ABSTRACT
Wind exposure may reduce the snowpack and increase frost damage to lowbush blueberry (Vaccinium angustifolium Ait.). Farmers in the Saguenay-Lac-Saint-Jean region (Québec) have therefore installed natural windbreaks to maintain a deep snow cover. We verified the efficiency of 39 windbreaks distributed over two farms. Over two consecutive years, snow depth was measured along transects established perpendicular to these windbreaks. In the first summer, frost damage and yields were assessed at each point where snow depth had been measured. We found large fluctuations in snow depth along most transects, with deeper snow occurring within 20 m on either side of windbreaks. Relative snow depth distribution was correlated between years on 19 transects. On one farm, one third of total transect length was below the recognized critical depth of 30 cm. On this farm, the proportion of damaged stems, the mean length of stem damage and the damaged-to-total stem length decreased exponentially with increasing snow depth. Likewise, the number of fruits per stem decreased exponentially with the length of stem damage and increased linearly with snow depth. Given current trends in climate change, our results serve as a timely call for improving winter windbreak design in the Saguenay-Lac-Saint-Jean region.
Introduction
The Saguenay–lac-Saint-Jean (SLSJ) region represents the most northerly district in the province of Quebec (Canada) where agriculture is a chief staple to the local economy. The major crops of the region are adapted to the short cool summers and, in the case of perennial crops, to severe winter climates. The most notable of these is the cultivation of wild lowbush blueberry (Vaccinium angustifolium Ait.), which is practised by 419 landowners and generates annual revenues of over 40 million $ (MAPAQ, Citation2016). Lowbush blueberry is an ericaceous shrub that develops in boreal forest understories, but persists after major forest disturbance such as wildfire (Moola and Mallik, Citation1998; Thiffault et al., Citation2015). Post-disturbance light conditions result in a high production of berries, which can be maintained by managing the competing vegetation.
Typically, commercial blueberry fields are created by clearing forest patches and then burning and/or chipping the remaining slash. In the SLSJ region, these fields have grown progressively larger over the years (i.e. average = 69 ha: MAPAQ, Citation2016), creating open spaces that allows for wind speeds to increase. In winter, these high winds may sweep away the snow and expose vegetative and floral buds to cold air temperatures, which can typically linger between −25 and −40°C for several days, sometimes weeks, each year in the SLSJ region. In comparison, lowbush blueberry buds were shown to be killed or severely damaged when exposed to winter temperatures below −20°C, or to spring temperatures below −5°C (Cappiello and Dunham, Citation1994). Fortunately, snow has a low thermal conductivity such that a ground snow layer will retain the soil’s latent heat and thus act as an insulator. For example, Pomeroy and Brun (Citation2001) reported that a 50 cm snow cover resulted in soil surface temperatures of around 0°C when air temperature was at −20°C. Hence, one solution to prevent frost damage is to install porous snow fences, which reduce wind speed and allow the snowpack to accumulate (Lemieux et al., Citation1995). These snow fences represent, however, a monetary cost to farmers and require time to be installed, removed and maintained each year. A more practical solution is to plant widely spaced tree rows that act as natural windbreaks to maintain the snowpack (Read et al., Citation1996). Accordingly, many blueberry producers in the SLSJ region have installed rows of Jack pine (Pinus banksiana Lamb.) and red pine (P. resinosa Ait.) in their fields, oriented perpendicularly to the prevailing north-westerly winds.
One of the assumptions regarding winter windbreaks is that their efficiency decreases gradually with distance, making it possible to prescribe the optimal distance between tree rows. For example, Percival et al. (Citation2002) measured decreasing blueberry yields with increasing distance from windbreaks and assumed that this was due to a gradual decline in snow-depth and micro-climatic conditions. It has long been known, however, that snowpack distribution will depend on windbreak architecture, notably its porosity (Brandle et al., Citation2009; Kort, Citation1988). Windbreaks that are too opaque may create turbulence in the immediate leeward side of tree rows, whereas those that are too porous do not sufficiently reduce wind speed (Perri and Bloomberg, Citation2002; Rouse and Hodges, Citation2004). Thus, there is disagreement about the optimum porosity of windbreaks, with some proponents advocating 50% porosity (Current et al., Citation2012; Guyot, Citation1977), some advocating 60–70% (Fortin et al., Citation2013) and still others recommending up to 80% porosity (Vézina, Citation2001). There is a need, therefore, to evaluate whether the standard windbreak architecture that is used in the SLSJ region results in the intended deeper snowpack as well as in a gradual decrease in snow depth with distance from tree rows.
