ABSTRACT
The effects of combined infrared-vacuum drying parameters, including infrared power (300–400 W), pressure (5–25 kPa) and time (0–140 min) on the drying kinetics of grapefruit slices were investigated. Both the infrared lamp power and vacuum pressure influenced the drying time of grapefruit slices. The regression results showed that the quadratic model satisfactorily described the drying behavior with highest R and lowest SE values. The effective moisture diffusivity increases as power increases and range between 5.83 × 10–10 and 2.13 × 10–9 m2/s. The color scale parameters, including redness (a*), yellowness (b*) and lightness (L*) values of dried grapefruit slices increased during drying. The rise in infrared power has a negative effect on the color change intensity (∆E) and with increasing in infrared radiation power it was increased. Different kinetic models were used to fit the experimental data and the results revealed that the power model was the most suitable to describe the ∆E.
Introduction
Grapefruit is a citrus fruit with especially high amounts of ascorbic acid and phenolic compounds, which are beneficial for human health. Nowadays, techniques like infrared, vacuum and freeze drying have opened up new alternatives for the fruit processing, as it is easier to handle, package and transport the obtained fruit powder at the same time that they provide a high-quality product with a prolonged shelf life (Moraga et al., Citation2012; Salehi et al., Citation2017; Vanamala et al., Citation2005). Effect of vacuum impregnation with calcium lactate on the osmotic dehydration kinetics and quality of osmodehydrated grapefruit studied by Moraga et al. (Citation2009). the water effective diffusion coefficient is reduced from 3.64 × 10−11 to 1.80 × 10−11 m2/s when calcium lactate was added during the osmotic treatment. The objective of Castro-Vazquez et al. (Citation2016) study was to evaluate changes in bioactive flavonoids, antioxidant behavior, and in vitro cytoprotective effect of processed white and pink peels after oven-drying (45–60°C) and freeze-drying treatments. It was found that naringin, followed by isonaringin, was the main flavonoid occurring in fresh, oven-dried and freeze-dried grapefruit peels. Reaction order, activation energy and average rate constant were evaluated by dynamic modeling for color degradation of grapefruit juice during thermal processing studied by Cohen et al. (Citation1994).
One of the ways to shorten the drying time is to supply heat by infrared radiation. This method of heating is especially suitable to dry thin layers of material with large surface exposed to radiation (Allanic et al., Citation2016; Salehi et al., Citation2016a). Application of infrared heating to food drying is recently of special interest because of the progress in radiator construction. Their efficiency is between 80% and 90%, the emitted radiation is in narrow wavelength range and they are miniaturized (Salehi et al., Citation2016b). In the vacuum method the contact between the material being dried and oxygen is limited. Owing to the reduced pressure, effective drying can be achieved at low temperature (Nawirska et al., Citation2009). In recent years, infrared-vacuum drying has been investigated as a potential method for obtaining high quality dried food products, including fruits, vegetables and grains. Infrared-vacuum drying combines the advantages of both infrared heating and vacuum drying. The low temperature and fast mass transfer conferred by vacuum combined with rapid energy transfer by infrared heating generates very rapid, low temperature drying and thus it has the potential to improve energy efficiency and product quality (Giri and Prasad, Citation2007).
Color has to be considered as a special parameter that seems to be one of the first attributes of quality that a consumer perceives and may influence the consumers judgment of other attributes, such as flavor (Sayyari et al., Citation2017). The procedure for color assessment is based on the determination of the Hunter L*a*b* values. The L* values are used as an indicator of brightness, a* to indicate chromaticity on a green (−) to red (+) axis, and b* to indicate chromaticity on a blue (−) to yellow (+) axis (Salehi and Kashaninejad, Citation2015).
Color changes and mass transfer phenomena take place during drying cause physicochemical changes, which affect the color and surface of the dried products. We found no report on infrared-vacuum drying of grapefruit slices in the literature. Therefore, the aim of the present work was to investigate infrared-vacuum drying characteristics of grapefruit slices in respect to drying kinetics, moisture diffusivity, surface changes and color changes kinetics of the dried products.
