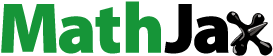
ABSTRACT
The application of nano-iron chelate and iron chelate was evaluated in this study. The treatments were applied in mid-spring and mid-summer. The experiment was conducted using a completely randomized block design with three replications during 2013–2014. The results showed that applying two times spraying of nano-iron chelate 9% (2000 mg/L) can significantly increase leaf area, chlorophyll content, along with greater catalase enzyme activity. foliar application of two times spraying of nano-iron, 9% (2000 mg/L) were observed to cause increases in, total soluble solids (TSS), ascorbic acid and total phenol contents at the time of harvest. One time foliar spraying of nano-iron, 9% (2000 mg/L) and one time soil application of nano-iron chelate, 9% increased fruit firmness. Iron and calcium nutrients inside the leaves and fruits increased in amount as a result of applying two times spraying of nano-iron, 9% (2000 mg/L). We concluded that the foliar application of nano-iron chelate is more effective than iron-chelate in treating chlorosis and in enhancing the fruit quality of quince.
KEYWORDS:
Introduction
Quince (Cydonia oblonga Mill.) belongs to the Rosaceae family and is known to have originated from Persia, Turkey, Caucasus and Central Asia. It has been cultivated since ancient times, and current records have dated its use to at least 4000 years B.C. by Asiatic people (Zielinski, Citation1997). There are approximately 106000 acres of quince throughout the world today. Turkey is the leading producer of quince fruit, as it hosts 26500 acres of land dedicated to quince trees (FAO, Citation2015).
Plant nutrition and the different types of soil are two of the most important factors in the plant production. Quince trees are very susceptible to lime-induced chlorosis. This disorder can also occur when quince is grown on calcareous soils, whereby symptoms of yellowing appear in young leaves during the growing season. Such cases are especially reported during the summer months. Quince trees generally have a low ability to take up iron from the soil (Cinelli et al., Citation2004). This problem is not only important for the quince orchard but is also a limiting factor in the cultivation of pear when grafted onto quince (Kobelus et al., Citation2007). Iron is one of the most important micronutrients required for several physiological processes in plants, including photosynthesis and respiration. In Iran, growing the quince in areas with high pH levels usually limits the availability of Fe to the trees, thereby causing leaf chlorosis and reducing fruit size and quality at harvest time. Foliar Fe application can result in greater amounts of leaf chlorophyll, although there are variations in the degrees of re-greening in response to various Fe-compounds (Kalbasi et al., Citation1986). Organic sources of Fe (Fe-chelate) and mineral sources (FeSO4. 7 H2O) can be used for alleviating Fe-deficiency in fruit trees (Martens and Westermann, Citation1991). The possibility to deliver small amounts of Fe to fruit trees via foliar sprays could be a cheaper method to overcome Fe deficiency in fruit crops, although variable responses to Fe sprays have been reported (Fernandez and Elbert, Citation2005).
The chloroplasts, along with photosystem I and II, are major sites for the production of O2 and O2−, superoxide radicals, OH, hydroxyl radicals and ROS, alkoxy radicals (Gill and Tuteja, Citation2010). Also, an induced-ROS accumulation is countered by enzymatic antioxidants such as peroxidase (PO) and catalase (CAT). Peroxidase enzyme is able to decompose H2O2 by oxidation of co-substrate, whereas catalase can break down H2O2 into water and molecular oxygen (Mittler, Citation2002). The activities of these enzymes are known to increase in response to higher concentrations of iron in plants (Marschner, Citation2012)
Nanotechnology is increasingly becoming a common term in horticultural research. Nano-iron chelate is a form of iron that is absorbable by plants. It contains 9% Iron and is stable at pH values ranging from 3 to 11. In general, nanotechnology reduces the rate at which nutritional elements are wasted and protects natural resources (Chinnamutha and Boopathi, Citation2009). Nanotechnology can involve using nano-scale fertilizer particles which physiologically provide new methods for alleviating nutritional problems in fruit trees. In spray applications of nano-iron oxide particles on soybean, the yield was observed to increase at harvest (Sheykhbaglou et al., Citation2010). Prasad et al. (Citation2012) reported that peanut seeds can be soaked in nano-zinc oxide (Zno) and in ZnSo4 solutions, the seedlings of which showed better stem and root growth, along with higher pod yield. In this regard, treating the seedlings with Zno led to better results in comparison with the application of ZnO4.
