ABSTRACT
Composts can have beneficial effects on strawberry production and these benefits can be dependent on the type of compost used. Four commercial composts were evaluated: cow manure, spent mushroom, yard trimmings, and vermicompost. The nutrient composition, abundance of fungi and bacteria, and microbial activity were determined. Five field trials assessed effects on plant growth, root development, soil microbial activity, nutrient availability, and yield during one growing season. Manure and mushroom compost significantly increased soil electrical conductivity levels to 9.9 ± 1.7 dS/m and 7.3 ± 0.8 dS/m, respectively. Manure, yard trimmings, and mushroom composts shifted soil pH toward optimal levels for up to 7 months. Mushroom compost had the greatest effect on soil nitrate, which was up to 32 mg/kg of soil higher than non-amended soil. Significant effects on yield were more likely to occur where environmental conditions and management practices were less than optimal or compost was not routinely added. Several factors important to plant production were significantly affected by compost: soil salinity, plant establishment, soil nitrate, production curves, soil microbial activity, and soil pH.
Introduction
Managing soil productivity is important to California strawberry growers because changing fumigation practices have increased the incidence and severity of many soilborne diseases (Koike et al., Citation2012). Soil fumigation with methyl bromide and chloropicrin has been standard practice for nearly 60 years, providing excellent control of soilborne diseases, as well as weed control and risk mitigation (Wilhelm and Sagen, Citation1974). International agreements associated with the Montréal Protocol initiated a phase out of methyl bromide (White, Citation2013), and regulations in California are limiting the use of other fumigants, such as chloropicrin. These changes have forced growers to adopt new practices, which have proven less effective in suppressing soilborne pathogens (Koike et al., Citation2009, Citation2012). Growers are also losing synergistic effects of combining methyl bromide and chloropicrin, which can increase yields by up to 25% compared to non-fumigated soils, even in the absence of major pathogens (Yuen et al., Citation1991). Identifying practices effective in narrowing the yield gap between production in fumigated and non-fumigated soils, and generating productive soils will be critical to survival of the California strawberry industry.
Pre-plant incorporation of amendments, such as compost, is a common practice in California strawberry production that provides both agronomic and environmental benefits. Compost contains micro- and macronutrients, enhances water-holding capacity, and improves soil structure. Compost also has been long identified as a contributor to disease suppression based on qualitative and quantitative changes it induces in the soil microbial community (Noble and Coventry, Citation2005). Furthermore, compost provides a productive use for the approximately one billion metric tons (mt) of organic and inorganic agricultural wastes generated yearly in the United States (Edwards and Someshwar, Citation2000). Thus, the use of compost in commercial plant production has the dual benefit of advancing agricultural production practices while managing compostable wastes.
California’s Central Coast strawberry growers typically apply compost every 1–2 years at 4.4–6.6 mt/ha in conventional production and 15.5–24.4 mt/ha in organic production. Growers have a choice of commercially available compost materials, yet little is known about differences between composts and the extent to which they influence soil health and plant productivity. Feedstocks used to make compost can vary widely and the resulting composts differ in physical, chemical, and biological properties. It would be useful for growers to know the extent to which these differences affect soil characteristics and plant growth. Accordingly, this study was undertaken to evaluate four composts that are readily available to California strawberry growers: manure compost, spent mushroom compost, vermicompost and yard trimmings compost. The objective was to determine whether compost type differentially affects biotic and abiotic soil characteristics and berry production, including total yield, seasonal production curves, plant growth rate, root development, soil nutrient levels, and soil microbial activity.
Materials and methods
Compost materials
Compost was obtained from each of four commercial producers () throughout the research project and stored at room temperature (24 ± 2 °C) for up to 90 days prior to use. Each collection was a composite sample from finished piles available to growers for purchase. The California Composting Council and Cal Recycle have established regulations that require materials to reach 55 °C (131 °F) or higher for 15 days or longer with a minimum of five turnings of the windrow during this time (Cal Recycle, Citation2010). Z-Best and Central Coast Compost are state permitted composting facilities that are visited routinely by the local enforcement authority to ensure compliance with these regulations. TerraVesco and Monterey Mushroom are commercial facilities but are not state permitted.
Table 1. Compost feedstock description and commercial source.
Experimental design and amendment rates
In 2013, five field trials were established in northern California to evaluate four commercial composts: mushroom compost, cow manure compost, vermicompost, and yard trimmings (). The five experimental sites included two fields in the Central Valley (CV 1, CV 2) (Sacramento County) where conventional practices were used, and three fields on the Central Coast (Monterey and Santa Cruz Counties), which consisted of two certified organic fields (CC 1, CC 2) and one fumigated field (CC-F) (a 50:50 blend of methyl bromide and chloropicrin at 420 kg/hectare). Soil types at each site were as follows: CV 1, San Joaquin silt loam; CV 2, San Joaquin-Xerarents complex; CC 1, Salinas Clay loam; CC 2, Watsonville loam; and CC-F, Baywood loamy sand. All composts, except vermicompost, were broadcast applied to individual beds at 67.2 mt/ha or 819 kg/100 linear bed meters and incorporated to a depth of approximately 30 cm by hand or rototiller. Vermicompost was applied at approximately 11.2 mt/ha by adding 236 ml per planting hole and blending with surrounding field soil in situ using a trowel. Due to the high cost, vermicompost is not broadcasted. Each trial included four replications of five treatments (four composts and a non-amended control) in a randomized complete block design. A replication (= plot) was represented by 5.5 to 9.1 linear bed meters, depending on location. All trials included at least one buffer row on both sides and a minimum of 1 m buffer at each end.