Few landowners, if any, regularly monitor snow depth distribution in their fields to verify the efficiency of their windbreaks, as this activity may be time consuming. There is a need, therefore, to evaluate whether snow depth measurements taken only once during the middle of winter is sufficient to inform landowners on potential winter frost damage and loss of yields the following summer. It could be argued that frost damage is not necessarily caused during the coldest winter months. For example, flowering buds may be damaged by cold temperatures during their developmental stage in early spring (Yarborough, Citation1999). Hiemstra and Liston (Citation2014) explain, however, that deeper winter snowpacks on the leeward side of tree boundaries may delay the onset of snow-free days and thus reduce the damage caused by extreme early-season temperatures. Accordingly, a study from Minnesota (Wildung and Sargent, Citation1989), in which snow depth was experimentally manipulated, showed that mid-winter snow depth does correlate with blueberry yields the following summer.
Winter windbreaks in blueberry fields tend to be of similar architecture and layout in the SLSJ region, in that they are composed of local pine species erected at 50–200 m intervals, with branches pruned from the bottom third of the stem to increase porosity (Gagnon et al., Citation2016; ). This raises the question of whether the correlation between tree-row-distance and snow depth, and between snow depth and blueberry yields, can be generalized within and across farms and windbreaks. Also, as the total annual snowpack is variable, it is important to verify whether the relative distribution of snow remains the same from year to year. The ability to generalize and extrapolate snow pack distribution over space and time would facilitate the transfer of knowledge on windbreak efficiency to landowners.
Table 1. Physical characteristics of our two study locations; meteorological data obtained from Environnement Canada (Citation2015).
We report on a study where we verified whether windbreaks (N = 39) in the SLSJ region resulted in monotonically decreasing snow packs on the leeward side of tree rows. We also verified whether the same patterns of snow-depth distribution were recurring from year to year. Finally, we verified whether a single mid-winter snow-depth measurement was correlated to frost damage and blueberry yields the following summer.
Material and Methods
Experimental Sites
Our study was performed on two commercial lowbush blueberry farms in the SLSJ region. The first is located near the Town of Saint-Méthode (48°43ʹ35” N, 72°24ʹ50” W) while the second is located near the Town of Saint-Honoré (48°32ʹ00” N, 71°05ʹ00” W). A description of each site is given in . Lowbush blueberry production at each farm comprises one year of vegetative growth alternating with either one or two harvest years. During vegetative years, producers may apply herbicides, fertilizers, fungicides and/or insecticides. Blueberry bush density is 3000–3600 ha−1 and average yields are ca. 1500 kg ha−1.
Snow-Depth Measurements
In mid-February 2015, transects were established perpendicular to windbreaks at each farm (11 and 28 transects at Saint-Méthode and Saint-Honoré, respectively). Walking along each transect, we measured snow depth at regular short intervals using a meter stick. Snow depth was only recorded, however, when snow depth changed by more than 2–3 cm from the previous measurement. Each time snow depth was recorded, the geographic location was also recorded using GPS coordinates. Snow depth was measured a second time at the same GPS coordinates in mid-February 2016.
Assessing Frost Damage
In July 2015, a harvest year at both farms, we revisited each GPS point in order to assess winter frost damages and yields. First, we established a quadrat (625 cm2) in which we counted the total number of stems and the total number of damaged stems (i.e. > 5 mm of necrotic discoloration). We then randomly sampled 15 stems within a 1 m radius circle around each quadrat and noted their total height, the length of the frost damaged portion of the stem and the number of fruits per stem.
Statistical Analyses
1. Correlating snow pack distribution between years
Given the inter-annual differences in snowfall, it was necessary to standardize snow depth data in order to test the correlation in snow pack distribution between sampling years. To do so, we first converted snow depth at each GPS coordinate as a percentage of the maximum snow depth along that given transect. We then plotted a spline curve to smooth the snow depth data across sampling points using Microsoft Excel software (Microsoft® Excel®, Citation2013). Each transect was then divided into 10 equal distances, and the relative snow depth at each distance was estimated along the spline curve using the open source ImageJ software (Schneider et al., Citation2012). For each transect, we then correlated the relative snow depth between sampling years using Pearson’s correlation coefficient.