The purpose of this study was to demonstrate how the application of nano-Fe chelate and Fe-chelate to soil, and as foliar application, can alleviate chlorosis in leaves, besides improving fruit quality and enhancing the activity of antioxidant enzymes in quince fruits under field conditions.
Materials and Methods
Plant Material and Growth Conditions
The experiments were conducted from 2013 to 2014 in a commercial orchard in Hassan Abad, Neyriz, Fars province, Iran (longitude: 54° 27׳, latitude: 29° 16׳; 2034 m above sea level). The quince trees were 5 years old (Cydonia oblonga Mill. cv Esfahan) and had been grafted onto local quince rootstocks. The quince trees were planted with a spacing of 4 × 6 m and were trained as open center. The physical properties of the soil (0–60 cm) were measured ().
Table 1. Some physiochemical properties of the soil.
Experimental Design and Sampling
The experiment comprised 11 treatments and was replicated 3 times. 33 uniform trees were selected each tree was considered as a replicate. The treatments were as follows:
Control (C);
One time foliar spraying of Fe-EDDHA, 6% (Grow More, CA. USA) (1500 mg/L) (FS11T);
Two times foliar spraying of Fe-EDDHA, 6% (1500 mg/L) (FS12T);
One time spraying of Fe-EDDHA, 6% (3000 mg/L) (FS21T);
Two times foliar spraying of Fe-EDDHA, 6% (3000 mg/L) (FS22T);
One time foliar spraying of nano-iron, 9% (Khazra Co., Tehran, Iran) (1000 mg/L) (FN11T);
Two times spraying of nano-iron, 9% (1000 mg/L) (FN12T);
One time foliar spraying of nano-iron, 9% (2000 mg/L) (FN21T);
Two times spraying of nano-iron, 9% (2000 mg/L) (FN22T);
One time soil application of Fe-EDDHA, 6% (50 gram per each tree) (SS1T);
One time soil application of nano-iron chelate, 9% (33.33 gram per each tree) (SN1T). Where
F: foliar spraying (three liters per tree); S: soil application
Fe-EDDHA and nano-iron chelate were dissolved in five liters of water and applied to the soil below the drip irrigation system, and almost 20 cm deep to avoid photodecomposition of chelates.); S1 and S2: first and second concentrations of Fe-EDDHA, 6%; N1 and N2: first and second concentrations of nano-iron chelate, 9%; 1T and 2T: once and twice. Treatments were applied early in the morning. The first application of each treatment was carried out 4 weeks after full bloom (May 15th) and the second one was 16 weeks after full bloom (August 1) during 2013 and 2014 seasons.
At the end of the growth season, ten mature leaves were dissected from the middle of the fruiting branches for analysis. Additionally, ten fruits were dissected at the stage of physiological maturity (i.e. when the skin color of fruits turned light-yellow).
Measurements of Plant Leaves
Leaf Area Measurement
The leaf area was measured (Delta-T-Devices LTD England) in ten leaves that had been collected from different sides of each tree for each replication. The results were described as a mean value of ten leaves.
Chlorophyll and Carotenoid Analysis
Chlorophyll and carotenoid contents were measured according to the methods of Arnon (Citation1949). Chlorophyll contents were determined by a spectrophotometer (Shimadzu UV-1700) at wavelengths of 645 and 663 nm for chlorophylls and at 480 nm for carotenoid compounds. Chlorophyll and carotenoid concentrations were calculated by Arnon equations (Citation1949):
Enzyme Activity
Catalase Assay
The fresh leaf tissue (0.5 g) was homogenized in 5 mL phosphate buffer (50 mM), pH 7.0, containing 1% insoluble polyvinylpyrrolidone. The homogenate was centrifuged at 15 000 g for 10 min and the supernatant was used as enzyme extract. All steps in the preparation of the enzyme extract were carried out at 0–4°C. An aliquot of the extract was used for determining its protein content. Catalase was assayed by measuring the initial rate of disappearance of hydrogen peroxide by the method of Chance and Maehly (Citation1955). The 3 mL reaction mixture contained 50 mM phosphate buffer, pH 7.0, 15 mM hydrogen peroxide, and 25 µL enzyme extract. The decrease in the amount of hydrogen-peroxide was followed by a decline in A240 using Shimadzu UV-1700 spectrophotometer. The activity was expressed in units where one unit of catalase activity is defined as the amount of 0.01 at 240 nm after one minute of incubation. Enzymatic activity was expressed as enzyme units per gram of fresh weight (U g−1 FW).
