ABSTRACT
Brassica seed meal amendments and anaerobic soil disinfestation (ASD) control a spectrum of soil-borne plant pathogens via a diversity of mechanisms. Transformations in microbial community structure and function in certain instances were determinants of disease control and enhanced plant performance. For instance, in strawberry field soils, increased strawberry yields in response to ASD were attained in a carbon-source input-dependent manner. ASD conducted with rice bran as the carbon input, but not molasses, resulted in significantly greater yields. Effective ASD treatments, but not ineffective treatments, resulted in increased abundance of bacteria in phylum Bacteroidetes in treated soils, specific genera of which are known to provide biological disease control. Brassica seed meal amendment resulted in development of a soil system suppressive towards disease incited by the root pathogen Macrophomina phaseolina. Brassica seed meal treated orchard soil systems exhibit resistance to re-infestation by soil-borne pathogens, including the plant parasitic nematode Pratylenchus penetrans. System resistance is associated with significant and prolonged changes in rhizosphere microbiology and specifically amplification of specific organisms with potential to parasitize P. penetrans.
Introduction
A multiplicity of amendment-based disease control strategies have been evaluated as potential replacements to the use of chemical fumigants for the control of soil-borne plant diseases. In many instances a single input replacement approach, either chemical or biological, has been examined as a strategy to develop feasible alternatives to methyl bromide soil fumigation. The complexity of the causal pathogen systems endemic to strawberry field or orchard soils and their dynamic interactions has resulted in most alternatives lacking the capacity to yield disease control levels comparable to those previously attained through soil fumigation with methyl bromide (Duniway, Citation2002; Leandro et al., Citation2007; McSorely et al., Citation1997). In the study of alternative non-fumigant soil-borne disease control tactics, it is impractical to examine efficacy of the practice toward the broad spectrum of pathogens that may function in any plant production system. Rather, a multitude of studies have been directed toward examination of a practice for control of a specific pathogen operating independently in the field (Elad et al., Citation1986) or laboratory (El Hadrami et al., Citation2011). Such a strategy has tended to yield positive results in controlled environment studies, which subsequently failed to live up to their promise when evaluated across a diversity of field settings.
The failure of proposed non-fumigant strategies in field applications may be attributed, in part, to a lack of understanding regarding functional modes of action. In addition, the diversity of pathogens encountered in agricultural soil systems are not likely to be similarly susceptible to the limited mechanisms of action that may operate in the application of strategies, such as use of compost amendments or biological agents. This may clearly be grasped when comparing the use of compost for control of disease incited by Pythium and Rhizoctonia spp., two elements that function coordinately in many crop production systems, including strawberry and apple (LaMondia, Citation2003; Mazzola, Citation1997, Citation1998; Yuen et al., Citation1991). The specific elimination of one of these two frequently co-residing pathogens commonly results in greater disease severity resulting from the elevated activity of the non-diminished pathogen (Mazzola, Citation1998; Pieczarka and Abawi, Citation1978). Pythium and Rhizoctonia spp. consistently exhibit differential responses to compost amendments with suppression of Pythium reportedly due to a general increase in overall biological activity in soil (Boehm et al., Citation1993; Chen et al., Citation1987), while control of Rhizoctonia is attributed to the function of specific microorganisms resident to the compost amendment (Hoitink et al., Citation1991). Similarly, development of biological agents for soil-borne disease control has most commonly focused on targeting an individual pathogen without attention to the diversity of species or genotypes resident to the soil ecosystem that may incite disease. Such a strategy may fail to recognize variation in pathogen sensitivity to biocontrol mechanisms (Schouten et al., Citation2004), which could contribute to disease control failure (Mazzola et al., Citation1995).
The implementation of disease control practices that employ multiple mechanisms of action may provide a strategy that has potential to yield a greater spectrum of activity towards the pathogen population indigenous to an agricultural soil system. Brassica seed meal (SM) soil amendments or anaerobic soil disinfestation (ASD) are methods that may provide such a range of activity as multiple modes of disease control action have been intimated (Mazzola et al., Citation2007; Momma et al., Citation2013). Disease control attained in response to either method has commonly been attributed to chemical mechanisms of action, including the generation of biologically active volatiles (Angus et al., Citation1994; Hewavitharana et al., Citation2014). However, the role of the indigenous soil microbiology in disease suppression resulting from either practice has also been demonstrated (Momma et al., Citation2010; Weerakoon et al., Citation2012). An understanding of the operative biological agents contributing to disease control and the reproducibility of the phenomenon across production sites will be vital to the predictive use of these treatments as an alternative to soil fumigation for soil-borne disease control in strawberry production systems.