Table 2. Description and experimental design of field trials.
Soil sampling
Soil samples were collected at planting in November, early harvest in March, and end of season, June or October. Soil cores (3.5 cm diameter × 11 cm long) were taken 15 ± 3 cm from the crown, toward the drip tape situated in the center of the bed. Four to six samples per plot were randomly selected for soil cores. Soil cores were bulked as a single composite sample per plot, transported on ice and subsequently stored at 4 °C. These samples were used for physiochemical analyses (nutrient, pH, and electrical conductivity) conducted at Perry Labs (Watsonville, CA, USA), and microbial activity using fluorescein diacetate hydrolysis, as described below. At Perry Labs, nitrate analysis was performed on a dried sample, extracted with sodium acetate:acetic acid and analyzed on a Timberline Ammonia Analyzer (Timberline Instruments, Boulder, CO, USA). Soil pH was measured from a saturated paste. The extract from the saturated pasted was used to measure electrical conductivity.
Microbial activity
Soil microbial activity was measured by fluorescein diacetate (FDA) hydrolysis. For FDA hydrolysis, soil samples described above were analyzed within 48 h of collection. Before field trials were initiated, the effect of 4 °C storage duration for 30 min, 24 h, and 48 h on microbial activity of pure composts was shown not be significant (data not included).
For each field site, one standard curve was generated at the beginning of the trial to estimate the influence of the native field soil on the FDA hydrolysis result. To do so, 45 grams of soil was autoclaved twice over two consecutive days at 120 °C for 20 min. For each sample, eighteen 2-gram subsamples were dispensed into 50-ml flasks. Serial amounts of FDA (0, 10, 25, 50, 75, 100, 150, 200, and 300 μl) were added to glass tubes containing 5 ml of 60-mM potassium phosphate and prehydrolyzed by boiling in water for 60 min. Prehydrolyzed FDA was added to the flasks of autoclaved soil, placed on a rotary shaker for 20 min and the remainder of the protocol was performed, as described below, for measuring FDA hydrolysis.
Two replicate 2-gram samples from each plot were ground with a glass rod following the addition of 15 ml of 60-mM potassium phosphate. To each flask, 200 μl of FDA were added. FDA was prepared fresh for each use, dissolved in acetone (2 mg/ml), and stored on ice for the duration of the protocol. Flasks were covered with parafilm and incubated at room temperature (24 ± 2 °C) on a rotary shaker at 90 rpm for 20 min. To stop the reaction, 15 ml of chloroform/methanol (2:1 v/v) were added to each flask. To measure the effect of soil on fluorescence, ‘blank controls’ were established by following the same protocol except chloroform/methanol was added immediately following addition of FDA. Two samples were taken from each flask and spun at 14000 rpm for 2 min in a centrifuge. The supernatant was decanted and used for absorbance measurements. Hydrolysis of FDA was quantified based on absorbance at 490 nm, using a Genesys 10 vis spectrophotometer (Thermo Electron Corporation, Waltham, MA, USA). Microbial activity was expressed per gram of dry soil.
Plant growth rate
Plant canopy diameter was determined as the longest distance from leaf tip to leaf tip, across the center of the crown. Measurements were taken from two plants randomly selected from each side of the bed, for a total of four measurements per plot for which the mean was used for analysis. This process was repeated three to four times during the season, starting in November and ending in June (data not included). Mean growth rate was determined by subtracting the canopy of the earlier date from the later date, dividing by the number of days between sampling, and expressed as cm per 30 days.
Root development
Root development was evaluated by taking one core (3.5 cm diameter × 11 cm long) per plot, 3.8 cm from the strawberry crown in the direction of the drip line so as to capture new roots, closest to the water and fertigation source. Cores were taken from areas under plastic and absent of weeds. Each core was dried, weighed, and either processed immediately or stored at 4 °C in zip lock bags. After rehydrating the core, samples were spread on a tray, flooded with water, and all visible roots were removed. Next, the sample was passed through soil sieve #20 (850 μm) and any roots retained on the sieve were collected. The filtrate was also checked for roots, and for consistency each sample was examined for 2 min.
Roots collected from each core were spread on a transparent tray with water and separated using tweezers to minimize overlap. The tray was scanned using a flatbed Epson Expression 1640XL scanner (Epson America, Inc., Long Beach, CA) adapted with an Epson A3 transparency unit. Root length density (RLD) was quantified using the software WinRHIZO (Regent Instrument Inc., Quebec, Canada). For each batch of samples processed, a scanned image of an empty tray with water was used to measure background noise caused by marks in the tray. All data were expressed as root length density, i.e., the cumulative root length per gram of dry soil.