Peroxidase Assay (POD)
The activity of POD was assayed following the procedure outlined by Tuna et al. (Citation2008) with slight modifications. An amount of 100 μL plant extract was added to 3 mL of the assay solution which was comprised of 3 mL of the reaction mixture containing 13 mm L−1guaiacol, 5 mmol L−1H2O2, and 50 mm L−1Na-phosphate buffer (pH 6.5). An increase in absorbance at 470 nm for 3 min at 25°C was recorded on a UV- spectrophotometer (Shimadzu UV-1700). One unit of POD activity is defined as the amount of 0.01 at 470 nm after one minute of incubation (Molassiotis et al., Citation2006). Enzymatic activity was expressed as enzyme units per gram of fresh weight (U g−1 FW).
Mineral Analysis
Leaf and fruit samples were treated by standard procedures that led to the quantification of mineral elements in fruits and leaves (Abadía et al., Citation1985, Citation1989). Specifically, the guidelines of the Association of Official Analytical Chemists were followed (AOAC, Citation1990). Oven dried plant materials were ground to a fine powder. A 0.5 g mass of dry sample was ashed at 500°C for 8 h, and was then extracted with 5 mL 2N hydrochloric acid (HCl). After digestion, the volume of the sample was increased with distilled deionized water and reached 100 mL (Tandon, Citation1998). Calcium and Fe were measured by atomic absorption spectrophotometry (Varian 220, Australia).
Measurements of Fruits
Firmness
Fruit firmness was measured on four sides of each fruit, using a penetrometer fitted with an 8-mm diameter probe (Copa-Technologie S.A., Tarascon, France), results were expressed as mean values of the four sides.
Total Soluble Solids Content (TSS)
Peeled slices of samples (5 g) were pooled and juiced together to obtain a composite sample which was then analyzed for total soluble solids (TSS) using a digital refractometer (Atago Model PR-1, Tokyo).
Ascorbic Acid
Ascorbic acid content was determined by the photometric adaptation of the 2, 6 dichlorophenol indophenol method (Klein and Perry, Citation1982). An aliquot of 100 µL of quince extract was diluted to a volume of 10 mL via 2% metaphosphoric acid and was vortexed for 30s. Then, 1 mL of the mixture was sampled and diluted with 9 mL of indophenol. After the vortex, the ascorbic acid content was measured at 515 nm using a spectrophotometer (Shimadzu UV 240, Cambridge, USA).
Total Phenolics
Total phenolics were determined using the Folin-Ciocalteu reagent as described by Velioglu et al. (Citation1998) with some modifications. Accordingly, 200 mg of the sample was extracted for 2 hours with the help of 2 mL methanol (80%) at room temperature on an orbital shaker set at 200 rpm. The mixture was centrifuged at 1000 g for 15 min and the pellet was considered as extract. Then, 100 µL of extract was mixed with 1.5 mL of Folin- Ciocalteu reagent (which had been previously diluted ten times with distilled water). This solution was allowed to stand at 22°C for 5 min, followed by the addition of 1.5 mL sodium bicarbonate solution (20%) to the mixture. After 90 min, the absorbance was measured at 750 nm (Shimadzu UV 240, Cambridge, USA).
Statistical Analysis
The experimental design was a randomized complete block design with three replications or blocks. Each block was comprised of 11 treatments. The analysis of variance was performed using the SAS software package (9), and mean values were compared by Duncan’s Multiple Range Test (DMRT). Variations of significance existed among the differences between the mean values (P < .05).