2. Comparison of Snow Pack Distribution Across Transects
The spline curves, approximating the continuous snow depth in each transect and in each year, were split in half. The “total snowpack” (i.e. area under spline curve) in both the windward and leeward halves of each transect was then calculated using the open source ImageJ software (Schneider et al., Citation2012). For each transect half, we also calculated the difference between the maximum and minimum snow depth (i.e. “amplitude”) as well as the lowest snow depth value (“lowpoint”). Then, for each farm and each sampling year, we tested for significant differences between windward versus leeward transect halves in terms of total snowpack, amplitude and lowpoint using Wilcoxon’s matched-pairs signed ranks test.
3. Relating snow depth to frost damage
For each GPS coordinate, we plotted the proportion of damaged stems within each quadrat as a function of snow depth measured in the previous winter (2015). We then plotted the mean length of frost damage to the 15 surrounding stems, as well as their damaged-to-total stem length, as a function of snow depth. Finally, we plotted the average number of fruits per stem as a function of the damaged stem length as well as snow depth. The significance of these relationships was tested with either linear or exponential least squares regressions, using the R Studio software Version 3.1.0, Vienna, Austria (R Development Core Team, Citation2014).
Results
Snowpack Distribution
Snow depth distribution between sampling years was significantly (P < 0.05) correlated on 8 of the 11 transects at Saint-Méthode () and on 11 of the 28 transects at Saint-Honoré. For all windbreaks, snowpack depth was not monotonically decreasing on the leeward side of tree rows. Instead, large fluctuations in snow depth were observed within most transects, notably at Saint-Méthode in 2015 (). In that particular year, the total snowpack accumulation at Saint-Méthode was significantly higher on the windward than on the leeward side of transects (). That same year, the average snow depth amplitude at both sites was significantly (P < 0.05) higher on the windward than on the leeward side of transects. All other comparisons in snowpack distribution were not significant ().
Table 2. Results of Wilcoxon matched-pairs signed ranks test comparing windward vs. leeward halves of transects in terms of three snowpack attributes at each site.
Figure 1. Representation of snow depth distribution along seven continuous transects at the Saint-Méthode site. The best correlations in snow depth distribution between years were found along these seven transects. Tree rows are represented by dashed vertical lines. The windward and leeward sides are respectively the right and left hand sides of windbreaks and transects.
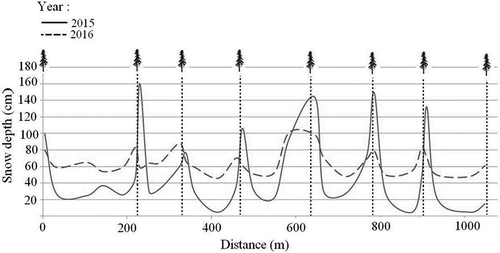
Frost Damage
The proportion of damaged stems in the sampled quadrats at Saint-Méthode decreased exponentially with increasing snow depth (). These damages, estimated from our regression model, ranged from 20% to 77% across the range of measured snow depths. Likewise, the mean length of stem damage (), as well as the damaged-to-total stem length (), decreased exponentially with snow depth. From the corresponding regression models, length of stem damage decreased from 9.5 to 0.4 cm whereas damaged-to-total stem length decreased from 37% to 2% across the range of measured snow depths. The mean number of fruits per stem decreased exponentially with the mean length of stem damage, and increased linearly with measured snow depth (). Beyond 4 cm of stem damage, most stems bore no fruits.
Figure 2. The relationship between snow depth and the proportion of damaged stems within 625 cm2 quadrats established at each sampling point at Saint-Méthode in 2015.
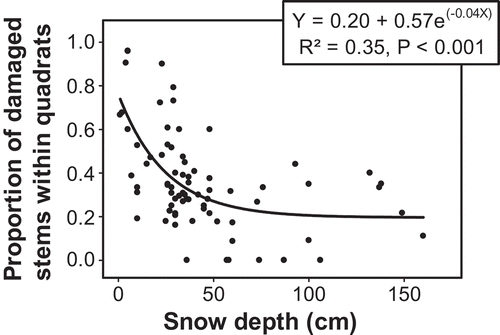
Figure 3. The relationship between snow depth and (a) the mean length of stem damage, and (b) the damaged-to-total stem length ratio of 15 randomly chosen stems within a 1 m radius around each sampling point at Saint-Méthode in 2015.
