitrate and iron is clearly greater in V. arboreum than in V. corymbosum under conditions of this experiment, as indicated by the increased activities of NR and FCR in V. arboreum. The average root NR activity in V. arboreum was about 3-fold higher and specific nitrate uptake rates were about 2.5-fold higher than was found in V. corymbosum over the course of the experiment. We have previously reported higher root NR activity and nitrate uptake rates in V. arboreum compared with V. corymbosum (CitationPoonnachit and Darnell, 2004; CitationDarnell and Hiss, 2006) under higher external concentrations of nitrate. The present work shows that a similar response is observed when plants are grown at external nitrate concentrations that are up to 10-fold lower. Although NR activity was significantly greater in V. arboreum, root activities in both Vaccinium species were less than those observed in other woody plant species, such as Juglans nigra (CitationNicodemus et al., 2008) and Quercus robur (CitationThomas and Hilker, 2000). In addition, many woody species also exhibit substantial NR activity in leaves (CitationNicodemus et al., 2008; CitationNatali et al., 2009), and previous studies indicate leaf NR activity is negligible in Vaccinium species (CitationDarnell and Hiss, 2006; CitationPoonnachit and Darnell, 2004). Thus, the total capacity to reduce nitrate in Vaccinium species is low compared to other woody species, and resides primarily in the roots.
Nonetheless, the increased activity of NR in roots of V. arboreum was reflected in increased specific uptake of nitrate throughout the experiment compared with the cultivated V. corymbosum. Higher NR activity also resulted in increased concentrations of reduced nitrogen in roots and whole-plants of V. arboreum compared with V. corymbosum. Since negligible nitrate reduction occurs in leaves of either species, the reduced nitrogen in leaves would be a result of reduction and transport from the roots. The higher concentration of reduced nitrogen in leaves of V. corymbosum compared with V. arboreum may indicate greater transport capacity from roots to leaves or it may reflect less recycling of reduced nitrogen from leaves to roots (CitationSubasinghe, 2007).
Higher NR and reduced nitrogen concentrations in V. arboreum compared with V. corymbosum was also reflected in increased root dry weight in V. arboreum, although this did not translate into increased whole-plant dry weight except at the higher external nitrate concentration. This differs from previous work where higher external concentrations of nitrate were used (CitationDarnell and Hiss, 2006) and suggests that growth in the present experiment was limited at external concentrations of 0.5 mM nitrate.
Nitrate reductase is an inducible enzyme and studies have demonstrated increases in activity (CitationNicodemus et al., 2008) and amount (CitationWang et al., 2003) of NR as external nitrate concentrations increase. In the present study, increasing external nitrate concentration increased cumulative nitrate uptake, without a consistent significant increase in NR activity. However, the trend towards increased NR activity at 1.0 vs. 0.5 mM external nitrate at each sampling time suggests an effect of external nitrate concentration on activity. This is supported by the increase in whole-plant reduced nitrogen concentration in V. corymbosum at the higher compared with the lower external nitrate concentration. While a similar response was not seen in V. arboreum, it is likely that the increased whole-plant dry weight of V. arboreum at the higher nitrate concentration resulted in a dilution of the reduced nitrogen concentration in the plant. In fact, total reduced nitrogen content per plant was greater in V. arboreum at the higher compared with the lower external nitrate concentration (319 vs. 265 mg reduced nitrogen per plant, respectively; P ≤ 0.07).
The higher specific iron uptake rate in V. arboreum compared with V. corymbosum was correlated with increased FCR activity throughout the experiment. This agrees with previous work (CitationDarnell and Hiss, 2006) and supports the hypothesis that V. arboreum is more efficient at iron assimilation than is the cultivated species, V. corymbosum. The higher total and active iron concentrations in roots of V. arboreum compared with V. corymbosum give further support to this hypothesis.