Results and Discussion
Leaf Area
Due to the similarities between the results of the two years of experiments (i.e. no significant differences existed between the two years), leaf area is reported as the mean values of both years. The effects of foliar and soil application on leaf area differed depending on the type of treatment, i.e. either nano-iron chelate or iron-chelate (). As the concentration of iron increased, the leaf area grew broader. Carrying out twice the foliar application of nano-iron chelate at 2000 mg L−1 (FN22T) led to the greatest leaf area (9.18 cm2), whereas the lowest leaf area was observed in the control (6.60 cm2) (). Leaf area is one of the most important factors that affect photosynthesis (Repková et al., Citation2009). Our results in this regard accorded closely with those report by Incesu et al. (Citation2015) in which plants grown without Fe produced the lowest amount of leaf area.
Table 2. Effect of different iron treatments on leaf area, carotenoid and chlorophyll in quince tree.
Chlorophyll and Carotenoid Contents
Chlorophyll and carotenoid contents were reported as mean values of both years. Treatment with Fe-containing solutions always resulted in the increase of leaf chlorophyll (Chl). The chlorophyll content was significantly dependent on the Fe sources. As the concentration of iron increased, chlorotic symptoms decreased in the leaves of quince trees. In general, the foliar spray of nano-iron chelate resulted in the greatest total chlorophyll content of leaves (). In both years of the study, the foliar spray of nano-iron chelate at 2000 mg L−1 and soil application of Fe-EDDHA (6%) significantly increased the total amount of chlorophyll (). The current study was consistent with previous research on nano-iron compounds. Ghafari and Razmjoo (Citation2013) reported that nano-iron oxide can be more effective than other sources of iron fertilizer. The results suggested that foliar application of nano-iron chelate is more effective than Fe-EDDHA in alleviating Fe deficiency among quince trees. According to the mentioned report, the decrease in particle size can result in higher numbers of particles per unit of weight and per specific surface area. These alterations contribute to the increase in contact area between the particles of fertilizers and the leaf surface, thereby increasing Fe uptake to a greater extent (Liscano et al., Citation2000). The reduction of chlorophyll in leaves of quince trees may be due to the dilution of leaf chlorophyll caused by growth and the inability to produce and/or stabilize new chlorophyll molecules in the thylakoid membrane (Belkhedja et al., Citation1998).
The carotenoid content of leaves was significantly affected by iron concentration and its type of source (). The foliar application of nano-iron chelate at 2000 mg l−1 significantly increased the carotenoid content in leaves (0.382 mg g−1 FW) in comparison with the control (0.226 mg g−1FW). Both nano-iron chelate and iron-chelate produced the highest amount of carotenoid content in the leaves, but nano-iron chelate appeared to be more effective than iron-chelate in this study. In a previous research, the same results were obtained regarding the production of carotenoid in wheat when comparing the efficiency of nano-iron oxide with that of iron-sulfate (Ghafari and Razmjoo, Citation2013). Generally, carotenoids act as mechanisms of antioxidant defense that protect plants against oxidative stress damage via ROS scavenging (Gill and Tuteja, Citation2010).
Enzyme Activities
Catalase and Peroxidase
The activity of catalase (CAT) and proxidase (POD) were affected by iron sources. As shown in , an increase in CAT and POD activities resulted from the application of iron. In general, the activity of CAT was significantly higher than that of POD (). The activity of CAT became higher in response to the application of nano-iron chelate. The activity was observed in weaker forms in response to Fe-chelate, however (). In both years of the study, spraying twice the nano-iron chelate at 2000 mg L−1 (FN22T) on quince leaves significantly increased the CAT activity (27.47 Ug−1 FW) in comparison with the control (20.53 Ug−1 FW). The activity of CAT can be regarded as an index of the nutritional status of Fe in plants (Marschner, Citation2012). The same results were obtained regarding POD activity. Peroxidase enzyme activity was affected by the type of Fe source. Applying the nano-iron chelate twice on quince leaves significantly increased the POD activity (4.20 Ug−1 FW). A previous study indicated that enzymatic activities of two Fe-containing enzymes in the root and leaf, i.e. CAT and POD, were significantly affected by Fe-deficiency in tolerant grape genotypes, either through direct or induced bicarbonate (Ksouri et al., Citation2006). Similar results were reported in relation to the decrease in CAT and POD activities, due to Fe-deficiency in GF677 (Molassiotis et al., Citation2006).
