ABSTRACT
Citrus is an important fruit crop in Bangladesh and its growth is often constrained by salt stress. A polyhouse experiment was conducted to assess salt tolerance of three citrus rootstocks. Six-month-old seedlings of three citrus rootstocks, namely pomelo, Cleopatra mandarin, and calamansi, were irrigated with six concentrations of NaCl (0.75, 3, 6, 9, 12 and 16 dS m–1) for 3 months. Seedlings of all citrus rootstocks were significantly affected by salinity of irrigation water. All growth parameters were decreased with increase in salinity level of irrigation water. Based on growth parameters, the seedlings of Cleopatra mandarin were the least affected followed by pomelo and calamansi. The seedlings of Cleopatra mandarin accumulated less Na and Cl, while calamansi accumulated the highest amount of Na and Cl. Chlorophyll content was decreased and proline content was increased with increase in salinity level of irrigation water. Proline content was the highest in the leaves of Cleopatra mandarin with 16 dS m–1 salinity level followed by pomelo and calamansi. Overall, Cleopatra mandarin and pomelo rootstocks were more tolerant to saline conditions than calamansi rootstock. The relative susceptibility of citrus species to salinity should be taken into consideration for plant breeding programs, and growing of salt-tolerant citrus rootstocks should be promoted in salt-affected soils for better productivity.
Introduction
Citrus belongs to the group of salt-sensitive crop (Maas, Citation1993). It is an important fruit crop in Bangladesh where the total area under this crop was about 35,000 ha and the total production was around 0.14 mt in 2011. Bangladesh is one of the most vulnerable countries to climate change, and many of the negative impacts of climate change including an increase in salinity level are already evident in many parts of the country. Both water and soil salinity are a serious problem in the coastal regions of Bangladesh. The salt-affected area in Bangladesh has increased from 8,330 km2 in 1973 to 10,560 km2 in 2009, and about 53% of the coastal areas are affected by salinity (SRDI, Citation2010). Citrus production in Bangladesh is mostly confined to the north-eastern and south-eastern regions, but the vast coastal region is still out of commercial cultivation due to salinity problem though other agro-climatic conditions are favorable. The coastal zone of Bangladesh covers 32% of the total land area of the country accounting for 30% of the total cultivable land and accommodates more than 35 million people.
Groundwater of coastal regions is often exposed to seawater with high salinity, which deteriorates its quality and restricts its suitability for irrigation purpose (Simpson et al., Citation2014). Citrus species vary in their tolerance to salinity and some citrus plants can withstand a high salt content of up to 16 dS m–1 (Grieve and Walker, Citation1983). The tolerance level of different species of citrus can be determined by their capacity to exclude the potentially toxic Na+ and Cl – ions (Khoshbakht et al., Citation2015; Moya et al., Citation2003).
Water salinity affects crops in two ways: by osmotic effects and specific ion toxicity, and both types of damage can affect citrus crops. Osmotic effects-induced salinity damage occurs when the amount of the dissolved salt in water is high enough to reduce crop growth. Indirect movement of water in the leaf tissues of citrus can cause an accumulation of Cl – ion negatively affecting transpiration rate and photosynthesis (Moya et al., Citation2003). Moreover, when Cl – concentration in the leaf tissue reaches around 1.5% (dry weight basis), it accelerates defoliation by enhancing leaf abscission and ethylene production (Arbona et al., Citation2006). Citrus species are unable to absorb salts from the soil solution and accumulate them in their tissues as a mean of adjusting osmotic pressure. Rather, they accumulate organic solutes (proline) and nontoxic inorganic ions in their cells to cope with salinity stress (Lauchli and Epstein, Citation1990). Accumulation of B, Na, and Cl ions in plant tissue either from leaf absorption and/or root uptake causes specific ion toxicities (Garcia-Sanchez et al., Citation2000). This commonly occurs in evergreen trees, where the leaves survive for longer times and transpire longer (Lauchli and Epstein, Citation1990).