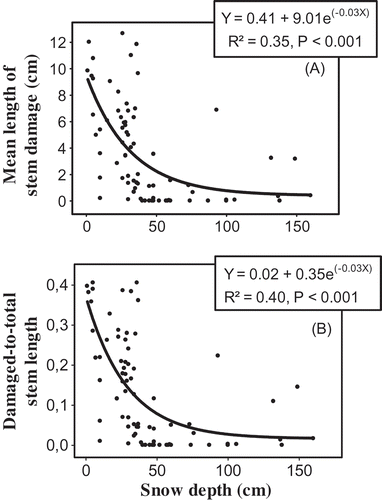
Figure 4. The relationship between fruits per stem and (a) mean length of damaged stems, and (b) snow depth at Saint-Méthode in 2015.
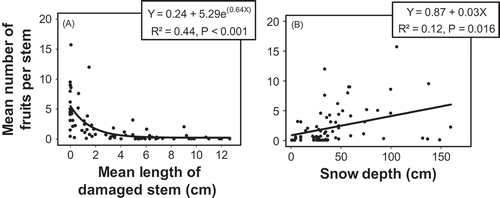
At Saint-Honoré, lowbush blueberry plants were shorter than at Saint-Méthode () while the snow cover was deeper (data not shown). Symptoms of frost damage were nearly imperceptible in summer 2015 and we found no relationship between the measured snow depth and yields.
Discussion
Both farms have a relatively flat topography such that patterns in snow depth distribution across transects likely reflect a redistribution of snow due to windbreaks rather than topographic variations. Accordingly, we conclude that many of the sampled windbreaks clearly do not achieve their intended effects on snowpack distribution. Instead of observing monotonically decreasing snow depth on the leeward side of each windbreak, we found large deviations (high amplitude) in snow depth along most transects. For example, in 2015 the mid-winter snow depth at the Saint-Méthode site varied between 1 and 160 cm (). Typically, snow accumulated close to (i.e. within 20 m) and on either side of the windbreaks, while the lowest snow depths occurred near the centre of each transect. In order to assess the net value of these windbreaks, we need to calculate the proportion of each transect where snow depth is greater than it would be if trees were absent. As no nearby fields without trees (i.e. control plots) were available for our study, we rely on data from the Roberval weather station located 25 km away. They reported that open-field snow depths varied from 16 to 21 cm during the entire month of February 2015 Environnement Canada (Citation2015). In comparison, about 30% of the total transect distance shown in is near or below these open-field snow-depth values. Blueberry producers in the SLSJ region should therefore be concerned about the efficiency of their windbreaks and, if necessary, should be seeking ways to improve them.
The snowpack distribution pattern at Saint-Méthode provides us with a few clues as to how to improve the performance of these windbreaks. For example, while the total snowpack accumulation in 2015 was higher on the windward side of transects (i.e. leeward side of windbreaks), so was the amplitude in snow depth. This suggests that these windbreaks are not sufficiently porous and thus create turbulent wakes on their leeward side (Fortin et al., Citation2013; Wilson, Citation1987). As previously mentioned, snowpack distribution may be improved by optimizing windbreak porosity. Total porosity may be, however, an imprecise metric, as the vertical and horizontal distribution of gaps, as well as gap-size distribution, also affect turbulence and snow drift (Brandle et al., Citation2004; Cleugh et al., Citation2002). The height, width and orientation of windbreaks are also to be considered (Brandle and Finch, Citation1991; Brandle et al., Citation2004), but optimizing these parameters depends on site specific variables such the prevailing wind intensity and the upwind distance of open terrain (Pomeroy and Brun, Citation2001). There is also a strong case to be made for shortening the distances between windbreaks at Saint-Méthode, as the shallowest snow was consistently found towards the middle of each transect. Typically, authors have recommended a tree row spacing of 10–15 times the height of trees for a uniform snow cover (Brandle and Hodges, Citation2000; Fortin et al., Citation2013), which at Saint-Méthode would translate into tree rows spacing of 90–135 m. Obviously, reducing the spacing between tree rows could reduce yields through the loss of productive surface area and by increased shading. Hence, there is no quick fix solution as to the best way to improve the design of these windbreaks, which should incite blueberry producers in the SLSJ region to engage in participatory research and outreach activities. The good news is that snowpack distributions in 2015 and 2016 were correlated on half the number of transects, which suggests that some farmers could assess the efficiency of their altered windbreaks by sampling snow depth distribution only once in mid-winter.