Although increased iron concentrations in roots may represent iron precipitates and thus may not be a true representation of tissue iron levels (CitationHell and Stephan, 2003), the increased active iron concentrations found in leaves of V. arboreum, which correlates well with the increased leaf FCR activity, argue strongly for increased assimilation capacity compared with V. corymbosum. Since iron is transported to the leaves via the xylem as a ferric-citrate complex (CitationJeong and Connolly, 2009), a leaf plasma membrane-bound FCR is likely required to reduce the iron before it can be transported from the apoplast into the cell (CitationJeong and Connolly, 2009; CitationSmith and Cheng, 2006). The present work supplies the first evidence that leaf FCR is active in Vaccinium species and appears to play an important role in iron assimilation in leaves. The increased active iron concentration in leaves of V. arboreum suggests that this species may be better able to utilize iron transported to the shoot compared with the cultivated V. corymbosum. What constitutes active iron has not been well defined, yet it is clear that active iron correlates much better with the iron status of leaves than does total iron (CitationSmith and Cheng, 2005; CitationSmith and Cheng, 2006).
The results from the present study indicate that the wild species, V. arboreum, has much greater capacity for both nitrate and iron assimilation than does the cultivated species, V. corymbosum. This is supported by increased nitrate and iron uptake rates, increased NR and FCR activities, and increased concentrations of reduced nitrogen and active iron. The greater assimilation capacity of V. arboreum may be a factor in the ability of this species to grow on more typical agricultural soils, where iron concentrations are low and nitrogen is present primarily as nitrate.
While traits from a wide array of wild Vaccinium species have been incorporated into commercial cultivars of V. corymbosum (CitationLyrene, 1997), none has resulted in significant increases in soil adaptation to date. Furthermore, only recently have fertile hybrids of V. corymbosum × V. arboreum been obtained (P. Lyrene, personal communication). Work is now ongoing examining the use of V. arboreum as a source of genes for wider soil adaptation in V. corymbosum.
LITERATURE CITED
- Anderson , K.A. and Case , T.E. 1999 . Evaluation of plant extraction techniques and effect on commonly used analytical methods of detection . Comm. Soil Sci. Plant Anal. , 30 : 1479 – 1495 .
- Ballington , J.R. 1998 . Performance of own-rooted ‘Premier’ rabbiteye blueberry (Vaccinium ashei Reade) compared to ‘Premier’ grafted on Vaccinium arboreum Marsh (sparkleberry) over four harvest seasons , Edited by: Cline , W.O. and Ballington , J.R. 178 – 181 . Raleigh, N.C : Proc. 8th North American Blueberry Res. Ext. Workers Conf .
- Brown , J.C. and Draper , A.D. 1980 . Differential response of blueberry (Vaccinium) progenies to pH and subsequent use of iron . J. Amer. Soc. Hort. Sci. , 105 : 20 – 24 .
- Camp , W.H. 1945 . The North American blueberries with notes on other groups of Vacciniaceae . Brittonia , 5 : 203 – 275 .
- Chaney , R.L. , Brown , J.C. and Tiffin , L.O. 1972 . Obligatory reduction of ferric chelates in iron uptake by soybeans . Plant Physiol. , 50 : 208 – 213 .
- Darnell , R.L. and Hiss , S.A. 2006 . Uptake and assimilation of nitrate and iron in two Vaccinium species as affected by external nitrate concentration . J. Amer. Soc. Hort. Sci. , 131 : 5 – 10 .
- Gonzalez-Vallejo , E.B. , Susin , S. , Abadia , A. and Abadia , J. 1998 . Changes in sugar beet leaf plasma membrane Fe(III)-chelate reductase activities mediated by Fe-deficiency, assay buffer composition, anaerobiosis, and the presence of flavins . Protoplasma , 205 : 163 – 168 .
- Grusak , M.A. , Kochian , L.V. and Welch , R.M. 1993 . Spatial and temporal development of iron (III) reductase activity in root systems of Pisum sativum challenged with iron-deficiency stress . Amer. J. Bot. , 80 : 300 – 308 .
- Hell , R. and Stephan , U.W. 2003 . Iron uptake, trafficking and homeostasis in plants . Planta , 216 : 541 – 551 .
- Jaworski , E.G. 1971 . Nitrate reductase assay in intact plant tissues . Biochem. Biophys. Res. Comm. , 43 : 1274 – 1279 .