Materials and methods
Brassica seed meal trials
The potential contribution of soil biology to suppression of the strawberry pathogen Macrophomina phaseolina by Brassica juncea SM amendment was assessed in controlled environment trials. The soil used in this study is characterized as Adkins very fine sandy loam with 1.3% organic matter and pH 7.6. Seed meal was incorporated in soil with thorough mixing at a rate of 0.3% (wt/wt), soils were decanted into 3.8-liter plastic pots, and treated soils were incubated in impermeable bags for 24 h to simulate tarping of soil in the field. Treated and non-treated soils were incubated for 8 weeks to eliminate possible herbicidal activity of glucosinolate degradation products (Handiseni et al., Citation2013). Soils were then inoculated with M. phaseolina to attain a final density of 2.5 microsclerotia g–1 soil and planted immediately with bare root strawberry transplants (Fragaria ananassa cv. Camarosa). Plants were grown using a 30 °C/18 °C day/night temperature regime with a 16 h photoperiod. Strawberry plants were removed with roots intact after 10 wk and DNA was extracted from crown tissues using the MoBio PowerPlant Pro DNA isolation kit (Mo Bio Laboratories, Carlsbad, CA, USA) per the manufacturer’s protocol. Crown infection by M. phaseolina was determined by real-time quantitative polymerase chain reaction (PCR) analysis (Mazzola, unpublished).
An orchard field trial was conducted at a replant site near Rock Island, Washington at the Washington State University Sunrise orchard possessing a Pogue fine sandy loam soil type. The site was treated with a Brassica juncea/Sinapis alba (1:1) seed meal (SM) formulation in Sept. 2009 at a rate of 8 t ha–1. Seed meal was incorporated into moist soil using a rotovator to a depth of 15 cm and soils were immediately tarped using a virtually impermeable film 152-μm thick sheeting (Sunbelt Plastics, Monroe, LA, USA), which was removed 1 wk post-SM application. The orchard was planted at 5 weeks post-SM application with apple (Malus domestica borkh. cv. Gala) grafted onto M.9 rootstock. No treatment and 1,3-dichloropropene-chloropicrin (Telone-C17; 282 L ha–1) soil fumigation controls were included. Each treatment was replicated five times in a randomized block design with 10 trees per replicate. The effect of soil treatments on tree growth was assessed by monitoring increase in tree diameter for the duration of each trial. Fruit was harvested from the internal 8 trees from each 10-tree plot, and yield data were recorded in the field on a per tree basis.
Roots and associated rhizosphere soil samples were collected from the trial on 2 Nov. 2011 for examination of treatment effects on overall bacterial and fungal community composition. Samples were obtained from two trees in each plot resulting in a total of 10 samples per soil/apple rootstock treatment. For each sample, DNA was extracted from 7 g of rhizosphere soil using the UltraClean Mega Soil DNA Isolation Kit (Mo Bio Laboratories, Carlsbad, CA, USA). DNA was normalized to a concentration of 20 ng μL–1 and sent to Research and Testing Laboratories, Lubbock, Texas for amplification and pyrosequencing on a 454 Life Sciences GS-FLX instrument (Roche Diagnostics Corporation, Branford, CT).