Materials and methods
Infrared-vacuum drying of grapefruit
Grapefruit was purchased from a local supermarket and stored at 7°C and 90–95% relative humidity. The initial moisture content of grapefruit was 91 ± 1.1. Slices of grapefruit with 0.5 cm thickness were prepared with the aid of a steel cutter and were immediately placed into the dryer ().
The grapefruit slices were dried in an infrared-vacuum dryer (infrared radiation lamp (NIR), Philips, Germany; Vacuum oven (VO), Memmert Universal, Schwabach, Germany). The effect of infrared radiation power (at three levels 300, 350 and 400 W), system pressure (at three levels 5, 15 and 25 kPa), time (0–130 min) on the drying kinetics and characteristics of grapefruit slice were investigated. Weight loss of samples was recorded by using a digital balance (Digital balance, LutronGM-300p, Taiwan), with a sensitivity of ±0.01 g.
Drying kinetics
The experimental moisture content data were nondimensionlized using the equation:
where MR is the dimensionless moisture ratio, Mt, M0 and Me are moisture content at any time, initial moisture content and equilibrium moisture content (kg water/kg dry matter), respectively. (Mt–Me)/(M0–Me) was simplified to Mt/M0 as the relative humidity of drying air continuously fluctuated during drying experiments (Ceylan et al., Citation2007; Doymaz, Citation2011).
Selected thin-layer drying models, detailed in , were fitted to the drying curves (MR vs. time) (Akpinar and Bicer, Citation2005; Doymaz, Citation2011). A nonlinear estimation package (Curve Expert, Version 1.34) was used to estimate the coefficients of the given models. The two criteria of statistic analysis have been used to evaluate the adjustment of the experimental data to the different models, R (correlation coefficient) and SE (standard error). A good fitting between the experimental data and the correlations is obtained when there is a combination of the high R value and the values of SE, which should be as low as possible (Doymaz, Citation2011).
Table 1. Mathematical models used to kinetics modeling of grapefruit drying.
Calculation of moisture diffusivity
Fick’s second law of diffusion has been widely used to describe the drying process during the falling rate period for most food materials (Sacilik, Citation2007). The solution of diffusion model, considering negligible external resistance, in terms of average moisture content, negligible shrinkage, constant diffusion coefficients and temperature, is presented for slab geometry (Doymaz, Citation2011):
where MR is the moisture ratio (dimensionless); t is the drying time (s), Deff is the effective diffusivity (m2/s), and L is the half slab thickness of the slices (m). For long drying periods, Equation (2) can be further simplified to:
The effective diffusivity was calculated through Equation (3) by using the method of slopes. The effective diffusivities are typically determined by plotting experimental drying data in terms of lnMR versus time (as given in Equation (3)). From Equation (3), a plot of lnMR versus time gives a straight line with a slope (K) of:
Color measurement
In order to investigate the effect of drying temperature on color changes of dried grapefruit, a computer vision system was applied. Sample illumination was achieved with HP Scanner (Hp Scanjet G3110). Since the computer vision system perceived color as RGB signals, which is device-dependent, the taken images were converted into L*a*b* units to ensure color reproducibility (Salehi, Citation2017).
The calculation of color changes (ΔE) for total color difference was made with the following equations:
The change in the surface of the grapefruit slice during drying is calculated using the following equation:
Where ΔA is the surface change percent (dimensionless), A0 and At (m2) are surface of grapefruit at first time (fresh grapefruit) and drying period, respectively.
In this study, the image analysis of dried gums was performed using Image J software version 1.42e, USA.
Color changes kinetics
The power (Equation (7)) and quadratic (Equation (8)) models were chosen to describe the color changes intensity (ΔE) within the drying process:
Power model:
Quadratic model:
The experimental data were modeled by using non-linear regression in Curve Expert 1.34 software.