Table 3. Effect of different iron treatments on Fe content in leaf and fruit, leaf catalase and peroxidase enzyme activities of quince tree.
Fe Content of Leaf and Fruit
In evaluating the Fe contents in the leaf, the effect of year and treatment were significant, but their interactions were not significant (data not shown). In both years of the study, applying twice the foliar application of nano-iron chelate at 2000 mg L−1(FN22T) caused the Fe content in the leaf to become highest (100 and 111.4 mg kg−1 DW, respectively), whereas the lowest Fe content was observed in the control treatment of quince trees (65.26, 68.42 mg kg−1DW, respectively). Due to the similarity between the results of the two years (i.e. no significant difference existed between the years), the Fe content in fruits is herein reported as the mean values of measurements during the two years. The fruit Fe contents were significantly affected by the application of nano-iron chelate and iron chelate (). Applying twice the foliar application of nano-iron chelate at 2000 mg L−1 caused the highest content of Fe in quince fruits (19.91 mg kg−1), whereas the lowest amount of content was observed in the control treatment (11.05 mg kg−1 FW). In another context, it has been reported that woody cuttings of tolerant grape genotypes under direct Fe-deficient conditions experienced lower levels of leaf chlorosis, along with higher Fe contents in the leaf, compared to susceptible genotypes (Ksouri et al., Citation2006). The Fe (III)-reductase activity in roots is known to be strongly stimulated by direct or bicarbonate-induced Fe deficiency (Ksouri et al., Citation2006). In this study, the local quince rootstocks were very susceptible to high levels of pH in the soil which induce Fe deficiency (). Spraying the nano-iron chelate was more effective than soil application in increasing Fe contents of the leaf and fruit. Marschner (Citation2012) reported that the spraying of iron-chelate can be more effective than its application to the soil. Woody cuttings of quince rootstocks in comparison with pear rootstocks under Fe-deficient conditions have shown lower contents of Fe in the leaves and roots (Donnini et al., Citation2009). The GF677 rootstock is tolerant to Fe-deficiency and has higher potentials for transferring more iron to the leaf, as compared to the Cadaman rootstock which is susceptible to Fe (Molassiotis et al., Citation2006). The results of this study showed that spraying nano-iron chelate can be more effective than iron-chelate in increasing Fe contents in the leaves and fruits of quince trees.
Ca Contents of Leaf and Fruit
The Ca concentrations in leaves and fruits increased by applying twice the foliar treatments of nano-iron chelate 2000 mg L−1 (FN22T) and by applying once the Fe-EDDHA to the soil (50 g per tree) (SS1T) in the first year of the experiment (). In the second year, however, the highest Ca concentration was caused by the application of Fe-chelate to the soil (50 g per tree) (). The Ca content of the quince fruit became highest as a result of applying Fe-chelate to the soil, and the lowest content was observed in the control. A previous research on peach trees revealed that the Ca content of the leaf can increase from 60 to 120 DAFB in all peach trees (Belkhedja et al., Citation1998). Under conditions of Fe-deficiency, the absorption and translocation of Ca would decrease (El-Shazly and Dris, Citation2004).
Table 4. Effect of different iron treatments on Ca content in leaf and fruit and fruit firmness of quince tree.
Phenolic Content
At harvest time, the control treatment had the highest total phenol content, while the lowest content was observed as a result of applying twice the foliar treatment of nano-iron chelate at 2000 mg−1L (FN22T) and, also, by applying once the Fe-EDDHA (6%) to the soil (50 g per tree) (SS1T). These two treatments reduced the phenolic contents of quince fruits at harvest time (by 16.24 and 15.64%, respectively) in comparison with control fruits (). Fe-deficiency reduces the activity of POD in the roots of some plants and causes the accumulation of phenolic compounds in the ribosome (Marschner, Citation2012). In this study, Fe-deficient trees reduced the POD activity and increased the phenol content in fruits. Álvarez-Fernández et al. (Citation2003) reported that peach trees with moderate to severe chlorotic symptoms can bear fruits in which the total phenolic content of the mesocarp increases. The same author (Citation2011) reported higher amounts of phenolic compounds in the fruits of Fe-deficient trees (Álvarez-Fernández et al., Citation2011). High phenolic contents in the fruits can be a cause of astringency, associated with unfavorable fruit flavor (Álvarez-Fernández et al., Citation2003). Fruit maturity in Fe-deficient peach trees is known to be delayed, and higher phenolic compounds can be found in peach fruits accordingly (Álvarez-Fernández et al., Citation2003).