Strawberry cultivars and cultivation
Two public strawberry cultivars, Albion a day neutral cultivar and Chandler a short day, June bearing cultivar, were used in the study. Dormant bare root strawberry plants (Fragaria × ananassa) of each cultivar were transplanted between 18 Oct. and 16 Nov. 2013. Plants originated from a high elevation nursery (Lassen Canyon Nursery Inc., Redding, CA, USA) and underwent supplemental chill for the recommended interval of 10–18 days. Plants were grown in raised beds, covered with black plastic measuring 122 cm center-to-center with two planted rows, with one to two drip lines, except field site CC 2, which consisted of one row of berries and 102 cm center-to-center beds. Strawberries were harvested by hand 1–3 times per week for the duration of the growing season and marketable yield was recorded. Management of pests, weeds, irrigation, and nutrients was performed by each grower as part of his or her standard practice.
Total fungal and bacterial counts
One gram of compost was added to 10 ml of 1× basic salt solution (1 g KH2PO4/L and 5 g NaCl/L; de Boer et al., Citation1998) and placed on a rotary shaker for 120 min (150 revolutions/min). Serial dilutions were made and an automated spiral plater (Eddy Jet, IUL Instruments, Barcelona, Spain) was used to distribute 50 μl on a plate of 1/10 strength tryptic soy agar amended with one ml natacid solution (50 mg natacid/ml methanol) to control for fungal growth; 50 μl were also placed on a plate of 1/10 diluted potato dextrose agar amended with antibiotics (chlorotetracycline, 100 mg/L; streptomycin sulfate, 100 mg/L; and neomycin sulfate, 100 mg/L) to control for bacterial growth. Plates were incubated at 20 °C for 72 h. Colonies were enumerated and converted to CFU/ml using an automatic colony counter (Flash & Go, IUL Instruments, Barcelona, Spain).
Statistical analysis
The Shapiro-Wilk test was used to determine whether the data were normally distributed. Analysis of variance (ANOVA) was used to test for significant treatment effects, and where significant F values were obtained, the significance of mean differences was assessed using Tukey post-hoc multiple comparison. All tests were performed using R statistical computing software (R Core Team, Citation2013).
Results
Pure compost
Mean nitrate-N levels of pure compost were collected from 2–5 independent batches taken over 6 months. Vermicompost had the highest mean nitrate-N level, 502 mg/kg, and yard trimmings had the lowest, 6.6 mg/kg. Yard trimmings and vermicompost had the highest C:N ratio (17:1), followed by mushroom compost (14:1) and manure compost (12:1) ().
Table 3. Carbon and nitrogen composition of each compost.
Fungal abundance from lowest to highest was: manure compost, yard trimmings, vermicompost, and mushroom compost (). Bacterial abundance followed a similar ranking except manure compost had higher abundance than yard trimmings and vermicompost. Mushroom compost consistently had the highest bacterial and fungal abundance. Rankings were the same when measured as compost only or in potting soil amended at a rate of 22.4 mt/ha. Microbial activity was highest in mushroom compost and vermicompost. Both were significantly higher than yard trimmings, which was significantly higher than manure compost.
Table 4. Summary of fungal and bacterial abundance and microbial activity of composts.
Soil nitrate-N
The effect on soil nitrogen levels varied significantly by compost (). In addition to nitrate-N originating from the compost over the duration of the trial, additional nitrate may become available from the compost. At 67.2 mt/ha application rate (and 11.2 mt/ha for vermicompost), total nitrogen added to each plot ranged from 269 kg/ha (vermicompost) to 1283 kg/ha (yard trimmings).
Figure 1. Mean soil nitrate-N at 2 weeks post compost incorporation (A) and 7 months post incorporation (B) at five field locations. Vertical bars represent the standard error of the mean. * indicates mean is significantly different from the non-amended control based on Tukey HSD post-hoc analysis at P ≤ 0.05.
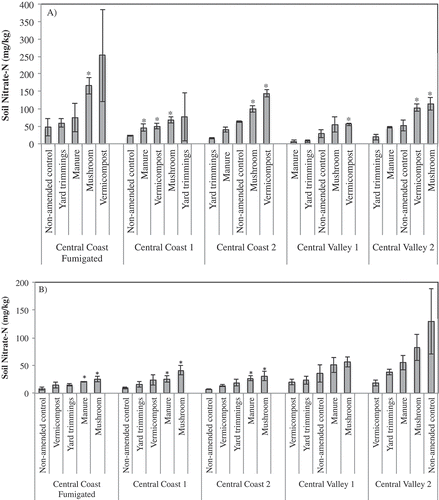
Further, 2 weeks after incorporation, mushroom compost and vermicompost significantly increased soil nitrate levels compared to non-amended field soil in four of five fields. Soil nitrate levels in plots amended with mushroom compost and vermicompost ranged from 55–166 mg/kg and 50–252 mg/kg, respectively. Mean soil nitrate levels from manure compost and yard trimmings were greater than the non-amended control in two fields and lower than the control in three fields. By June, the highest nitrate levels in four of the five plots were those amended with mushroom compost, ranging from 25–82 mg/kg across sites, with a mean of 47 mg/kg. By June, at the three coastal fields, the lowest mean soil nitrate was in non-amended plots, with levels ranging from 7.1–8.8 mg/kg.