Results and discussion
Drying time
Although juice production is the most important process for citrus fruits, the development of a drying process for the production of powders, flakes and slices also promotes their consumption. The effects of infrared power and vacuum pressure on the moisture loss of grapefruit slices are shown in and , respectively. As expected, the moisture loss was decreased by increasing the power because of the increased temperature and heat transfer gradient between the air and samples. The drying times of grapefruit samples were 130, 120 and 100 min at 300, 350 and 400 W, respectively (15 kPa). In conclusion, experimental results showed that the infrared power has a significant effect on the evolution of moisture loss. With increasing infrared intensity, due to the increase in sample temperature and evaporation rate and the decrease in drying time, the specific energy for drying of grapefruit slices decreases.
Figure 2. Variations of moisture loss with drying time of grapefruit slices at different infrared power (15 kPa).
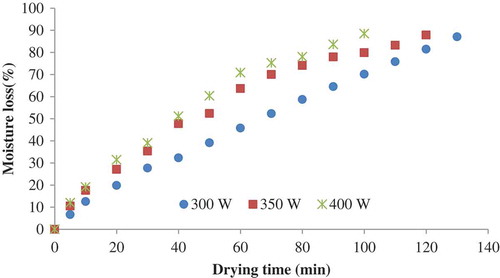
Figure 3. Variations of moisture loss with drying time of grapefruit slices at different system pressure (300 W).
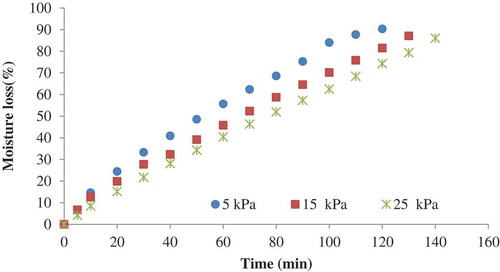
The influence of vacuum pressure on the moisture loss as a function of drying time at an infrared power of 300 W is shown in . It is evident that increase in vacuum pressure enhanced the drying rate. This was expected because as vacuum pressure increases, there is an accelerated removal of moisture buildup in the chamber, which consequently enhanced the drying process. In combined infrared-vacuum drying, the most important effect is to cause an increase in drying rate. In our study, no burning of dried grapefruit slices was observed even at the highest power of 400 W in combination with the various vacuum pressures. The general improved quality of the dried products using this infrared-vacuum dryer may be due to the combined effects of increased pressure gradient between the inner and outer layers of the grapefruit, as well as the low temperatures maintained throughout the drying process.
Masamura et al. (Citation1988) observed increased drying rates of food products with increasing power supply to infrared heater. The increase in power might have caused a rapid increase in the temperature at surface of product, resulting into an increase in the water vapor pressure inside the product and thus in higher drying rates (Datta and Ni, Citation2002).
Vacuum drying is a unit operation in chemical and engineering process, in which moist material is dried under sub-atmospheric pressures. The lower pressure allow drying temperature to be reduced and higher quality to be obtained than with classical air conventional process at atmospheric pressure (Arévalo-Pinedo and Murr, Citation2007). The drying time reduced from 140 to 120 min when the vacuum pressure was decreased from 250 to 50 kPa (300 W). The experimental results are consistent with the literature reports for other vegetables (Toğrul, Citation2006). Microwave-vacuum drying resulted in 70–90% decrease in the drying time and the dried products had better rehydration characteristics as compared to convective air drying (Giri and Prasad, Citation2007).
Infrared-vacuum dryer system was developed and tested by Mongpraneet et al. (Citation2002) using welsh grapefruit as a test material. The results showed that the rate of moisture reduction of the sample decreased with an increase in the power input to the far infrared radiator. This is expected as higher power input implied higher radiation intensity. However, when a very low level of the power input (40 W) was applied the required final moisture content of grapefruit could not be achieved.