- Jeong , J. and Connolly , E.L. 2009 . Iron uptake mechanisms in plants: Function of the FRO family of ferric reductases . Plant Sci. , 176 : 709 – 714 .
- Korcak , R.F. , Galletta , G.J. and Draper , A.D. 1982 . Response of blueberry seedlings to a range of soil types . J. Amer. Soc. Hort. Sci. , 107 : 1153 – 1160 .
- Lyrene , PM. 1997 . Value of various taxa in breeding tetraploid blueberries in Floirda . Euphytica , 94 : 15 – 22 .
- Mengel , K. 1994 . Iron availability in plant tissues—iron chlorosis on calcareous soils . Plant Soil , 165 : 275 – 283 .
- Merhaut , D.J. and Darnell , R.L. 1995 . Ammonium and nitrate accumulation in containerized southern highbush blueberry plants . HortScience , 30 : 1378 – 1381 .
- Natali , S.M. , Sanudo-Wilhelmy , S.A. and Lerdau , M.T. 2009 . Effects of elevated carbon dioxide and nitrogen fertilization on nitrate reductase activity in sweetgum and loblolly pine trees in two temperate forests . Plant Soil , 314 : 197 – 210 .
- Nicodemus , M.A. , Salifu , K.F. and Jacobs , D.F. 2008 . Nitrate reductase activity and nitrogen compounds in xylem exudate of Juglans nigra seedlings: Relation to nitrogen source and supply . Trees , 22 : 685 – 695 .
- Poonnachit , U. and Darnell , R.L. 2004 . Effect of ammonium and nitrate on ferric chelate reductase and nitrate reductase in Vaccinium species . Ann. Bot. , 93 : 399 – 405 .
- Romheld , V. 2000 . The chlorosis paradox: Fe inactivation as a secondary event in chlorotic leaves of grapeving . J. Plant Nutr. , 23 : 1629 – 1643 .
- Rosen , C.J. , Allan , D.L. and Luby , J.J. 1990 . Nitrogen form and solution pH influence growth and nutrition of two Vaccinium clones . J. Amer. Soc. Hort. Sci. , 115 : 83 – 89 .
- Smith , B.R. and Cheng , L. 2005 . Photoprotective mechanisms of ‘Concord’ grape leaves in relation to iron supply . J. Amer. Soc. Hort. Sci. , 130 : 331 – 340 .
- Smith , B.R. and Cheng , L. 2006 . Fe-EDDHA alleviates chlorosis in ‘Concord’ grapevines grown at high pH . HortScience , 41 : 1498 – 1501 .
- Strik , B.C. and Yarborough , D. 2005 . Blueberry production trends in North America, 1992 to 2003, and predictions for growth . HortTechnology , 15 : 391 – 398 .
- Subasinghe , R. 2007 . Effect of external nitrogen and potassium supply on xylem sap composition of sugarcane . J. Plant Nutr. , 30 : 187 – 201 .
- Thomas , F.M. and Hilker , C. 2000 . Nitrate reduction in leaves and roots of young pedunculate oaks (Quercus robur) growing on different nitrate concentrations . Environ. Expt. Bot. , 43 : 19 – 32 .
- Touraine , B. , Daniel-Vedele , F. and Forde , B.G. 2001 . “ Nitrate uptake and its regulation ” . Edited by: Lea , P.J. and Morot-Gaudry , J.F. 1 – 36 . Berlin : Plant nitrogen. Springer-Verlag .
- Wang , R. , Okamoto , M. , Xing , X. and Crawford , N. 2003 . Microarray analysis of the nitrate response in Arabidopsis roots and shoots reveals over 1,000 rapidly responding genes and new linkages to glucose, trehalose-6-phosphate, iron, and sulfate metabolism . Plant Physiol. , 132 : 556 – 567 .
- Williamson , J.W. and Lyrene , P.M. 1998 . “ Florida's commercial blueberry industry ” . In Bulletin HS742 , Gainesville, FL : Univ. of Florida Coop. Ext. Serv .
- Williamson , J.W. and Miller , P.M. 2009 . Effects of fertilizer rate and form on vegetative growth and yield of southern highbush blueberry in pine bark culture . HortTechnology , 19 : 152 – 157 .