Anaerobic soil disinfestation trials
Replicated field trials were established during the 2012–13 field season in Watsonville, California (Monterey Bay Academy [MBA] and Plant Sciences International [PSI]), with both sites possessing an Elder sandy loam soil. At the MBA site, treatments included ASD with rice bran (RB; 20 t ha–1), molasses (Mol; 20 t ha–1) and RB (10 t ha–1) + Mol (10 t ha–1), a no treatment control, and a rice bran soil application (20 t ha–1) without additional water. Treatments at the PSI site consisted of the same ASD-RB, ASD-Mol, ASD-RB+Mol, and control treatments as well as a pre-plant methyl bromide-chloropicrin soil fumigation (1:1 mixture 449 kg ha–1) treatment. In both trials, plots were arranged in randomized block design with four replicates per treatment. At bed formation, soil water content was 59.0 g kg–1 soil and 79.5 g kg–1 soil at the MBA and PSI field sites, respectively. Immediately after listing beds, rice bran was applied to the bed top of the assigned plots and rototilled to a depth of 15 cm, beds were reshaped, drip tapes and plastic mulch applied, and the first irrigation began 2 days after rice bran application. At the PSI site, one drip tape was buried at 1.5 cm depth at the center of a 122-cm center-to-center wide bed. At the MBA field, two drip tapes were buried at 1.5 cm depth with a 23 cm spacing at the middle of a 132-cm center-to-center wide bed. Molasses was diluted with water at 1:2 to 1:5 in a water tank prior to application and then 6.5 t ha–1 was applied through drip tapes to the assigned plots. Seven days later, the balance of Mol (3.5 t ha–1) was applied in the same manner. For all ASD treatments, plots were intermittently drip irrigated for 3 weeks from the first irrigation, with a total irrigation amount of 60–75 mm. No water was added to rice bran without water plots until a week prior to transplanting, at which time 15 mm of water was drip irrigated.
Strawberry plants (cv. Albion) were transplanted in Nov. 2012 at both sites. Marketable fruit yield was surveyed at a harvest station in each plot with 40 (PSI) or 89 (MBA) plants per station from Apr. to Aug. 2013 biweekly. There was no known history of soilborne diseases at these sites at the time of trial initiation. A composite soil sample was collected 4 weeks post treatment application (2 Nov. 2012) from each plot at a depth of 0–15 cm with each composite sample comprised of a minimum of 10 sub-samples per plot. DNA was extracted individually from 5 g of each composite soil sample using the PowerMax soil DNA isolation kit (Mo Bio Laboratories, Carlsbad, CA, USA). DNA amplification and pyrosequencing was conducted as noted above.
Results and discussion
Infection of strawberry crowns by M. phaseolina was significantly diminished in soil amended with B. juncea SM relative to the no treatment control (). Although infection was not eliminated by this treatment, the quantity of pathogen DNA detected in strawberry crown tissue was reduced by greater than an order of magnitude relative to the control. The configuration of this trial excluded a potential for the biologically active volatile allyl isothiocyanate (AITC) in the observed disease suppression. In this trial, B. juncea SM was applied 8 weeks prior to infestation of soil with M. phaseolina. Repeated experiments using the rates employed in this study have demonstrated that AITC production and emission is completed within 24–48 h of B. juncea SM application (Mazzola et al., Citation2007; Mazzola and Zhao, Citation2010). Thus, it is probable that the observed suppression of disease incited by M. phaseolina was due to attributes of the soil microbial community that were altered or amplified in response to SM amendment. Densities of both Streptomyces spp. and total fungi were greater than an order of magnitude higher in SM amended than the control soil at planting and at harvest (data not shown). These microbial groups were previously shown to have a significant role in the B. juncea SM-induced biological suppression of disease incited by Rhizoctonia solani AG-5 (Mazzola et al., Citation2007) and Pythium attrantheridium (Weerakoon et al., Citation2012), respectively.
Figure 1. Quantity of Macrophomina phaseolina DNA detected in strawberry crowns by real-time quantitative PCR in non-treated (control) soil and soil that had been amended with Brassica juncea seed meal 8 wk prior to pathogen infestation. Error bars represent one standard deviation of the mean.