Fitting of the drying curves
Studying drying kinetics is a means to choose appropriate drying methods and to control the processes of drying. It is also important for engineering and process optimization. It is sometime expensive to conduct a full scale experiment to determine the suitable condition for drying. Drying kinetics is used to express the moisture removal process and its relation to the process variables and hence, a good understanding of the drying rate is important to develop a drying model (Maisnam et al., Citation2017). The drying kinetics models are essential for equipment design, process optimization and product quality improvement. Different mathematical models () were fitted with the drying data and among the all drying models; the quadratic model obtained the highest R values and the lowest SE values. Estimated parameters and statistical data obtained for this model are shown in . In all the cases, the R values for the models were greater than 0.997, indicating a good fit. Salehi et al. (Citation2017) reported that the Page model could adequately describe the combined infrared-vacuum drying of button mushroom slices.
Table 2. Curve-fitting coefficients of the quadratic model.
Suitability of thin layer models for infrared–hot air drying of grapefruit slices was studied by Praveen Kumar et al. (Citation2006). Thin layer models, such as Page, modified Page, Fick’s and Exponential models, which are used to describe the drying kinetics of food materials, were tested for the combination mode drying. The modified Page model gave better predictions for drying characteristics over the other models.
The six mathematical models were used to fit the experimental data of grapefruit seeds. The statistical results indicated that Henderson model presented the best adjustment to describe the water sorption isotherm for grapefruit seeds under the different conditions (Cantu-Lozano et al., Citation2013). Also, The Fick’s second law and Page models proved to better represent the experimental data of grapefruit seeds. Both models presented the highest R2 and the lowest error values.
Moisture diffusivity
The effective diffusivities are determined by plotting experimental drying data in terms of lnMR versus time. The effects of infrared radiation power and vacuum pressure on the lnMR are shown in and , respectively. The Deff values lie within in general range of 10–11 to 10–9 m2/s for food materials (Rizvi, Citation1986). The values of Deff at different condition drying of grapefruit slice obtained by using Equation (4) and estimated values are shown in . The effective diffusivity values of grapefruit slices were ranged from 5.83 × 10–10 to 2.13 × 10–9 m2/s. Effective diffusivity values increased with increasing infrared radiation power because of the rapid movement of water at high temperatures (Doymaz, Citation2011). The values of Deff are comparable with the reported values of 0.83 to 2.33 × 10–9 m2/s for button mushroom slices (Salehi et al., Citation2017), 8.039 × 10−10 to 20.618 × 10−10 m2/s for mushroom (Darvishi et al., Citation2013), 0.71 to 2.86 × 10–9 m2/s for pumpkin (Cucurbita moschata) (Hosseini Ghaboos et al., Citation2016), and 4.27 to 13.0 × 10–10 m2/s for okra (Doymaz, Citation2005). These values are consistent with the present estimated Deff values for grapefruit slices. The results of such fitting gave a regression coefficient of 0.953 indicating that the quality of such a fitting was satisfactory. Sorption isotherms and drying kinetics of grapefruit seeds was studied by Cantu-Lozano et al. (Citation2013). Drying rates indicated that the drying of grapefruit seeds took place under the falling rate period. Effective moisture diffusivity in grapefruit seeds ranged from 4.36 × 10–10 to 6.82 × 10–10 m2 s−1.
Table 3. Values of effective moisture diffusivity of grapefruit slice obtained from drying experiments.
Table 4. The values of the kinetic model coefficients of color change (ΔE) during drying of grapefruit.
Color change
shows changes in L*, a* and b* values of grapefruit slices for increasing drying times and infrared power during drying. L* is a critical parameter in the drying industry as it is usually the first quality attribute evaluated by consumers when determining product acceptance. Low L* values indicate a dark color and are mainly associated with non-enzymatic browning reactions (Dueik et al., Citation2010). The results of the experimental lightness data are shown in . As shown in this figure, the L* value of dried grapefruit increased during drying by combined infrared-vacuum dryer. For instance, L* value diminished from L0* = 50.70 to L* = 60.98 at end point when drying at 400 W and 5 kPa pressure. Infrared power has a positive effect on the lightness of dried grapefruit slices. As the infrared power of drying increased from 300 to 400 W, the lightness of grapefruit increased from 56.70 to 60.98, respectively. In general an L* parameter decrease is not desired because that means a more dark product, which is not acceptable for dried grapefruit. Decrease in L* value has been linked with nonenzymatic browning reactions that accelerates at high temperatures (Dueik et al., Citation2010; Mariscal and Bouchon, Citation2008). Similar behavior in fried potato chips was reported by Nourian et al. (Citation2003). Since lightness is a very important color quality parameter, lower drying temperatures with lower boiling point of water are preferable to preserve the lightness and hence the attractiveness of fried products (Oyedeji et al., Citation2017). In addition, the reduction in L* may be attributed to intense browning reaction and increase crust formation due to exposure to high temperature (Mariscal and Bouchon, Citation2008).