Inadequate snow cover results in the exposure of blueberry stems to cold temperature, desiccating winds and freeze-thaw cycles. This in turn may result in floral bud mortality and a decrease in extracellular resistance of stem vascular tissues (Olson and Eaton, Citation2001; Percival et al., Citation2002). To our knowledge, our study is the first to report snowpack depth across natural windbreaks, and then to correlate this snowpack distribution to lowbush blueberry yields. For example, Percival et al. (Citation2002) reported decreasing blueberry yields with increasing distance from windbreaks, but they did not actually measure snowpack depth. Lemieux et al. (Citation1995) conducted a study similar to ours, but they tested the efficiency of 1.25-m-high snow fences rather than natural windbreaks. For their part, Wildung and Sargent (Citation1989) did not experiment with windbreaks but experimentally manipulated mid-winter snow depth in a Minnesota blueberry field; they concluded that 30 cm was a critical depth for preventing frost damage. Accordingly, our study found a sharp decrease in frost damage at Saint-Méthode when snow depth was greater than 30–40 cm. We note with concern, however, that about one third of our sampled points at Saint-Méthode in 2015 had a snow depth lower than 30 cm, and almost all of these stems bore two berries or less. By comparison, Percival et al. (Citation2002) reported an average of nine berries per stem when snow completely covered blueberry stems for the entire months of January and February. If a comparable number of berries could have been produced at Saint-Méthode by simply adding twice the number of windbreaks, there might well have been a net gain in total yield in 2015, in spite of a potential loss in productive surface area due to extra windbreaks.
It may be argued that the significant relationship between snow depth and blueberry yield at Saint-Méthode in 2015 was a spurious correlation. For example, lowbush blueberry yield is significantly related to the number of pollinators in springtime (Eaton and Murray, Citation1997); moreover, it has been speculated that pollinator densities increase with the presence of windbreaks (Read et al., Citation1996). This is supported by data showing a decrease in the abundance and diversity of pollinators in blueberry fields with increasing distance from a forest’s edge (Moisan-DeSerres et al., Citation2014). There may thus be a higher pollination rate nearer to the windbreaks where the snow, at Saint-Méthode, was coincidentally deeper. However in their study, Moisan-DeSerres et al. (Citation2014) found that pollinators were distributed homogeneously in blueberry fields with natural windbreaks, at least within the first 60 m of the windbreaks. On the other hand, the largest fluctuations in snow depth at Saint-Méthode specifically occurred within 60 m margins around the windbreaks, providing strong evidence that snow depth rather than pollinator densities controlled blueberry yields in 2015.
Among other confounding factors, the proximity to windbreaks may create favourable microclimate conditions during the growing season that could bolster yields. For example, windbreaks may lower evapotranspiration and heat stress as well as reduce wind speed and mechanical stress on the stems (Cleugh, Citation1998; Kort, Citation1988; Percival et al., Citation2002). In spite of this, the evidence that snow depth is a major factor contributing to loss of yields in the SLSJ region is compelling. Besides the relationships between snow depth, frost damages and yields that we have shown at Saint-Méthode for 2015, there is other evidence corroborating our claim. For example, there was hardly any sign of frost damage at Saint-Honoré in 2015, which is consistent with an adequate snowpack resulting from shorter stems and deeper snow at that site. Likewise, the fact that record yields were harvested at both sites in 2016 is consistent with the fact that winter temperatures were much milder and the snowpack much deeper in 2016 than in the previous year Environnement Canada (Citation2015).
Our study serves as a timely call for improving winter windbreak design in the SLSJ region, in light of current trends in climate change. For example, Brown and Robinson (Citation2011) reported that spring snow cover in the Northern Hemisphere has undergone significant reductions over the past century due to warmer temperatures. Furthermore, this trend has accelerated over recent decades, corresponding to a 7% and 11% decrease in snow cover during March and April respectively, in comparison to pre-1970 values. A deeper snowpack will not only protect lowbush blueberry from winter frost damage, but will also delay the onset of snow-free days and potential damage to flowering buds in early spring.