Table 5. Effect of different iron treatments on some fruit properties in quince tree.
Ascorbic Acid and Fruit TSS
The amounts of ascorbic acid and TSS in quince fruits were affected by Fe-deficiency (). The lowest amount of ascorbic acid in quince fruits was observed in the control, and the highest was obtained in response to applying the foliar nano-iron chelate twice at 2000
mg L−1 (43.64%). These results are consistent with previous ones reported by Álvarez-Fernández et al. (Citation2011) on peach and pear, and by Zhang et al. (Citation2014) on mandarin. Ascorbic acid acts as a soluble antioxidant to protect cells from damage when caused by ROS in plants (Khan and Ashraf, Citation2008).
In both years of the experiment, the highest TSS contents were obtained by applying nano-iron chelate twice as a foliar treatment at 2000 mg L−1 (FN22T), whereas the lowest contents were observed in fruits of the control group. This treatment increased the TSS content of fruits at harvest time by 15.41 and 18.74% in 2013 and 2014, respectively, compared with the control treatment (). The results of our study were not in agreement with previous research on peach (Álvarez-Fernández et al., Citation2003) wherein it was reported that peach trees with severe chlorotic symptoms produced fruits with higher TSS content. Our results are in agreement with Zhang et al. (Citation2014) on mandarin and with Razeto and Valdes (Citation2006) on nectarine fruits and pear (Álvarez-Fernández et al., Citation2011).
Fruit Firmness
The fruit firmness at harvest was significantly affected by the application of FS12T, FN12T and FN21T treatments (). The lowest degree of fruit firmness (6.37 kg cm2) was measured in the fruits of trees that had been treated with FS12T, which indicates a 15.85% reduction in comparison with the control treatment. The highest values of fruit firmness, i.e. 7.97 and 7.79 kg cm2, were measured in quince fruits as a result of applying the FN12T and SN1T treatments, respectively. The current results are consistent with previous research on pear by Álvarez-Fernández et al. (Citation2011). In the mentioned research, it was found that Fe-deficiency can cause a decrease in fruit firmness. On the contrary, the firmness of peach fruits were reportedly not affected by moderate symptoms of chlorosis, whereas it increased in fruits that suffered severely from Fe-deficiency (Álvarez-Fernández et al., Citation2011).
Conclusion
The chlorophyll content in the leaf increased significantly in quince trees. The application of nano-iron chelate and iron-chelate increased the leaf area significantly. The treatments were also associated with higher carotenoid content, more activities of iron-containing enzymes (i.e. CAT and POD) and higher Fe and Ca contents in the leaf and fruit. Spraying nano-iron chelate on the leaves proved to be more effective in alleviating chlorotic symptoms than when spraying iron-chelate. It is likely that nano-iron chelate can be dissolved in water more efficiently and could enable plants to use fertilizers more efficiently. The application of nano-iron chelate and iron-chelate at higher concentrations reduced the phenolic content in fruits at harvest time, whereas the amounts of ascorbic acid and TSS content increased in fruits. These findings support the hypothesis that the application of nano-iron chelate on quince trees can be seen as a superior treatment in comparison with the conventional types of iron supplements.
Acknowledgments
The authors wish to extend their thanks and appreciation to Shiraz University Research and Technology Council for their financial support.
References
- Abadía, A., M. Sanz, J. De Las Rivas, and J. Abadía. 1989. Photosynthetic pigments and mineral composition of iron deficient pear leaves. J. Plant. Nutr. 12:827–838. doi: 10.1080/01904168909363996.
- Abadía, J., J.N. Nishio, E. Monge, L. Montañés, and L. Heras. 1985. Mineral composition of peach leaves affected by iron chlorosis. J. Plant Nutr. 8:965–975. doi: 10.1080/01904168509363398.