Figure 6. Effect of infrared power on lightness (L*), redness (a*) and yellowness (b*) parameters during drying of grapefruit slices at 5 kPa system pressure.
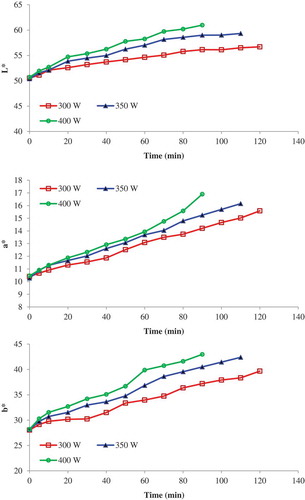
As with the case of the redness parameter, a* is also affected by the infrared power and drying time (). As shown in this figure the redness of dried grapefruit increased during drying (become more red). As shown in , the yellowness of dried grapefruit slices increased during drying. In general higher b* parameter values give more yellow products, which is desirable for dried products. Grapefruit slices dried under different infrared power showed a increase in their color coordinates, reaching final values of L* = 60.978, a* = 16.902 and b* = 42.971, when dried at 400 W and 5 kPa system pressure. Swasdisevi et al. (Citation2007) studied the drying behavior of banana slices undergoing combined infrared-vacuum drying. The results showed that lightness decreased with increasing surface temperature, while yellowness increased with increasing surface temperature due to browning reaction occurring during drying process.
In color change (ΔE) is presented as functions of drying time and infrared power. As shown in this figure, the ΔE increased during drying of grapefruit. The color change intensity (ΔE) gets more intense at higher power. The increase in magnitude of total color difference values could be attributed to the high temperature and low moisture content, which initiated the nonenzymatic browning, such as Maillard reaction and caramelization of sugars (Baik and Mittal, Citation2003). Therdthai and Zhou (Citation2009) reported that high temperature during drying of mint leaves could lead to increase ΔE values (Therdthai and Zhou, Citation2009). The rise in power has a negative effect on the ΔE and with increasing in infrared radiation power from 300 to 400 W, it was increased from 14.14 to 18.96 (5 kPa), respectively.
Figure 7. Effect of infrared power on color change intensity (ΔE) during drying of grapefruit slices at 5 kPa system pressure.
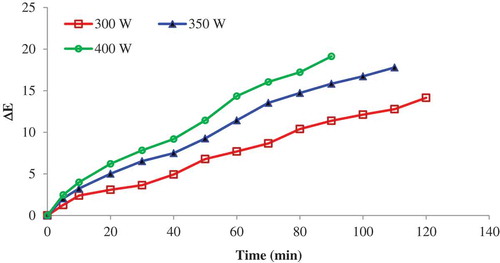
The mathematical models were fitted to the color change (ΔE) data and the parameter estimation resulted from fitting Equations (7) and (8) to the experimental data. The power model gave better predictions for color change intensity over the quadratic model. The results of fitting the power model to the experimental data are reported in .