Accumulation of salts in the chloroplast reduces chlorophyll content, which directly affects photosynthetic activities and yield (Abbas et al., Citation2013). However, the response of citrus to salinity depends on many factors including rootstock, rootstock–scion combination, irrigation system, soil type, and local climatic conditions. Changing one or more of these factors could help expand citrus cultivation in the problematic areas or help adapt to adverse climatic conditions. Grafting and budding with desired varieties on compatible rootstocks are an inexpensive way to obtain desired fruit crop, which could be disseminated in problematic areas (saline, submerged and drought-prone areas). It is well established that rootstock exerts profound influence on the vigor, precocity, productivity, quality of fruit and resistance or tolerance to disease, salt, adverse soil condition, nutrition, winter hardiness and finally to the longevity of scion grafted or budded on the rootstock (Gainza et al., Citation2015). Therefore, most commercial orchards of citrus are established on the grafted or budded planting materials. Different types of citrus species are being grown in Bangladesh, especially in the south-eastern hilly region. Among them, Rangpur lime (Citrus limonia Osbeck), rough lemon (Citrus jambhiri Lush.), calamansi (Fortunella japonica Thunb.), pomelo (Citrus maxima Merr.), Cleopatra mandarin (Citrus reshni Hort. ex Tan) and sweet orange (Citrus sinensis L. Osbeck) are largely used as rootstocks. Because of the dearth of studies, the response of the seedlings of three widely grown citrus rootstocks in Bangladesh (pomelo, Cleopatra mandarin, and calamansi) was evaluated under different water salinity levels in terms of their initial vegetative growth, mineral nutrient accumulation and biochemical parameters.
Materials and Methods
Plant Culture
The experiment was conducted in a polyhouse at the Bangladesh Agricultural Research Institute, Gazipur, Bangladesh in 2016. Six-month-old seedlings of pomelo, Cleopatra mandarin and calamansi were used in this study. The seedlings were raised in plastic pots (14 cm height and 15 cm diameter), which were filled with 1.8 kg of growth medium consisting of 40% well-decomposed cow dung and 60% topsoil. The growth medium contained 3.2% organic matter, 7.3 mg kg–1 available N, 98.8 mg kg–1 available P, and 693.6, 5.3 and 412.7 mg kg–1 exchangeable K, Ca, and Mg, respectively. The seedlings were sprayed with Fighter 2.5 EC (Lambda Cyhalothrin) at 2 mL L–1 water once a week for controlling citrus leafminer (Phyllocnistis citrella Stainton). No herbicide was applied and pots were hand-weeded as and when required.
Preparation and Application of Saline Water
Commercial NaCl and distilled water were purchased from the local market for preparing different concentrations of saline water. The electrical conductivity of all concentrations of saline water was measured using an electrical conductivity meter (EC meter) to verify the salinity of the respective solution was at the desired level. Normal tap water was used for the control (0.75 dS m–1), whereas 2.25, 4.50, 6.75, 9.00, and 12.00 g NaCl were dissolved in 1-L distilled water for making 3, 6, 9, 12, and 16 dS m–1 water salinity, respectively. Saline water was applied to all citrus species during the morning (8:30–10:30 AM) on the same day. Plants were irrigated twice a week with 250 mL of water applied per pot in each irrigation event without any visible leaching throughout the study period.
Growth Measurements
Data on growth parameters such as survivability percentage of seedlings (measured by counting the live seedlings), plant height and shoot diameter [measured by Electronic Digital Vernier Caliper (Model Number: EBC143, China)] were collected at 90 days after treatment. After harvest, seedlings were washed with normal water and gently rinsed by deionized water for collecting subsequent data. All leaves were removed from the stem and leaf area was measured using a leaf area meter (LI-3100C, LI-COR, Lincoln, Nebraska, USA). Immediately after leaf area measurement, a portion of fully expanded mature leaf was separated and was stored in a refrigerator for chlorophyll and proline analyses. The rest of the mature leaf was dried and stored for nutrient analysis. The seedlings were separated into root and shoot, and dry weights were measured after 72 h of drying in a forced-air oven (72°C).