Acknowledgements
We thank Club Conseil Bleuet for their technical support, as well as Les Entreprises Gérard Doucet Ltée and La Régie de l’Aéroport Dolbeau-Mistassini for providing access to our research sites. The project was funded by Fonds de Recherche Axé sur l’Agriculture Nordique (FRAN-02), Fonds de Recherche du Québec – Nature et Technologies (FRQNT), AgroPhytoSciences – NSERC CREATE Program, University of Sherbrooke – Faculty of Sciences, and by an NSERC Discovery Grant awarded to R. Bradley.
Additional information
Funding
References
- Brandle, J.R., and S. Finch 1991. How windbreaks work. University of Nebraska-Lincoln, Extension Paper 121.
- Brandle, J.R., and L. Hodges 2000. Field windbreaks. University of Nebraska-Lincoln, Extension Paper 846.
- Brandle, J.R., L. Hodges, J. Tyndall, and R.A. Sudmeyer. 2009. Windbreak practices. in North American agroforestry: an integrated science and practice. Edited by, Garrett H.E. America Society of Agronomy (Inc.), Madison, WI, U.S.A. 75–103.
- Brandle, J.R., L. Hodges, and X.H. Zhou 2004. Windbreaks in North American agricultural systems. University of Nebraska-Lincoln, Faculty of Agronomy & Horticulture Publications, Paper 389.
- Brown, R.D., and D.A. Robinson. 2011. Northern hemisphere spring snow cover variability and change over 1922–2010 including an assessment of uncertainty. Cryosphere 5:219–229.
- Cappiello, P.E., and S.W. Dunham. 1994. Seasonal variation in low-temperature tolerance of vaccinium angustifolium ait. HortScience 29:302–304.
- Cleugh, H.A. 1998. Effects of windbreaks on airflow, microclimates and crop yields. Agrofor. Syst. 41:55–84. doi: 10.1023/A:1006019805109.
- Cleugh, H.A., R. Prinsley, R.P. Bird, S.J. Brooks, P.S. Carberry, M.C. Crawford, T.T. Jackson, H. Meinke, S.J. Mylius, I.K. Nuberg, et al. 2002. The Australian National windbreaks program: overview and summary of results. Aust. J. Exp. Agr. 42:649–664. doi: 10.1071/EA02003.
- Current, D., J. Sackett, G. Wyatt, and D. Zamora 2012. Selecting trees and Shrubs in Windbreaks. University of Minnesota Extension. Available from https://www.extension.umn.edu/environment/agroforestry/selecting-trees-shrubs-windbreaks/docs/windbreaksrevised_1.pdf
- Eaton, L.J., and J.E. Murray. 1997. Relationships of pollinator numbers in blueberry fields to fruit development and yields. Acta Hort. 446:181–188. doi: 10.17660/ActaHortic.1997.446.27.
- Environnement Canada. 2015. 2016. past weather and climate – historical data. Ottawa, Canada: Government of Canada.
- Fortin, R., G. Grenon, G. Savard, J. Savard, and L. Tremblay 2013. Snow management using windbreaks. Government of New Brunswick, wild blueberry fact sheet A.4.1. Available from http://www2.gnb.ca/content/dam/gnb/Departments/10/pdf/Agriculture/WildBlueberries-BleuetsSauvages/SnowManagementWindbreaks.pdf
- Gagnon, S., G. Chiasson, G. Gilbert, and L. Tremblay 2016. La production du bleuet sauvage dans une perspective de développement durable Centre de référence en agriculture et agroalimentaire du Québec (CRAAQ) at. Available from https://www.craaq.qc.ca/Publications-du-CRAAQ/guide-de-production-du-bleuet-sauvage/p/PAUT0108-PDF.
- Guyot. 1977. Les effets aérodynamiques des brise-vent. Proclim E 8:157–188.
- Hiemstra, C.A., and G.E. Liston. 2014. Snow and vegetation interaction, p. 1067–1070. In: Singh V.P., Singh P., and Haritashya U.K., (Edited by). Encyclopedia of snow, ice and glaciers. Springer Publishers, Dordrecht, The Netherlands.
- Kort, J. 1988. Benefits of windbreaks to fields and forages crops. Agr. Ecosyst. Environ. 22/23:165–190. doi: 10.1016/0167-8809(88)90017-5.