Surface change
Surface change % (shrinkage) is a common phenomenon during drying. Rapid water loss resulted in significant shrinkage in grapefruit during drying (Maity et al., Citation2014). In surface change is presented as functions of drying time and infrared power. As shown in this figure, the surface change % was increased with the progression of drying time. As soon as the grapefruit slices were introduced in the low infrared power, the slices shrunk. As the infrared power increased from 300 to 400 W, the surface change % of grapefruit slices decreased from 34.68% to 41.06%, respectively. Similar behavior was also reported in change in shrinkage during vacuum frying of banana chips (Yamsaengsung et al., Citation2011). Higher shrinkage in potato chips was also reported during high temperature frying (Yagua and Moreira, Citation2011).
Conclusion
In this study a vacuum drying system was fitted with different infrared lamp for radiative heating. The drying characteristics were satisfactorily described by quadratic model with the latter providing the best representation of the experimental data. Values for the effective moisture diffusivity of grapefruit samples were obtained in the range of 5.83 × 10–10 and 2.13 × 10–9 m2/s. The present study also verified that the color parameter (L*, a*, b* and ΔE) of grapefruit was influenced by the drying process condition (infrared power and system pressure). L*, a* and b* values of dried grapefruit slices increased during drying. The rise in power has a negative effect on the color change intensity and with increasing in infrared radiation power from 300 to 400 W, it was increased from 14.14 to 18.96 (5 kPa), respectively. Different kinetic models were used to fit the experimental data and the results revealed that the power model was the most suitable to describe the color change intensity (∆E). At the end of drying the % surface change in grapefruit slices at 5 kPa pressure was 34.68, 37.27 and 41.06 at 300, 350 and 400 W, respectively.
References
- Akpinar, E.K., and Y. Bicer. 2005. Modelling of the drying of eggplants in thin‐layers. Int. J. Food Sci. Tech. 40:273–281. doi:10.1111/ifs.2005.40.issue-3.
- Allanic, N., P. Le Bideau, P. Glouannec, and R. Deterre. 2016. An experimental study on infrared drying kinetics of an aqueous adhesive supported by polymer composite. Heat and Mass Transfer. 53(1):223–231.
- Arévalo-Pinedo, A., and F.E.X. Murr. 2007. Influence of pre-treatments on the drying kinetics during vacuum drying of carrot and pumpkin. J. Food Eng. 80:152–156. doi:10.1016/j.jfoodeng.2006.05.005.
- Baik, O., and G. Mittal. 2003. Kinetics of tofu color changes during deep-fat frying. LWT-Food Sci. Technol. 36:43–48. doi:10.1016/S0023-6438(02)00175-5.
- Cantu-Lozano, D., J. Viganó, A. Alvarado Lassman, N.A. Vallejo Cantu, and J. Telis-Romero. 2013. Sorption isotherms and drying kinetics of grapefruit seeds. Acta Scientiarum. Technol. 35. doi:10.4025/actascitechnol.v35i4.13658.
- Castro-Vazquez, L., M.E. Alañón, V. Rodríguez-Robledo, M.S. Pérez-Coello, I. Hermosín-Gutierrez, M.C. Díaz-Maroto, J. Jordán, M.F. Galindo, and M.D.M. Arroyo-Jiménez. 2016. Bioactive flavonoids, antioxidant behaviour, and cytoprotective effects of dried grapefruit peels (Citrus paradisi Macf.). Oxid. Med. Cell Longev. 2016:1–12. doi:10.1155/2016/8915729.
- Ceylan, I., M. Aktaş, and H. Doğan. 2007. Mathematical modeling of drying characteristics of tropical fruits. Appl. Therm. Eng. 27:1931–1936. doi:10.1016/j.applthermaleng.2006.12.020.
- Cohen, E., Y. Birk, C.H. Mannheim, and I.S. Saguy. 1994. Kinetic parameter estimation for quality change during continuous thermal processing of grapefruit juice. J. Food Sci. 59:155–158. doi:10.1111/j.1365-2621.1994.tb06922.x.
- Darvishi, H., G. Najafi, A. Hosainpour, J. Khodaei, and M. Aazdbakht. 2013. Far-infrared drying characteristics of mushroom slices. Chem. Prod. Model. 8:107–117. doi:10.1515/cppm-2013-0035.