Soil electrical conductivity
At 2 weeks post-incorporation, electrical conductivity (EC) had the following range: non-amended control, 1.3–3.4 dS/m; vermicompost, 1.7–3.8 dS/m; yard trimmings compost, 1.7–3.4 dS/m; manure compost, 3.1–9.9 dS/m; and mushroom compost, 4.4–7.3 dS/m (). The type of salt was not analyzed. In most cases, vermicompost and yard trimmings compost had the least impact on soil EC at planting. Soil salinity in the three coastal field trial locations was 2.3 dS/M or less 7 months post-incorporation. However, at both Central Valley field trial locations 7 months post-incorporation, plots amended with mushroom and manure compost maintained an EC level of 3.7–4.6 dS/M compared to 1.2–1.5 dS/M in the non-amended control plot. Comparing fields, Central Coast 1 and Central Valley 1, the change in soil electrical conductivity over several months post-incorporation shows two scenarios, one in which levels decrease over time (CC 1) and two in which little change is exhibited (CV 1) (). Visible damage and plant death were observed in plots amended with manure and mushroom compost, especially in the Central Valley locations ().
Table 5. Electrical conductivity at two sampling intervals, approximately 2 weeks, and 7 months post incorporation.
Figure 2. Mean electrical conductivity in compost-amended soil at time of compost incorporation (Oct. 2013) and 5 months later at Central Coast 1 (A) and 7 months later at Central Valley 1 (B). Vertical bars represent the standard error of the mean. Means followed by the same letter are not significantly different based on Tukey HSD post-hoc analysis at P ≤ 0.05.
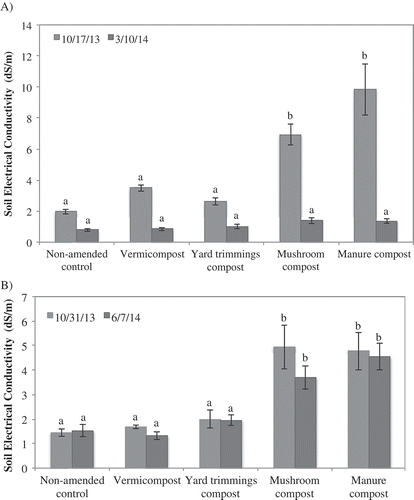
Soil pH
Yard trimmings, manure, and mushroom compost significantly buffered soil pH in most fields, moving soil pH closer to optimal levels (). At 8 months after composts were incorporated in soil with an acidic pH, yard trimmings, manure, and mushroom compost raised the pH from 5.8 to 7.2–7.4 (CV 2), 6.5 to 7.0–7.3 (CV 1), and 6.6 to 7.2–7.6 (CC 2), respectively. In neutral soil, all composts raised the pH from 7.0 to 7.3–7.6 (CC-F). In plots amended with vermicompost, pH remained close to that of non-amended soil, probably because vermicompost was applied only to the planting hole and soil sampling captured non-amended soil outside the planting hole. Finally, where non-amended soil was basic (CC 1), compost lowered the pH from 8.0 to 7.7–7.9 by the end of the season. For all locations, effect of compost on soil pH was maintained throughout the growing season.
Microbial activity
At the time of planting, yard trimmings and mushroom compost consistently had the greatest effect on microbial activity, followed by vermicompost and manure compost (). Mean microbial activity was significantly increased by yard trimmings and mushroom compost in all field trials. Mean microbial activity in soil amended with vermicompost and manure compost was higher than non-amended soil in all fields except CC-F, and was only significantly greater in one field, CV 1. Over time, microbial activity generally decreased from the initial spike after compost incorporation. Nevertheless, compared to the non-amended plots, mean microbial activity in compost-amended plots remained elevated in several fields 7–10 months post-incorporation and was significantly higher than the non-amended plot in at least one case in four of the five fields. Yard trimming-amended plots had the highest mean soil microbial activity in three of five fields, and second highest in the other two, 7 months post-incorporation.
Figure 5. Mean soil microbial activity at five field trial locations measured at planting 2–4 weeks post-incorporation of compost (A) and 7 months post-incorporation (B). Vertical bars represent the standard error of the mean. Means followed by the same letter are not significantly different based on Tukey HSD post-hoc analysis at P ≤ 0.05.