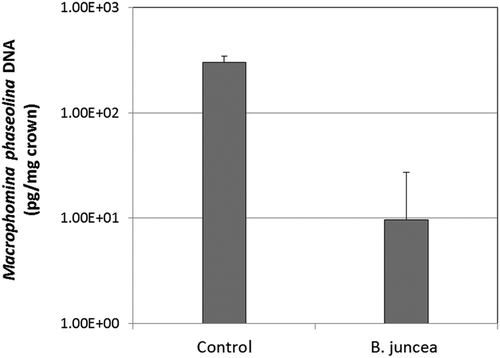
Field application of a Brassicaceae SM formulation for the control of orchard replant disease induced development of a soil microbial community that was resistant to re-infestation of the soil system by root infecting soil-borne pathogens (Mazzola et al., Citation2015). Pre-plant soil fumigation with 1,3-dichloropropene/chloropicrin suppressed Gala/M.9 root densities of P. penetrans () and infection of roots by Pythium spp. (Mazzola et al., Citation2015) relative to the no treatment control during the initial growing season. However, as measured by disease incidence, both pathogens rapidly became re-established in fumigated soil to where there existed no difference in pathogen density between the fumigation and control treatments by the end of the second growing season. In contrast, the B. juncea/S. alba SM treated soil was resistant to re-infestation by either pathogen and root densities of P. penetrans in response to this soil treatment were suppressed for multiple growing seasons (). System resistance to pathogen re-infestation corresponded with greater apple growth and yield in the seed meal amended orchard soil (Mazzola et al., Citation2015). Pratylenchus penetrans functions as a pathogen in strawberry production systems, and can exacerbate black root rot symptoms through its interaction with the cortical root rotting pathogen Rhizoctonia fragariae (LaMondia, Citation2003). Thus, our findings from studies in a perennial orchard environment suggest that the seed meal treatment described here may provide multi-season lesion nematode control in strawberry production systems.
Figure 2. Effect of pre-plant soil treatments on populations of Pratylenchus penetrans recovered from roots of Gala/G11 planted at the Sunrise orchard, Rock Island, WA. Treatments were as follows: Control = no pre-plant treatment; 1,3D-C17 = 1,3-dichloropropene/chloropicrin soil fumigation; BjSa SM = Brassica juncea/Sinapis alba seed meal formulation (1:1). Bars indicate standard deviation of the mean.
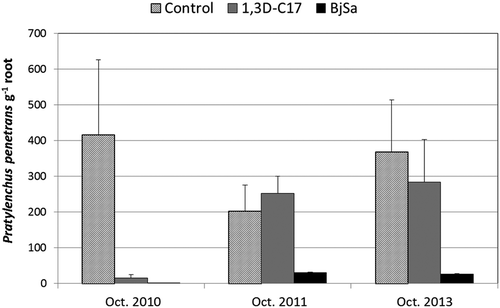
Biological attributes of the Gala/M.9 rhizosphere microbial communities from the control and fumigated soil treatments were indistinguishable when sampled at the end of the second growing season. In contrast, the same communities from the seed meal treatment exhibited clear variance and were significantly different from either the control or fumigation treatments (Mazzola et al., Citation2015). For example, fungi with a known role as nematode parasites, including Arthrobotrys spp., Dactylella oviparasitica, and Plectosphaerella cucumerina, were significantly elevated in or unique to the SM amended soil system. These persistent differences suggest that altered microbial community structure had a significant role in the development of a soil system resistant to pathogen re-infestation.
Carbon input used in the conduct of ASD influenced the strawberry growth and yield response attained in multiple field trials. ASD conducted using rice bran as the carbon input consistently provided yields that were greater than that attained in non-treated soil (). In contrast, strawberry yields achieved in response to ASD with molasses were typically inferior to the ASD-rice bran treatment and on occasion were not significantly different from the control. ASD conducted with a mixture of rice bran and molasses as the carbon input typically resulted in yields that were intermediate between those attained using either carbon source alone.
Table 1. Cumulative strawberry yields (kg ha–1) attained at the MBA and PSI field trials in response to anaerobic soil disinfestation treatments.
Differential yield responses attained with ASD using different carbon sources were associated with relative shifts in the soil microbial community induced by the different treatments. The post-treatment bacterial communities detected in ASD-molasses treated soils exhibited greater similarity to the community detected in non-treated soil at both the MBA and PSI study sites ( and ). The bacterial community detected in ASD-rice bran, ASD-rice bran+molasses, or methyl bromide/chloropicrin treated soils clearly differed from the non-treated control. With the exception of the fumigated soil, the capacity of an ASD treatment to enhance strawberry growth and yield was related to differential treatment effects on abundance of certain bacterial groups. Specifically, carbon source-dependent efficacy of ASD was associated with increased abundance of bacteria in phylum Bacteroidetes. In control and ASD-molasses treated soil this group represented only 8–12% of the total bacterial population in strawberry field soils. Among individual samples, Bacteroidetes represented 15–20% of the bacterial community in ASD-rice bran+molasses treated soil and 25–35% of the populations detected in soils treated with ASD-rice bran.