- Datta, A., and H. Ni. 2002. Infrared and hot-air-assisted microwave heating of foods for control of surface moisture. J. Food Eng. 51:355–364. doi:10.1016/S0260-8774(01)00079-6.
- Doymaz, I. 2011. Drying of eggplant slices in thin layers at different air temperatures. J. Food Process. Preserv. 35:280–289. doi:10.1111/j.1745-4549.2009.00454.x.
- Doymaz, İ. 2005. Drying characteristics and kinetics of okra. J. Food Eng. 69:275–279. doi:10.1016/j.jfoodeng.2004.08.019.
- Dueik, V., P. Robert, and P. Bouchon. 2010. Vacuum frying reduces oil uptake and improves the quality parameters of carrot crisps. Food Chem. 119:1143–1149. doi:10.1016/j.foodchem.2009.08.027.
- Giri, S., and S. Prasad. 2007. Drying kinetics and rehydration characteristics of microwave-vacuum and convective hot-air dried mushrooms. J. Food Eng. 78:512–521. doi:10.1016/j.jfoodeng.2005.10.021.
- Hosseini Ghaboos, S.H., S.M. Seyedain Ardabili, M. Kashaninejad, G. Asadi, and M. Aalami. 2016. Combined infrared-vacuum drying of pumpkin slices. J. Food Sci. Technol. 53:2380–2388. doi:10.1007/s13197-016-2212-1.
- Maisnam, D., P. Rasane, A. Dey, S. Kaur, and C. Sarma. 2017. Recent advances in conventional drying of foods. J. Food Technol. Preserv. 1(1):24–34
- Maity, T., A. Bawa, and P. Raju. 2014. Effect of vacuum frying on changes in quality attributes of jackfruit (Artocarpus heterophyllus) bulb slices. Int. J. Food Sci. 2014:1–8. doi:10.1155/2014/752047.
- Mariscal, M., and P. Bouchon. 2008. Comparison between atmospheric and vacuum frying of apple slices. Food Chem. 107:1561–1569. doi:10.1016/j.foodchem.2007.09.031.
- Masamura, A., H. Sado, T. Honda, M. Shimizu, H. Nabetani, M. Nakajima, and A. Watanabe. 1988. Drying of potato by far infrared radiation. J. Jpn. Soc. Food Sci. Technol. (Japan) 35:309–314. doi:10.3136/nskkk1962.35.309.
- Mongpraneet, S., T. Abe, and T. Tsurusaki. 2002. Far infrared–vacuum and –convection drying of welsh onion. Trans. ASAE 45:1529. doi:10.13031/2013.11045.
- Moraga, G., M. Igual, E. García-Martínez, L.H. Mosquera, and N. Martínez-Navarrete. 2012. Effect of relative humidity and storage time on the bioactive compounds and functional properties of grapefruit powder. J. Food Eng. 112:191–199. doi:10.1016/j.jfoodeng.2012.04.002.
- Moraga, M.J., G. Moraga, P.J. Fito, and N. Martínez-Navarrete. 2009. Effect of vacuum impregnation with calcium lactate on the osmotic dehydration kinetics and quality of osmodehydrated grapefruit. J. Food Eng. 90:372–379. doi:10.1016/j.jfoodeng.2008.07.007.
- Nawirska, A., A. Figiel, A.Z. Kucharska, A. Sokół-Łętowska, and A. Biesiada. 2009. Drying kinetics and quality parameters of pumpkin slices dehydrated using different methods. J. Food Eng. 94:14–20. doi:10.1016/j.jfoodeng.2009.02.025.
- Nourian, F., H. Ramaswamy, and A. Kushalappa. 2003. Kinetic changes in cooking quality of potatoes stored at different temperatures. J. Food Eng. 60:257–266. doi:10.1016/S0260-8774(03)00046-3.
- Oyedeji, A., O. Sobukola, F. Henshaw, M. Adegunwa, A. Ijabadeniyi, L. Sanni, and K. Tomlins. 2017. Effect of frying treatments on texture and colour parameters of deep fat fried yellow fleshed cassava chips. J. Food Qual. 2017:1-10. doi:10.1155/2017/8373801.