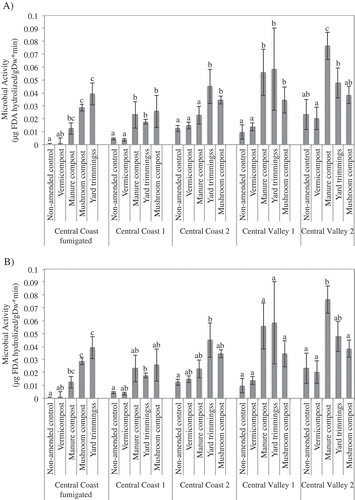
Plant growth rate
February–April is the rapid canopy growth period and April–July is early to peak harvest. At field sites CC 1 and CC 2, the effect of compost on growth rate was similar for both ‘Albion’ and ‘Chandler’ (). However, ‘Chandler’ grew faster in non-amended soil than in plots with compost, whereas non-amended plots planted with ‘Albion’ were ranked intermediate. For both cultivars, vermicompost ranked first or second for highest growth November through January, except for Chandler at CC 1. Plots amended with yard trimmings and manure compost were slower growing from November through January, but were among the faster growing plant canopies at most locations from February through April. Plant growth rate in plots amended with mushroom compost ranged from first to fourth ranking from November through January, and ranged from second to fourth ranking from February through April.
Table 6. Mean growth rate of Albion and Chandler in soils amended with compost.
Root development
An analysis of variance showed significant effects of cultivar (P = 0.0002) and location (P ≤ 0.001) on total root length. A post-hoc Tukey HSD test detected root length density (RLD) of Albion to be significantly greater at CC 1 than CC 2 and CC-F (Tukey HSD, P ≤ 0.05). For both cultivars, CC 2 had the lowest RLD, followed by CC-F with intermediate, and CC-1 with the highest RLD (). There were significant differences in RLD between ‘Albion’ and ‘Chandler’, with ‘Albion’ tending to produce more roots than ‘Chandler’ in all treatments. Within a single cultivar at a single location, no significant differences were detected between treatments. From 23 Apr. and 7 May, ‘Chandler’ RLD increased in all treatments, except in mushroom compost, but only vermicompost-amended soil manifested a significant increase in root length density (P = 0.015) over the 2 weeks. Plants in mushroom compost-amended plots had the greatest RLD when samples were taken on 23 Apr. (16 cm/g soil), and the mean decreased to 12 cm/g soil by 7 May. The ranking of mean RLD of each treatment followed a similar trend for both cultivars. At CC 1, excluding vermicompost, mean RLD for both cultivars, from lowest to highest, was the non-amended control, mushroom compost, yard trimmings, and manure compost. For vermicompost, ‘Albion’ had the lowest and ‘Chandler’ had the highest RLD. At CC 2, mean root length for both cultivars, from lowest to highest, was non-amended control, manure compost, vermicompost, yard trimmings, and mushroom compost.
Marketable yield
Analysis of variance detected highly significant (P ≤ 0.001) main effects for treatment, field, cultivar, and a significant treatment*field interaction. Given the significant interaction, data were analyzed separately by field. Within a field, the effect of treatment on yield was significant for ‘Albion’ at CC 1 (P = 0.021) and for ‘Chandler’ at CC 1 (P = 0.001), CV 1 (P = 0.036), and CV 2 (P = 0.015).
For ‘Chandler’, among treatments, vermicompost produced the highest mean yield in three of the four fields, though yields were not significantly different than the non-amended control (). In CC 2, plots amended with manure compost produced significantly less fruit than the non-amended control and vermicompost amended plots. In the Central Valley locations, vermicompost was more productive than manure compost (CV 1, P = 0.06) and yard trimmings (CV 2, P = 0.10), though not significantly higher. Plots amended with yard trimmings compost were consistently low yielding compared to other treatments for ‘Chandler’, but yields were intermediate to high with ‘Albion’ ().
Figure 7. Total marketable yield at four locations for ‘Chandler’ (A) and three locations for ‘Albion’ (B). Vertical bars represent the standard error of the mean. Means followed by the same letter are not significantly different based on Tukey HSD post-hoc analysis at P ≤ 0.05.
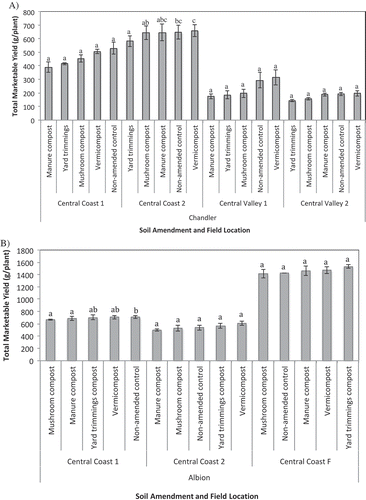
For ‘Albion’, the highest cumulative marketable yield was recorded at CC-F, followed by CC 1 and CC 2. Plots amended with mushroom compost produced lower yields in all field trials, whereas vermicompost-amended plots produced higher yields in all field trials as compared to other compost treatments. In no fields did compost amendments result in significantly higher cumulative yields than the non-amended control.
Seasonal production curves (cumulative weight of fruit harvested on a weekly basis) show treatment effects beginning 16 May (week 7) for ‘Chandler’ and 14 August (week 19) for ‘Albion’ ( and ). Even though final cumulative yield was not significantly different, these results show that higher mean yield is achieved with vermicompost amendments in the second half of the production season for both ‘Chandler’ (CC 1) and ‘Albion’ (CC 2).