Estimation of Nutrient Contents
Nitrogen was measured by the micro Kjeldahl method, while chloride was estimated by the colorimetric method. Other nutrients such as P, K, and Na were determined using an inductively coupled plasma-atomic emission spectrometer. All analyses were conducted at the Plant Breeding Laboratory of the Bangladesh Agricultural Research Institute, Gazipur.
Estimation of Chlorophyll Content
One gram of fresh leaf was sliced into small pieces and soaked in a mortar containing 20 mL acetone (80%). It was then grounded for 5 min and centrifuged at 5,000 rpm for 5 min at 4°C. After that, the supernatant was transferred to a fresh flask until the residue turned colorless. The supernatants were read in a spectrophotometer (UV-1800, Shimadzu Corporation, Kyoto, Japan) at 647 nm and 664 nm against 80% acetone blank for chlorophyll a (Chl a) and chlorophyll b (Chl b), respectively. Chl a, Chl b, and total chlorophyll (Chl t) contents were calculated based on the equations described by Wellburn (Citation1994): Chl a = 11.74 A664–2.66 A647, Chl b = 21.91 A647–4.53 A664 and chlorophyll t = Chl a + Chl b.
Determination of Proline Content
Proline content was measured at the Plant Breeding Laboratory of the Bangladesh Agricultural Research Institute, Gazipur, by following the method of Bates et al. (Citation1973). A total of 250 mg of fresh leaf sample was collected, washed in distilled water and the sample was homogenized in 10 mL sulfosalicylic acid (3%) using a mortar and pestle. The crude extract was then centrifuged at 3,000 rpm for 10 min. The supernatant (2 mL) was added into a fresh test tube to which 2 mL of 6 M orthophosphoric acid, 2 mL of acid-ninhydrin solution and 2 mL of glacial acetic acid were added. Tubes were incubated in a water bath at 100°C for 60 min. After cooling the reaction mixture, the sample was transferred in a separating funnel and 4 mL of toluene was added. It was then vortexed for 2–3 min and two layers were evident. Colorless bottom layer was removed, while the pink colored upper layer was collected and read at 520 nm in a spectrophotometer (UV-1800, Shimadzu Corporation, Kyoto, Japan). The proline concentration in the sample was determined from a standard curve using analytical grade proline and calculated on a fresh weight basis (mg proline g–1 fresh weight).
Statistical Analyses
The experiment was set up as a completely randomized design with 3 × 6 factorial combinations: 3 citrus rootstocks (pomelo, Cleopatra mandarin, and calamansi) and 6 concentrations of NaCl (0.75, 3, 6, 9, 12 and 16 dS m–1). There were three replications and each replication contained one seedling. The experiment was repeated. The data represent the mean of the two experiments, as there was no time by treatment interaction. The data were subjected to a two-way analysis of variance (ANOVA) using the F test conducted by the statistical software STAR 2.0.1 (Statistical Tool for Agricultural Research, version 2.0.1). Linear regression analysis was performed when there were significant effects.
Results
Effect of Salinity on Growth of Citrus Rootstocks
All growth parameters of citrus rootstocks in terms of survivability, plant height, shoot diameter, leaf area and dry weights of root and shoot were significantly (P < .01) influenced by the level of salinity (). The seedlings of all studied rootstocks showed significant difference in survivability after 3 months under all salinity treatments; however, the difference in survivability among three rootstocks was not significant under the control treatment. A negative linear relationship was observed between citrus rootstocks and water salinity in terms of survivability. The seedlings of Cleopatra mandarin were the least affected with the lowest slope of the regression line (1.43) and were tolerant to salinity level up to 9 dS m–1 with no significant difference compared with the control ()). The survivability of pomelo seedlings was not affected up to 3 dS m–1 water salinity. The survivability of the seedlings of calamansi was markedly influenced with an increase in NaCl concentration in irrigation water. The survivability of all the rootstocks was the lowest with the salinity level of 16 dS m–1 where Cleopatra mandarin, pomelo, and calamansi had 78%, 58% and 20% survivability, respectively. The highest slope of the regression line was evident in the seedlings of calamansi (–5.01) followed by that of pomelo (–2.71) and Cleopatra mandarin (–1.43).