Figure 3. Effect of preplant orchard soil treatments on bacterial community composition detected in strawberry field soils (MBA site) at the end of the harvest season as assessed by nonmetric multidimensional scaling of phylum level taxonomic data using the Bray–Curtis distance metric. Treatments:




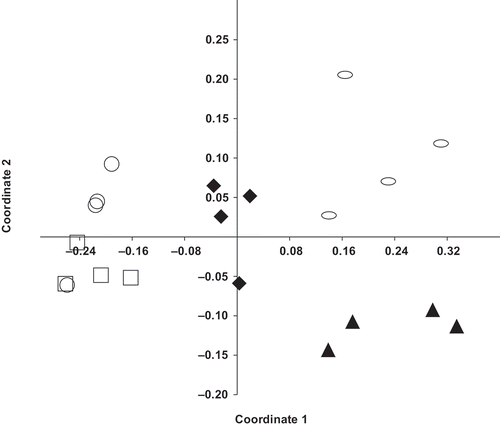
Figure 4. Effect of preplant orchard soil treatments on bacterial community composition detected in strawberry field soils (PSI site) at the end of the harvest season as assessed by nonmetric multidimensional scaling of phylum level taxonomic data using the Bray–Curtis distance metric. Treatments:



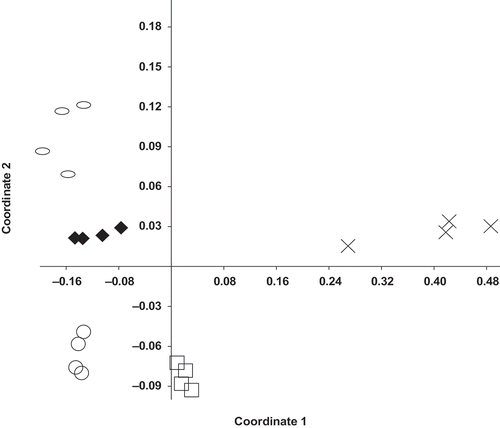
Kyselková et al. (Citation2009) found that members in the taxa Pseudomonas and Bacteroidetes were higher in soil suppressive to tobacco black rot caused by Thielaviopsis basicola. The population of Bacteroidetes recovered from ASD treated strawberry field soils in this study possessed a number of genera of interest from a disease control perspective, including Chryseobacterium, Chitinophaga, Cytophaga, Flavobacterium, and Sphingobacterium. Isolates of Chryseobacterium from wheat rhizosphere soils were shown to provide control of Rhizoctonia bare patch of wheat caused by Rhizoctonia solani AG-8 (Yin et al., Citation2013). Chryseobacterium accounted for only 0.09% and 0.06% of bacterial sequences detected in non-treated and ASD-molasses treated soils, respectively. In comparison, this bacterial group represented 3.72% and 1.35% of sequences from ASD-rice bran and rice bran alone (no additional water) soil treatments (). There are reports of Flavobacterium providing control of various plant pathogenic fungi, including Verticillium dahliae. Likewise, several chintinolytic bacteria have demonstrated biological control activity towards soil-borne plant pathogens (Singh et al., Citation1999). In the current study, the chitinolytic gliding bacterium Chitinophaga exhibited trends similar to Chryseobacterium, representing less than 1% of sequences detected in non-treated or ASD-molasses treated soils and 3–5% of identified sequences from ASD-rice bran or rice bran treated soils.
Table 2. Relative abundancez of select bacterial taxa that differed significantly among treatments from strawberry field soils.