- Álvarez-Fernández, A., P. Paniagua, J. Abadía, and A. Abadía. 2003. Effects of Fe deficiency chlorosis on yield and fruit quality in peach (Prunus persica L. Batsch). J. Agric. Food Chem. 51(19):5738–5744. doi: 10.1021/jf034402c.
- Álvarez-Fernández, A., J.C. Melgar, J. Abadía, and A. Abadía. 2011. Effects of moderate and severe iron deficiency chlorosis on fruit yield, appearance and composition in pear (Pyrus communis L.) and peach (Prunus persica (L.) Batsch). Environ. Exper. Bot. 71(2):280–286. doi: 10.1016/j.envexpbot.2010.12.012.
- AOAC. 1990. Official methods of analysis of the association of official analytical chemists. Ed. K Helrich, Washington, DC.
- Arnon, D. 1949. Copper enzyme in isolated chloroplasts. Polyphenol Oxidase in Beta vulgaris. Plant Physiol. 24:1–15. doi: 10.1104/pp.24.1.1.
- Belkhedja, R., F. Morales, M. Sanz, A. Abadía, and J. Abadía. 1998. Iron deficiency in peach trees: Effects on leaf chlorophyll and nutrient concentrations in flowers and leaves. Plant Soil 203(2):257–268. doi: 10.1023/A:1004373202458.
- Chance, B., and A. Maehly. 1955. Assay of catalase and peroxidase. Meth. Enzymol. 2:764–775.
- Chinnamutha, C.R., and P.M. Boopathi. 2009. Nanotechnology and agro ecosystem. Madras Agric. J. 96:17–31.
- Cinelli, F., F. Loreti, and R. Muleo. 2004. Regeneration and selection of quince BA29 (Cydonia Oblonga Mill.) somaclones tolerant to lime-induced chlorosis. Acta Hortic. 658:573–579.
- Donnini, S., A. Castagna, A. Ranieri, and G. Zocchi. 2009. Differential responses in pear and quince genotypes induced by Fe deficiency and bicarbonate. J. Plant. Physiol. 166(11):1181–1193. doi: 10.1016/j.jplph.2009.01.007.
- El-Shazly, S.M., and R. Dris. 2004. Response of’Anna’apple trees to foliar sprays of chelated iron, manganese and zinc. Inter. J. Food Agric. Environ. 2(34):126–130.
- FAO, F. 2015. Food and agriculture organization. <http://faostat.fao.org/site/291/default.aspx>.
- Fernandez, V., and G. Elbert. 2005. Foliar application fertilization a critical review. J. Plant. Nutr. 28:2113–2124. doi: 10.1080/01904160500320954.
- Ghafari, H., and J. Razmjoo. 2013. Effect of foliar application of Nano-Iron Oxidase. Iron chelate and Iron Sulphate rates on yield quality of wheat. Inter. J. Plant Prod. 4:2997–3003.
- Gill, S.S., and N. Tuteja. 2010. Reactive oxygen species and antioxidant machinery in abiotic stress tolerance in crop plants. Plant Physiol. Biochem. 48(12):909–930.
- Incesu, M., T. Yeşiloğlu, B. Cimen, and B. Yilmaz. 2015. Influences of different iron levels on plant growth and photosynthesis of W. Murcott mandarin grafted on two rootstocks under high pH conditions. Turk. J. Agric. Forest 39(5):838–844. doi: 10.3906/tar-1501-25.
- Kalbasi, M., N. Manuchehri, and F. Fisoofi. 1986. Local acidification of soil as a means to alleviate iron chlorosis in quince orchards. J. Plant Nutr. 9:1001–1007. doi: 10.1080/01904168609363500.
- Khan, A., and M. Ashraf. 2008. Exogenously applied ascorbic acid alleviates salt-induced oxidative stress in wheat. Environ. Exper. Bot. 63(1):224–231. doi: 10.1016/j.envexpbot.2007.10.018.
- Klein, B.P., and A.K. Perry. 1982. Ascorbic acid and vitamin A activity in selected vegetables from different geographical areas of the United States. J. Food Sci. 47(3):941–945. doi: 10.1111/j.1365-2621.1982.tb12750.x.