Table 1. Summary of analysis of variance (ANOVA) for survivability, plant height, shoot diameter, leaf area, dry weights of root and shoot of citrus rootstocks after 90 days of irrigation with different levels of water salinity.
Figure 1. Relationship between survivability and water salinity (A) and between plant height and water salinity (B) of citrus rootstocks after 90 days of irrigation with different levels of water salinity (R = Rootstocks, S = Water salinity, ** Significant at P< .01). Error bars on both axes represent standard error.
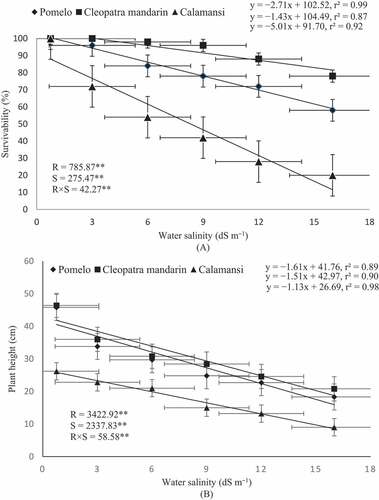
Plant height was negatively affected by an increasing salinity level as shown by a negative linear relationship between salinity level and plant height in the regression lines ()). This indicates that increasing water salinity reduced plant height in all citrus rootstocks. The lowest plant height was observed in the seedlings of calamansi (9.0 cm) with the highest water salinity (16 dS m–1). Maximum reduction in plant height was observed in the seedlings of calamansi (66%) followed by the seedlings of pomelo (60%) and Cleopatra mandarin (55%) when salinity level was increased from 0.75 to 16 dS m–1. Plant height was highly significantly (P< .01) related to the salinity level (). In the present study, 90%, 89% and 98% variation in plant height of Cleopatra mandarin (r2 = 0.90), pomelo (r2 = 0.89) and calamansi (r2 = 0.98), respectively, could be explained by the increasing level of water salinity.
There was a significant negative relationship between shoot diameter and water salinity ()). The highest shoot diameter was obtained in the seedlings of pomelo (5.0 mm) when treated with the normal water (0.75 dS m–1); however, it was reduced by 28% with the highest salinity level of 16 dS m–1. The degree of shoot diameter reduction was the least in the seedlings of Cleopatra mandarin (~19% with 16 dS m–1 water salinity). The seedlings of calamansi were more severely affected than the other two rootstocks when NaCl concentration was increased in irrigation water. Shoot diameter of calamansi was reduced by 40% when water salinity was increased from 0.75 to 16 dS m–1 ()). Similarly, the negative effect of salinity was also visible on leaf area of all citrus species, but with different intensities ()). Leaf area varied from 231.1 to 30.8 cm2 plant–1 in pomelo, 69.9 to 21.5 cm2 plant–1 in Cleopatra mandarin and 62.9 to 8.9 cm2 plant–1 in calamansi when salinity level increased from 0.75 to 16 dS m–1. The highest slope of the linear regression line was found in the seedlings of pomelo (–13.51) followed by that of calamansi (–3.45) and Cleopatra mandarin (–3.37). This indicates that leaf area of pomelo seedlings was drastically reduced with an increasing level of salinity compared with the other two rootstocks.
Figure 2. Relationship between shoot diameter and water salinity (A) and between leaf area and water salinity (B) of citrus rootstocks after 90 days of irrigation with different levels of water salinity (R = Rootstocks, S = Water salinity, ** Significant at P< .01). Error bars on both axes represent standard error.