Discussion
Soil fumigant phase-out is forcing growers to adopt alternative practices for management of soilborne diseases. This should include closer attention to the physical, chemical, and biological properties of soil, which can have a significant effect on the activity of soilborne pathogens and productivity of plants over the long growing season. Strawberry growers commonly incorporate compost prior to planting but little is known about how differing sources of compost may affect strawberry production. This study was undertaken to evaluate four readily available composts in order to characterize their effects on soil quality and fertility, strawberry growth and yield, and microbial activity and disease suppression.
Soil quality and fertility
Composts differ in salt content and consequently may affect the risk of salt damage and long-term soil quality. Ongoing drought in California, especially the reduction in winter rains critical to salt leaching, has heightened the risk of salt accumulation. Strawberries are salt-sensitive with an optimal EC level of 1–2 dS/M (IPM for Strawberries, Citation2008). Manure and mushroom composts significantly increased EC above optimal levels. Visible salt damage and poor plant establishment emphasized the potential for compost to salinize soil in the absence of proper leaching. Furthermore, high EC in the Central Valley, 7 months post-incorporation, was likely due to deficit irrigation and warmer temperatures. Though symptoms of salt damage from these two composts were observed at all locations, greater mortality occurred in Central Valley fields than on the Central Coast. This was likely due to lower irrigation levels and/or less effective salt leaching in the Central Valley during plant establishment. CC 1 and CC 2 had two drip lines per bed, one per row of berries, whereas both Central Valley fields had only one drip line centered between two rows of berries. Seasonal deficit irrigation could lead to minimal reduction in soil salinity over time causing salt accumulation to damaging levels in fields amended with compost high in salt. Placement of irrigation lines and/or the irrigation rates can significantly influence the extent of salt damage from compost.
The availability of plant nutrients is influenced by soil pH. Compost amendments moved soil pH closer to optimal levels (6.8–7.3), and did so most effectively in moderately acidic soils. Importantly, this effect was maintained throughout the growing season, potentially improving nutrient availability through the final harvest. The buffering capacity of compost could help ameliorate acidification of soil that can result from continued application of urea- and ammonium-based fertilizers.
Composts differ in their effects on soil nitrate levels. Vermicompost and mushroom compost were good sources of early season nitrate, and mushroom, manure, and vermicompost were good sources of slow-release nitrogen throughout the season. Mean soil nitrate in non-amended plots in June was lowest at the three coastal fields suggesting that composts are continuing to release nitrate 7 months after application. Slow release nitrogen fertilizers are important in strawberry production on the Central Coast because (1) dormant crowns are planted during the cool periods when growth is initially slow with correspondingly low nitrogen demands for the first 2 months, and (2) nitrogen is needed for an extended period to support continuous fruit production for up to 7 months. Thus, increased nitrate from compost during fruit production helps growers reach in-season nitrogen fertilization goals, which can be challenging to achieve within organic production standards.
For typical high yields achieved in coastal California, strawberries require 202–247 kg/ha of nitrogen. At 67.2 mt/ha of applied compost, estimates of total nitrogen added to each plot range from 1283 kg/ha (mushroom) to 269 kg/ha (vermicompost compost; ). However, total nitrogen does not correlate with available nitrogen. Nitrate is the most readily assimilated form of nitrogen for plants. At an application rate of 67.2 mt compost/ha, estimates of soil nitrate contribution from each compost would be: manure compost, 15.7 kg/ha; spent mushroom compost, 5.6 kg/ha; vermicompost, 5.6 kg/ha; and yard trimmings, 0.4 kg/ha. At these levels, compost would not provide sufficient nitrogen for optimal strawberry production. Therefore, although compost can contribute to a nitrogen program, it cannot suffice as a stand-alone nitrogen fertilizer source. However, with an annual compost application program, the regular addition of high total nitrogen and observed stimulation of microbial activity may lead to a greater availability of nitrate over time, potentially increasing yields over time.
Growth and yield
Compost has the potential to decrease, increase, or not affect yield. Reduced plant growth and yield in manure compost-amended plots was likely due to damaging levels of salt, as evidenced by both the high EC levels and plant symptoms. Applications at a lower rate coupled with sufficient irrigation would likely minimize this yield loss. Considering total yield from both cultivars at all locations, vermicompost had the highest mean yield of all treatments in four of seven trials, and had the highest mean yield of all composts in six out of seven trials (). In addition to total yield, timing of fruit production is important because prices change weekly, with early and late season fruit generally being more valuable. Vermicompost production curves described more productive plants later in the season, which can allow growers to capture higher, late season prices and possibly extend the production season. Adding compost every 1–2 years can slowly increase the benefits, providing long-term benefits to soil productivity.
Figure 9. Mean rank of total marketable yield across five field trials for two cultivars. Vertical bars represent the standard error of the mean.