- Praveen Kumar, D.G., H.U. Hebbar, and M.N. Ramesh. 2006. Suitability of thin layer models for infrared–hot air-drying of onion slices. LWT Food Sci. Technol. 39:700–705. doi:10.1016/j.lwt.2005.03.021.
- Rizvi, S.S. 1986. Thermodynamic properties of foods in dehydration, p. 239–251. In: M.A. Rao and S.S.H. Rizvi (eds.). Engineering properties of foods. New York, USA: Marcel Dekker, Inc.
- Sacilik, K. 2007. Effect of drying methods on thin-layer drying characteristics of hull-less seed pumpkin (Cucurbita pepo L.). J. Food Eng. 79:23–30. doi:10.1016/j.jfoodeng.2006.01.023.
- Salehi, F. 2017. Rheological and physical properties and quality of the new formulation of apple cake with wild sage seed gum (Salvia macrosiphon). J. Food Meas. Charact. 11:2006–2012. doi:10.1007/s11694-017-9583-5.
- Salehi, F., and M. Kashaninejad. 2015. Effect of drying methods on rheological and textural properties, and color changes of wild sage seed gum. J. Food Sci. Technol. 52:7361–7368. doi:10.1007/s13197-015-1849-5.
- Salehi, F., M. Kashaninejad, E. Akbari, S.M. Sobhani, and F. Asadi. 2016a. Potential of sponge cake making using infrared–hot air dried carrot. J. Texture Stud. 47:34–39. doi:10.1111/jtxs.2016.47.issue-1.
- Salehi, F., M. Kashaninejad, F. Asadi, and A. Najafi. 2016b. Improvement of quality attributes of sponge cake using infrared dried button mushroom. J. Food Sci. Technol. 53:1418–1423. doi:10.1007/s13197-015-2165-9.
- Salehi, F., M. Kashaninejad, and A. Jafarianlari. 2017. Drying kinetics and characteristics of combined infrared-vacuum drying of button mushroom slices. Heat Mass Transfer 53:1751–1759. doi:10.1007/s00231-016-1931-1.
- Sayyari, M., F. Salehi, and D. Valero. 2017. New approaches to modeling methyl jasmonate effects on pomegranate quality during postharvest storage. Int. J. Fruit Sci. 17:374–390. doi:10.1080/15538362.2017.1329051.
- Swasdisevi, T., S. Devahastin, R. Ngamchum, and S. Soponronnarit. 2007. Optimization of a drying process using infrared-vacuum drying of Cavendish banana slices. Optimization 29:810.
- Therdthai, N., and W. Zhou. 2009. Characterization of microwave vacuum drying and hot air drying of mint leaves (Mentha cordifolia Opiz ex Fresen). J. Food Eng. 91:482–489. doi:10.1016/j.jfoodeng.2008.09.031.
- Toğrul, H. 2006. Suitable drying model for infrared drying of carrot. J. Food Eng. 77:610–619. doi:10.1016/j.jfoodeng.2005.07.020.
- Vanamala, J., G. Cobb, N.D. Turner, J.R. Lupton, K.S. Yoo, L.M. Pike, and B.S. Patil. 2005. Bioactive compounds of grapefruit (Citrus paradisi Cv. Rio Red) respond differently to postharvest irradiation, storage, and freeze drying. J. Agric. Food Chem. 53:3980–3985. doi:10.1021/jf048167p.
- Yagua, C.V., and R.G. Moreira. 2011. Physical and thermal properties of potato chips during vacuum frying. J. Food Eng. 104:272–283. doi:10.1016/j.jfoodeng.2010.12.018.
- Yamsaengsung, R., T. Ariyapuchai, and K. Prasertsit. 2011. Effects of vacuum frying on structural changes of bananas. J. Food Eng. 106:298–305. doi:10.1016/j.jfoodeng.2011.05.016.