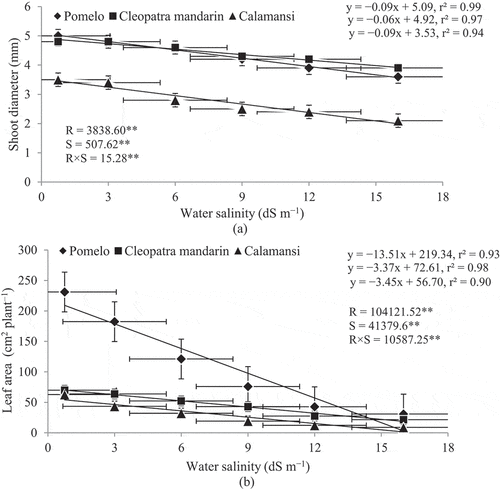
A significant negative linear relationship was evident between root and shoot dry weights of citrus rootstocks and water salinity levels (,), respectively). Root dry weight was reduced from 3.6 g (control) to 3.3, 1.4, 1.3, 0.8, and 0.7 g in pomelo due to an increase in the level of water salinity from 0.75 to 3, 6, 9, 12 and 16 dS m–1, respectively. Similar trends were also observed in the seedlings of Cleopatra mandarin and calamansi where root dry weight was reduced from 2.3 g to 0.9 g in Cleopatra mandarin and from 1.4 g to 0.2 g in calamansi when salinity level was increased from 0.75 to 16 dS m–1. Shoot dry weight was also decreased with an increase in the level of water salinity. The seedlings of calamansi had the highest reduction in shoot dry weight (93%) followed by the seedlings of pomelo (84%) and Cleopatra mandarin (75%) when salinity level was increased from 0.75 to 16 dS m–1.
Figure 3. Relationship between dry weight of root and water salinity (a) and between dry weight of shoot and water salinity (b) of citrus rootstocks after 90 days of irrigation with different levels of water salinity (R = Rootstocks, S = Water salinity, ** Significant at P< .01). Error bars on both axes represent standard error.
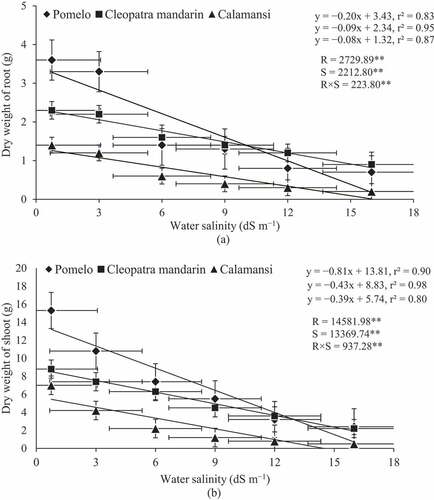
Effect of Salinity on Mineral Nutrient Accumulation
Nutrient content of different rootstocks was significantly affected by salinity levels (). A significant positive linear relationship was observed between Na+ and Cl – accumulation by citrus rootstocks and increasing salinity level (,), respectively). The amounts of Na+ and Cl – were significantly increased in the leaves of all rootstocks with an increase of NaCl in irrigation water. The lowest amount of Na+ was observed in the leaves of three rootstocks at the control (0.75 dS m–1), which was statistically similar with Na+ content at 3 dS m–1 water salinity for pomelo and calamansi, and at 9 dS m–1 water salinity for Cleopatra mandarin ()). The amount of Na+ was significantly increased in all the rootstocks when irrigated with 12 dS m–1 saline water. At this salinity level, the lowest amount of Na+ was accumulated in Cleopatra mandarin (0.71%) and the highest amount of Na+ was observed in the seedlings of calamansi (1.45%). Similarly, the seedlings of calamansi accumulated the highest amount of Na+ (1.52%) at the highest level of water salinity (16 dS m–1) followed by the seedlings of pomelo (1.20%) and Cleopatra mandarin (0.82%).