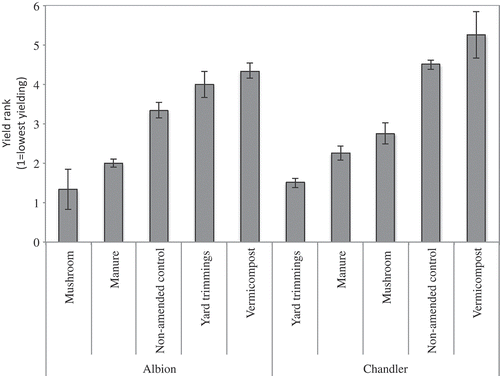
The effect of compost type on yield may be greater when strawberries are grown under less than optimal conditions. While significant yield differences between compost treatments was not consistently evident, as total yield decreased, greater differences between cumulative mean yields were apparent. In the most productive fields, CC 2 and CC-F, differences between the lowest and highest yielding treatment were 5% and 7%, respectively. In the least productive fields, CV 1 and CV 2, differences between the lowest and highest yielding treatment were 44% and 27%, respectively. This suggests that in locations where soil and/or climate are less than optimal and/or management practices are not optimized, compost and compost type can result in significant increases in mean yield. Another consideration is the history of compost application. CC 1, CC 2, and CC-F regularly apply compost whereas CV 1 and CV 2 did not; therefore, the effect of compost application on yield may be larger where compost is not a regular amendment.
Notable trends in plant growth included a larger canopy diameter from November through April in vermicompost amended soils, whereas plants in yard trimmings amended soils tended to have the slowest growth from November through February and the most rapid growth from February through April. At the same time as plant canopy diameters were measured, each plot was given a health rating based on plant vigor, evenness of plant stand, and color. Plant health was rated on a scale from one to five, from very poor to excellent, respectively (data not included). The relationship between plant health rating and growth rate over time tended to describe two scenarios: (1) slower canopy growth that advances in size and health over time (manure compost, yard trimmings) and (2) rapid early growth with large canopy and vigor earlier in the season that declines earlier (vermicompost). Composts that stimulate rapid early growth, such as vermicompost, may be particularly beneficial to short day cultivars, such as Chandler, which typically peaks in production early in the season, with the possibility of a second, lower production spike in August. This may be less true for Albion, a day-neutral cultivar that produces fruit more evenly over a production season of approximately 7 months. A trend relating plant canopy growth rate to yield was not clear for Albion.
A commonly observed response to soil fumigation is more extensive development of a fibrous root system (Wilhelm, Citation1961; Wilhelm and Paulus, Citation1980). However, root length density for ‘Albion’ in the fumigated soil (CC-F) was significantly greater than only one of the two other fields, which were both non-fumigated. It could be that root development in the fumigated field benefited from a lighter soil texture, which may have allowed deeper root penetration and hence fewer roots in the upper 10 cm, from which samples were taken. It is also possible that the expected impact of fumigation on root development, which is based on cultivars developed early in the fumigation era (Wilhelm et al., Citation1972), is less applicable to contemporary genotypes. Decades of breeding for performance in fumigated soils may have resulted in cultivars that do not require a robust root system because constraints imposed by soilborne pathogens were greatly reduced if not eliminated. Thus, a fumigation effect on root development may be less evident in recently released cultivars, such as Albion, than in short day cultivars, such as Chandler, which were predominant in the pre-fumigant era (Wilhelm et al., Citation1972).
Albion typically had a greater root length density than Chandler in May and in June, even though day neutral cultivars, such as Chandler, grow more vigorously early in the season as seen in the larger canopy diameter. Nonetheless, ‘Chandler’ showed a trend of greater root length density associating with higher fruit production. The roots of ‘Chandler’ plants growing in vermicompost amended soil at CC 1 increased significantly between 23 Apr. and 7 May. This was associated with high productivity during this time, as indicated by mean fruit production of 1416 g/plant compared to 772 and 755 g/plant produced in yard trimmings and manure amended plots. Mushroom compost-treated plots produced 1147 g/plant during this interval but also had the highest mean root length density in April, although root length declined in May, perhaps describing the intermediate production amount compared to other treatments. However, the non-amended control plots do not show the same trend. Yield during this window was highest in the non-amended control (1594 g/plant) but root length was second to lowest in April and the lowest in May. The reason for this is unclear. A similar assessment could not be made for ‘Albion’ because roots were not collected at multiple time points.
Microbial activity and disease suppression
Soil microbial activity is important for nutrient cycling and can contribute to disease suppression. Fluorescein diacetate (FDA) hydrolysis was used to provide a quick and sensitive measure of total microbial activity (Schnurer and Rosswall, Citation1982). In a meta-analysis by Bonanomi et al. (Citation2010), microbial activity as measured by FDA hydrolysis was found to be one of the most useful predictors of disease suppression. All composts evaluated led to substantial stimulation of microbial activity for a minimum of 7 months, irrespective of geographic location and native microbial populations. Increased microbial activity, as found in this study, indicates potential for disease suppression should pathogens be present. Separate studies evaluating disease suppression of these composts identified vermicompost, mushroom compost and yard trimmings as good suppressants of Pythium ultimum (Lloyd and Gordon, unpublished).