The preponderance of studies concerning the implementation of Brassica seed meal soil amendments or anaerobic soil disinfestation have been formulated with the notion that chemical or physical mechanisms of action are dominant determinants of the disease control outcome (Angus et al., Citation1994; Blok et al., Citation2000; Price et al., Citation2005). Although chemical mechanisms of action may contribute to pathogen suppression in response to either of these control strategies, an emerging body of evidence, including the current studies, either suggests or explicitly demonstrates the role of biological factors participating in the process. The mechanism(s) functional in pathogen suppression may differ with the target pathogen and may also differ in a temporal manner (Mazzola et al., Citation2007; Momma et al., Citation2013; Weerakoon et al., Citation2012). An understanding of these processes is integral to the effective utilization of ASD or Brassica SM amendments, alone or in combination, across the diversity of individual and complexes of pathogens encountered in strawberry and apple production systems. For example, sequence of application may inadvertently result in altered action or elimination of biological entities contributing to disease control. Our understanding of disease control processes attained through the use of biologically active inputs, such as ASD or Brassica SM amendments, will enable effective management of differing modes of action yielding an enhanced spectrum of pathogen control. Such a capacity is necessary to ensure the commercially required levels of soil-borne disease control previously achieved through pre-plant soil fumigation.
Funding
This work was funded in part through grants from the USDA-NIFA Organic Agriculture Research and Extension Initiative Grants Program, the USDA-NIFA Methyl Bromide Transitions Grants Program, and the California Strawberry Commission.
Additional information
Funding
Literature cited
- Angus, J.F., P.A. Gardner, J.A. Kirkegaard, and J.M. Desmarchelier. 1994. Biofumigation: Isothiocyanates released from Brassica roots inhibit growth of the take-all fungus. Plant Soil 162:107–112.
- Blok, W.J., J.G. Lamers, A.J. Termorshuizen, and G.J. Bollen. 2000. Control of soilborne plant pathogens by incorporating fresh organic amendments followed by tarping. Phytopathology 90:253–259.
- Boehm, M., L.V. Madden, and H.A.J. Hoitink. 1993. Effect of organic matter decomposition level on bacterial species diversity and composition in relationship to Pythium damping-off severity. Appl. Environ. Microbiol. 59:4171–4179.
- Chen, W.H., H.A.J. Hoitink, and A.F. Schmitthenner. 1987. Factors affecting suppression of Pythium damping-off in container media amended with composts. Phytopathology 77:755–760.
- Duniway, J.M. 2002. Status of chemical alternatives to methyl bromide for pre-plant fumigation of soil. Phytopathology 92:1337–1343.
- Elad, Y., Y. Zvieli, and I. Chet. 1986. Biological control of Macrophomina phaseolina (Tassi) Goid by Trichoderma harzianum. Crop Prot. 5:288–292.
- El Hadrami, A., L.R. Adam, and F. Daayf. 2011. Biocontrol treatments confer protection against Verticillium dahliae infection of potato by inducing antimicrobial metabolites. Mol. Plant-Microbe Interact. 24:328–335.
- Handiseni, M., J. Brown, R. Zemetra, and M. Mazzola. 2013. Effect of Brassicaceae seed meals with different glucosinolate profiles on Rhizoctonia root rot of wheat. Crop Prot. 48:1–5.
- Hewavitharana, S.S., D. Ruddell, and M. Mazzola. 2014. Carbon source-dependent antifungal and nematicidal volatiles derived during anaerobic soil disinfestation. Eur. J. Plant Pathol. 140:39–52.
- Hoitink, H.A.J., Y. Inbar, and M.J. Boehm. 1991. Status of compost-amended potting mixes naturally suppressive to soilborne diseases of floricultural crops. Plant Dis. 75:869–873.
- Kyselková, M., J. Kopecký, M. Frapolli, G. Défago, M. Ságová-Marecková, G.L. Grundmann, and Y. Moënne-Loccoz. 2009. Comparison of rhizobacterial community composition in soil suppressive or conducive to tobacco black root rot disease. ISME J. 3:1127–1138.
- LaMondia, J.A. 2003. Interaction of Pratylenchus penetrans and Rhizoctonia fragariae in strawberry black root rot. J. Nematol. 35:17–22.
- Leandro, L.F.S., L.M. Ferguson, F.J. Louws, and G.E. Fernandez. 2007. Strawberry growth and productivity in fumigated compared to compost-amended production systems. HortScience 42:227–231.