Table 2. Summary of analysis of variance (ANOVA) for Na, Cl, N, P, K, chlorophyll, and proline contents of citrus rootstocks after 90 days of irrigation with different levels of water salinity.
Figure 4. Relationship between Na content and water salinity (A) and between chloride content and water salinity (B) of citrus rootstocks after 90 days of irrigation with different levels of water salinity (R = Rootstocks, S = Water salinity, ** Significant at P< .01). Error bars on both axes represent standard error.
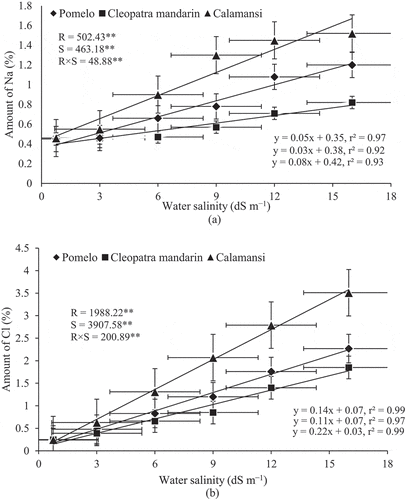
In case of Cl – content, all the rootstocks showed significant positive response with an increase in salinity level ()). At 3 dS m–1 water salinity, the highest amount of Cl – was recorded in the seedlings of calamansi (0.63%), while the seedlings of Cleopatra mandarin had the lowest amount of Cl – (0.39%) followed by the seedlings of pomelo (0.48%). The highest amount of Cl – was recorded in the seedlings of calamansi (3.51%) at 16 dS m–1 water salinity followed by the seedlings of pomelo (2.27%) and Cleopatra mandarin (1.85%). The highest slope of the regression line was observed for calamansi (0.22) indicating high responsiveness of this rootstock to increasing salinity level compared with the other two rootstocks.
The presence of NaCl in irrigation water significantly reduced N, P, and K contents in the leaves of citrus rootstocks and there were significant negative linear relationships between N, P, and K contents of citrus rootstocks and water salinity levels (–), respectively). The amount of N was consistently decreased in all the rootstocks with increasing NaCl concentration in irrigation water, and varied from 3.19% to 2.26% in pomelo, 3.30% to 2.66% in Cleopatra mandarin and 3.18% to 1.36% in calamansi at 0.75 and 16 dS m–1 salinity levels, respectively ()). Like all other parameters, P accumulation in leaves was also negatively influenced by salinity. The degree of reduction in P accumulation was the lowest in pomelo (24.3%) followed by Cleopatra mandarin (34.1%) and calamansi seedlings (37.5%) at 16 dS m–1 water salinity compared with the control ()). K content was significantly reduced in all the rootstocks with an increase in salinity. The highest amount of K (1.98%) was accumulated in the seedlings of Cleopatra mandarin when irrigated with normal water (0.75 dS m–1) and it was statistically at par with other rootstocks under the same treatment ()). The seedlings of calamansi had the lowest amount of K (0.86%) with the highest NaCl concentration (16 dS m–1) followed by the seedlings of pomelo (1.32%) and Cleopatra mandarin (1.75%). These results indicate that all the rootstocks contained less amount of P in their leaves compared with N and K.
Figure 5. Relationship between amounts of nitrogen (N) and water salinity (a), phosphorous (P) and water salinity (b) and potassium (K) and water salinity (c) of citrus rootstocks after 90 days of irrigation with different levels of water salinity (R = Rootstocks, S = Water salinity, ** Significant at P< .01). Error bars on both axes represent standard error.