- Kobelus, V., P. Salas, and V. Reznicek. 2007. Rootstock breeding and evaluation Cydonia Oblongoas a pear rootstock and its effect onthe young plant quality of pear in the nursery. Acta Hortic. 732:233–237. doi: 10.17660/ActaHortic.2007.732.33.
- Ksouri, R., S. Mrah, M. Gharsalli, and M. Lachaal. 2006. Biochemical response to true and biocharbonate-induced iron deficiency in grapevine genotypes. J. Plant Nutr. 29:305–315. doi: 10.1080/01904160500476897.
- Liscano, J.F., C.E. Wilson, R.J. Norman-Jr, and N.A. Slaton. 2000. Zinc availability to rice from seven granular fertilizers. AAES Res. Bull. 963:1–31.
- Marschner, H. 2012. Marschner᾽s mineral nutrition of higher plants. Acadamic Press School of Agriculture, Food and Wine. The University of Adelaide, Australia.
- Martens, D.C., and D.T. Westermann. 1991. Fertilizer application for correcting micronutrient deficiencies, p. 549–592. In: J.J. Mortvedtt, F.R. Cox, L.M. Shuman, and R.M. Welch (eds.). Madison: WI, USA. 2nd ed., SSSA Book Series 4, SSSA.
- Mittler, R. 2002. Oxidative stress, antioxidants and stress tolerance. Trends Plant. Sci. 7:405–410.
- Molassiotis, A., G. Tanou, G. Diamantidis, A. Patakas, and I. Therios. 2006. Effects of 4-month Fe deficiency exposure on Fe reduction mechanism, photosynthetic gas exchange, chlorophyll fluorescence and antioxidant defense in two peach rootstocks differing in Fe deficiency tolerance. J. Plant. Physiol. 163(2):176–185. doi: 10.1016/j.jplph.2004.11.016.
- Prasad, T.N.V.K.V., P. Sudhakar, Y. Sreenivasulu, P. Latha, V. Munasway, R. Rajo, T.S. Sreeprasad, P.R. Sajanlal, and T. Pradeep. 2012. Effect of mono scale zinc oxide particle on the germination, growth and yield of peanut. J. Plant. Nutr. 35:905–927. doi: 10.1080/01904167.2012.663443.
- Razeto, B., and G. Valdes. 2006. Fruit analysis as an indicator of the iron status of nectarine and kiwi plant. HortTech. 16:579–582. doi: 10.21273/HORTTECH.16.4.0579.
- Repková, J., M. Brestič, and K. Olšovská. 2009. Leaf growth under temperature and light control. Plant. Soil. Environ. 55:551–557. doi: 10.17221/PSE.
- Sheykhbaglou, R., M. Sedghi, M. Tajbakhsh Shishevan, and R.S. Sharifi. 2010. Effects of nano-iron oxide particles on agronomic traits of soybean. Notul. Sci. Biolo. 2(2):112–113. doi: 10.15835/nsb224667.
- Tandon, H.L.S. 1998. Methods of analysis of soils, plants, waters and fertilizers. Fert. Dev. Cons. Organ, New Delhi, India.
- Tuna, A.L., C. Kaya, M. Dikilitas, and D. Higgs. 2008. The combinedeffects of gibberellic acid and salinity on some antioxidantenzyme activities, plant growth parameters and nutritionalstatus in maize plants. Environ. Exp. Bot. 62(1):1–9. doi: 10.1016/j.envexpbot.2007.06.007.
- Velioglu, Y.S., G. Mazza, L. Gao, and B.D. Oomah. 1998. Antioxidant activity and total phenolics in selected fruits, vegetables, and grain products. J. Agric. Food Chem. 46(10):4113–4117. doi: 10.1021/jf9801973.
- Zhang, Y., C.X. Hu, Q.L. Tan, C.S. Zheng, H.P. Gui, W.N. Zeng, and X.H. Zhao. 2014. Plant nutrition status, yield and quality of satsuma mandarin (Citrus unshiu Marc.) under soil application of Fe-EDDHA and combination with zinc and manganese in calcareous soil. Sci. Hortic. 174:46–53. doi: 10.1016/j.scienta.2014.05.005.
- Zielinksi, Q.B. 1997. Modern systemic pomology. Pomona Books: Canada.