Mushroom compost had the highest level of microbial activity and the largest fungal and bacterial populations. Likewise mushroom compost induced the greatest increase in FDA hydrolysis in amended soils. Manure compost had low bacterial abundance and microbial activity as a pure sample and led to low activity in the field. Microbial activity was high in pure vermicompost, and fungi and bacteria were abundant, but little stimulation of the microbial community was evident in vermicompost amended soil. This could be due to the fact that vermicompost was applied only to the planting hole and soil core samples used for FDA hydrolysis were likely outside of the amended zone. Yard trimmings showed intermediate stimulation of microbial activity in field trials and intermediate fungal and bacterial abundance and activity as a pure compost. This study did not evaluate pathogen presence in the field soils so the contribution of microbial activity to disease suppression was not investigated at the field level.
This study demonstrates that commercially available composts derived from different source materials can significantly impact several important variables: slow-release nitrate, microbial activity, pH buffering capacity, and soil electrical conductivity. However, selection of compost is best determined based on production goals and environmental variables specific to each grower. For example, the relative importance of the impact on soil salinity will vary with irrigation technique and volume of winter rainfall. Significant effects on yield are more likely to occur where environmental conditions (soil and climate) and/or management practices are less than optimal. Further research could identify synergistic effects, such as pH buffering capacity and stimulated microbial activity on nutrient availability as part of a nutrient management program, especially for organic production.
Acknowledgments
The authors gratefully acknowledge the grower collaborators for making available their land and expertise to on-farm research. The authors are also grateful for the technical assistance from Emmi Koivunen, Lola Quasebarth, Peter Henry, Neville Mathews, and Dr. Ana Maria Pastrana Leon.
Literature cited
- Bonanomi, G., V. Antignani, M. Capodilupo, and F. Scala. 2010. Identifying the characteristics of organic soil amendments that suppress soilborne plant diseases. Soil Biol. Biochem. 42:136–144.
- Cal Recycle. Title 14, Chapter 3.1. Composting Operations Regulatory Requirements, Article 5. Composting Operation and Facility Siting and Design Standards, Section 17868.3. Pathogen Reduction, January 2010. 6 October 2016 http://www.calrecycle.ca.gov/Laws/Regulations/title14/ch31a5.html.
- de Boer, W., P.J.A. Klein Gunnewiek, P. Lafeber, J.D. Janse, B.E. Spit, and J.W. Woldendorp. 1998. Anti-fungal properties of chitinolytic dune soil bacteria. Soil Biol. Biochem., 30:193–203.
- Edwards, J.H., and A.V. Someshwar. 2000. Chemical, physical, and biological characteristics of agricultural and forest by-products for land application. In: J.F. Power and W.A. Dick (eds.). Land application of agricultural, industrial, and municipal by-products, p. 1–62. Soil Science Society of America, Madison, WI.
- IPM for Strawberries. 2008. UCANR publication 3351. Regents of the University of California Division of Agriculture and Natural Resources, Oakland, CA.
- Koike, S.T., T.R. Gordon, O. Daugovish, H. Ajwa, M. Bolda, and K. Subbarao. 2012. Recent developments on strawberry plant collapse problems in California caused by Fusarium and Macrophomina. Intl. J. Fruit Sci. 13:76–83.
- Koike, S., S.C. Kirkpatrick, and T.R. Gordon. 2009. Fusarium wilt of strawberry caused by Fusarium oxysporum in California. Plant Dis. 93(10):1077.
- Noble, R., and E. Coventry. 2005. Suppression of soil-borne diseases with composts: A review. Biocontrol Sci. Technol. 15(1):3–20.
- R Core Team. 2013. R: A language and environment for statistical computing. R Foundation for Statistical Computing, Vienna, Austria. http://www.R-project.org/.
- Schnurer, J., and T. Rosswall. 1982. Fluorescein diacetate hydrolysis as a measure of total microbial activity in soil and litter. Appl. Environ. Microbiol. 43:1256–1261.
- White, J. 2013. Special issue: Methyl bromide alternatives. Calif. Agr. 67(3): 121.
- Wilhelm, S. 1961. Diseases of strawberry. California Agricultural Experiment Station Extension Service Circulation: 494.
- Wilhelm, S., and Paulus, A.O. 1980. How soil fumigation benefits the California strawberry industries. Plant Dis. 64:264–270.
- Wilhelm, S., and J. Sagen. 1974. A history of the strawberry: from ancient gardens to modern markets. University of California, Division of Agriculture and Natural Resources, Oakland, CA.
- Wilhelm, S., P. Nelson, H. Thomas, and H. Johnson. 1972. Pathology of strawberry root rot caused by Ceratobasidium species. Phytopathology 62:700–705.
- Yuen, G.Y., M.N. Schroth, A.R. Weinhold, and J.G. Hancock. 1991. Effects of soil fumigation with methyl bromide and chloropicrin on root health and yield of strawberry. Plant Dis. 75:416–420.