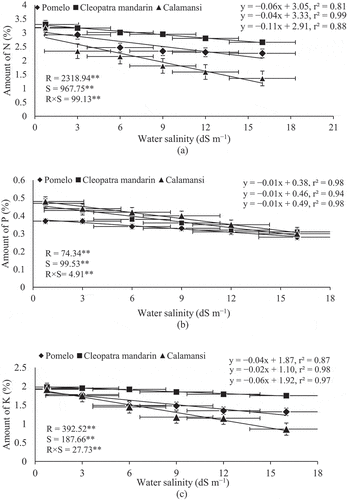
Effect of Salinity on Biochemical Parameters
Total chlorophyll and proline contents of three rootstocks were significantly affected due to the application of saline water (). A negative linear relationship was observed between chlorophyll content of citrus rootstocks and water salinity levels ()). The highest amount of chlorophyll (2.56 mg g–1) was recorded in the seedlings of Cleopatra mandarin when irrigated with normal water (0.75 dS m–1) followed by that of the same rootstock with 3 dS m–1 saline water. The lowest amount of chlorophyll (0.74 mg g–1) was recorded in the seedlings of calamansi when exposed to the highest water salinity (16 dS m–1). The highest salinity level of 16 dS m–1 resulted in the lowest chlorophyll content irrespective of citrus species. The amount of chlorophyll was 0.74, 1.43 and 1.70 mg g–1 in calamansi, pomelo and Cleopatra mandarin, respectively, when irrigated with 16 dS m–1 saline water. The highest chlorophyll reduction was observed in calamansi (60%) followed by pomelo (38%), while the lowest reduction was observed in Cleopatra mandarin (34%) when water salinity level was increased from 0.75 to 16 dS m–1 ()).
Figure 6. Relationship between amount of chlorophyll and water salinity (a) and between amount of proline and water salinity (b) of citrus rootstocks after 90 days of irrigation with different levels of water salinity (R = Rootstocks, S = Water salinity, ** Significant at P< .01). Error bars on both axes represent standard error.
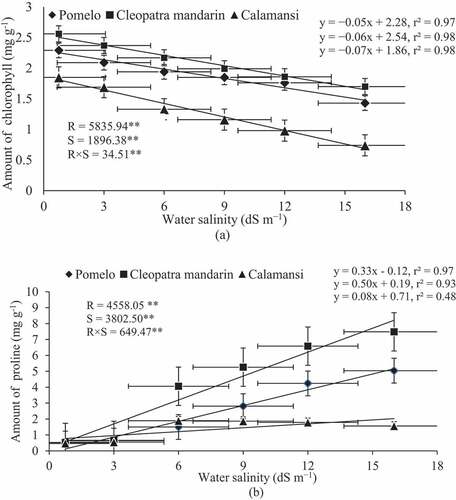
Proline accumulation of all rootstocks was significantly influenced by different salinity levels (). A positive linear relationship was found between proline accumulation of citrus rootstocks and water salinity levels ()). Proline content was the lowest and statistically similar in all three rootstocks when irrigated with normal water (0.75 dS m–1). The highest amount of proline (7.48 mg g–1) was recorded in the leaves of Cleopatra mandarin at 16 dS m–1 saline water followed by pomelo (5.04 mg g–1) and calamansi (1.56 mg g–1). The amount of proline was increased by 14 times in the leaves of Cleopatra mandarin when water salinity was increased from 0.75 to 16 dS m–1. The slope of the regression line was higher in Cleopatra mandarin (0.50) followed by pomelo (0.33), while the lowest of that was observed in calamansi (0.08). These results indicate that the seedlings of Cleopatra mandarin are more efficient in proline synthesis with an increasing level of water salinity ()).