- Lemieux, G.H., G. Vachon, M. Lebeuf, C. Brissson, and Y. Tremblay. 1995. Évaluation et expérimentation des mesures de protection contre le gel hivernal dans les bleuetières de la sagamie. équipe de recherche sur les bleuetières de la sagamie. rapport final, projet de recherche. Universit#xE9; du Québec à Chicoutimi. Saguenay, Québec, Canada. SE-18860235–048.
- MAPAQ. 2016. Monographie de l’industrie du bleuet sauvage au québec. Ministère de l’Agriculture, des Pêcheries et de l’Alimentation du Québec. ISBN: 978-2-550-75899-0.
- Microsoft® Excel®. 2013. Microsoft office professional plus. Microsoft Corporation, Redmond, WA, E.U.S.
- Moisan-DeSerres, J., M. Chagon, and V. Fournier. 2014. Influence of windbreaks and forest borders on abundance and species richness of native pollinators in lowbush blueberry fields in québec, Canada. Can. Entom. 147:432–442. doi: 10.4039/tce.2014.55.
- Moola, F.M., and A.U. Mallik. 1998. Morphological plasticity and regeneration strategies of velvet leaf blueberry (vaccinium myrtilloides michx.) following canopy disturbance in boreal mixedwood forests. For. Ecol. Manag. 111:35–50. doi: 10.1016/S0378-1127(98)00306-5.
- Olson, A.R., and L.J. Eaton. 2001. Spring frost damage to placental tissues in lowbush blueberry flower buds can. J. Plant Sci. 81:779–781.
- Percival, D., L.J. Eaton, D.E. Stevens, and J.P. Privé. 2002. Use of windbreaks in lowbush blueberry (vaccinium angustifolium ait.) production. Acta Hort. 574:309–316. doi: 10.17660/ActaHortic.2002.574.46.
- Perri, P.L., and M. Bloomberg. 2002. Windbreaks in southern patagonia, argentina: a review of research on growth models, windspeed reduction, and effects on crops. Agrofor. Syst. 56:129–144. doi: 10.1023/A:1021314927209.
- Pomeroy, J.W., and E. Brun. 2001. Physical properties of snow, p. 45–126. In: Jones H.G., Pomeroy J.W., Walker D.A., and Hoham R.W., (Edited by). Snow ecology: an interdisciplinary examination of snow-covered ecosystems. Cambridge University Press, Cambridge, U.K.
- R Development Core Team. 2014. R: A language and environment for statistical computing. R Foundation for Statistical Computing, Vienna, Austria. Available from http://www.R-project.org/.
- Read, G., J. Argall, and G. Chiasson 1996. Windbreak technology for wild blueberry fields. New Brunswick Department of Agriculture and Aquaculture, Fact Sheet A.4.0. Available from http://www2.gnb.ca/content/gnb/en/departments/10/agriculture/content/crops/wild_blueberries/windbreak_technology.html
- Rouse, R.J., and L. Hodges 2004. Windbreaks. University of Nebraska-Lincoln, Faculty of Agronomy & Horticulture Publications, Paper 427.
- Schneider, C.A., W.S. Rasband, and K.W. Eliceiri. 2012. NIH Image to ImageJ: 25 years of image analysis. Nature Meth. 9:671–675. doi: 10.1038/nmeth.2089.
- Thiffault, N., P. Grondin, J. Noël, and V. Poirier. 2015. Ecological gradients driving the distribution of four ericaceae in boreal Quebec, Canada. Ecol. Evol. 5:1837–1853. doi: 10.1002/ece3.2015.5.issue-9.
- Vézina, A. 2001. Les haies brise-vent. Ordre des ingénieurs forestiers du Québec, Éducation continue, Cours no. 19. Available from https://www.agrireseau.net/agroenvironnement/documents/Haies%20brise%20vent_OIFQ.pdf
- Wildung, D.K., and K. Sargent. 1989. The effect of snow depth on winter survival and productivity of Minnesota blueberries. Acta Hort. 241:232–237. doi: 10.17660/ActaHortic.1989.241.37.
- Wilson, J.D. 1987. On the choice of a windbreak porosity profile. Bound. Layer Meteor. 38:37–49. doi: 10.1007/BF00121553.
- Yarborough, D.E. 1999. Flower primordia development stage with temperature tolerance using irrigation systems for frost protection. University of Maine Cooperative Extension. Fact Sheet No. 216, U. Maine Extension No. 2003. Available from https://extension.umaine.edu/blueberries/factsheets/irrigation/flower-primordia-development-stage/