- Mazzola, M. 1997. Identification and pathogenicity of Rhizoctonia spp. isolated from apple roots and orchard soil. Phytopathology 87:582–587.
- Mazzola, M. 1998. Elucidation of the microbial complex having a causal role in the development of apple replant disease in Washington. Phytopathology 88:930–938.
- Mazzola, M., J. Brown, A. Izzo, and M.F. Cohen. 2007. Mechanism of action and efficacy of seed meal-induced suppression of pathogens inciting apple replant disease differ in a Brassicaceae species and time-dependent manner. Phytopathology 97:454–460.
- Mazzola, M., D.K. Fujimoto, L.S. Thomashow, and R.J. Cook. 1995. Variation in sensitivity of Gaeumannomyces graminis to antibiotics produced by fluorescent Pseudomonas spp. and effect on biological control of take-all of wheat. Appl. Environ. Microbiol. 61:L2554–2559.
- Mazzola, M., S. Hewavitharana, and S.L. Strauss. 2015. Brassica seed meal soil amendments transform the rhizosphere microbiome and improve apple production though resistance to pathogen re-infestation. Phytopathology 105:460–469.
- Mazzola, M., and X. Zhao. 2010. Brassica juncea seed meal particle size influences chemistry but not soil biology-based suppression of individual agents inciting apple replant disease. Plant Soil 337:313–324.
- McSorley, R., P.A. Stansly, J.W. Noling, T.A. Obreza, and J.M. Conner. 1997. Impact of organic soil amendments and fumigation on plant parasitic nematodes in a southwest Florida vegetable field. Nematropica 27:181–189.
- Momma, N., Y. Kobara, S. Uematsu, N. Kita, and A. Shinmura. 2013. Development of biological soil disinfestations in Japan. Appl. Microbiol. Biotech. 97:3801–3809.
- Momma, N., M. Momma, and Y. Kobara. 2010. Biological soil disinfestation using ethanol: Effect on Fusarium oxysporum f. sp. lycopersici and soil microorganisms. J. Gen. Plant Pathol. 76:336–344.
- Pieczarka, D.J., and G.S. Abawi. 1978. Effects of interaction between Fusarium, Pythium, and Rhizoctonia on severity of bean root rot. Phytopathology 68:403–408.
- Price, A.J., C.S. Charron, A.M. Saxton, and C.E. Sams. 2005. Ally isothiocyanates and carbon dioxide produced during degradation of Brassica juncea tissue in different soil conditions. HortScience 40:1734–1739.
- Schouten, A., G. van den Berg, V. Edel-Hermann, C. Steinberg, N. Gautheron, C. Alabouvette, C.H. (Ric) de Vos, P. Lemanceau, and J.M. Raaijmakers. 2004. Defense responses of Fusarium oxysporum to 2, 4-diacetylphloroglucinol, a broad-spectrum antibiotic produced by Pseudomonas fluorescens. Mol. Plant-Microbe Interact. 17:1201–1211.
- Singh, P.P., Y.C. Shin, C.S. Park, and Y.R. Chung. 1999. Biological control of Fusarium wilt of cucumber by chitinolytic bacteria. Phytopathology 89:92–99.
- Weerakoon, D.M.N., C.L. Reardon, T.C. Paulitz, A.D. Izzo, and M. Mazzola. 2012. Long-term suppression of Pythium abappressorium induced by Brassica juncea seed meal amendment is biologically mediated. Soil Biol. Biochem. 51:44–52.
- Yin, C., S.H. Hulbert, K.L. Schroeder, O. Mavrodi, D. Mavrodi, A. Dhingra, W.F. Schillinger, and T.C. Paulitz. 2013. The role of bacterial communities in the natural suppression of Rhizoctonia bare patch of wheat Triticum aestivum L. Appl. Environ. Microbiol. 79:7428–7438.
- Yuen, G.Y., M.N. Schroth, A.R. Weinhold, and J.G. Hancock. 1991. Effects of soil fumigation with methyl bromide and chloropicrin on root health and yield of strawberry. Plant Dis. 75:416–420.