Discussion
A variety of selection procedures has been used to find out the suitable citrus rootstocks for salt-affected soil. Among them, the most frequently used criteria were the measurement of vigorousness by establishing difference in height of plant, leaves initiation and defoliation, leaf area and Cl toxicity in leaves. It has been reported that growth suppression is one of the prime responses to salt stress in citrus and many other plants (Verslues et al., Citation2006). In the present study, Cleopatra mandarin showed better growth performance against different water salinity levels compared with other rootstocks, while the seedlings of calamansi had poor growth performance among all the rootstocks. The difference in the performance of the studied rootstocks under various levels of salinity might be due to a variation in internal capability to cope with elevated salinity. All the tested rootstocks have their distinct growth characteristics under non-stress conditions (0.75 dS m–1). At this condition, rootstocks had differences in plant height, shoot diameter, leaf size, and total biomass production. In this situation, comparison of changes in growth attributes under normal water and the highest level of salinity (16 dS m–1) would be more convenient to evaluate the growth performance of all citrus rootstocks. Yokota (Citation2003) mentioned that, based on the reduction of plant growth parameters, salt-stressed plants revealed no typical symptoms; only difference with normal plants could validate the degree of salt stress.
In this study, growth parameters of all the rootstocks were significantly reduced with the application of different levels of saline water (3–16 dS m–1). Seedlings of Cleopatra mandarin had better adaptive capability to grow under irrigation with higher NaCl concentration, whereas opposite results were evident in calamansi. Our results are similar to Anjum (Citation2008), who also reported better performance of the seedlings of Cleopatra mandarin over seedlings of Tryor citrange when exposed to different levels of NaCl salinity (0, 40 and 80 mM) in terms of growth parameters. In another study, Hussain et al. (Citation2015) observed significant differences among the seedlings of 20 citrus genotypes when treated with 75 mM of NaCl (moderate salt stress) for 12 weeks. They observed better tolerance of Cleopatra mandarin and pomelo to salt stress, while citron was very sensitive.
Dry weights of root and shoot were reduced in all rootstocks due to the application of different concentrations of saline water; however, the reduction was less in Cleopatra mandarin and was more in calamansi. This reduction in root and shoot dry weights with an increasing level of salinity might be due to the fact that high-salinity level interferes with the physiological and biochemical characteristics of seedlings (Munns and Tester, Citation2008) and reduces the number and area of leaves (Dong et al., Citation2007). Cells need extra energy when exposed to higher salinity for maintaining normal physiological processes including photosynthesis and thereby reduce the translocation of carbohydrates needed for normal growth and development. In addition, salt stress may also shrink root, shoot and leaves by decreasing turgor pressure in plant and accordingly reducing further cell growth and development (Sirousmehr et al., Citation2014). Camara-Zapata et al. (Citation2004) also reported a reduction in growth rate in different citrus rootstocks due to high osmolality in the growing medium. Balal et al. (Citation2011) observed a significant reduction in growth attributes of different citrus rootstocks due to the effect of NaCl where the reduction was larger at higher NaCl concentrations (60 and 90 mM). Higher concentration of salts in the root zone area creates an artificial deficit of water in the root zone and this situation forces plant to use more energy for water uptake from the soil solution (physiological drought), which hinders the growth of plant. It was observed that the seedlings of Cleopatra mandarin were the least affected and the seedlings of calamansi were the most affected rootstock when exposed to different levels of salinity in terms of growth.
Nutrient contents of three citrus rootstocks were significantly affected by different levels of water salinity. Similar results were also observed by Anjum (Citation2008). It was also observed that leaf tissues accumulated more Na+ and Cl – ions and consequently other nutrient contents were reduced in the leaf tissues when NaCl concentration was increased in irrigation water. The seedlings of Cleopatra mandarin were the lowest accumulator of Na+ and Cl – in their leaves, while the seedlings of calamansi were the highest accumulator of these ions when exposed to the highest level of water salinity (16 dS m–1). Overall, the seedlings of Cleopatra mandarin were efficient excluders of Na+ and Cl – ions, while the seedlings of calamansi showed lower exclusion of these ions. Therefore, the leaves of Cleopatra mandarin accumulated less Na+ and Cl – compared with the other two rootstocks, while the leaves of calamansi contained higher amount of these ions. This could be the possible reason that the seedlings of calamansi and Cleopatra mandarin showed more sensitivity and tolerance to water salinity, respectively. Similarly, Hussain et al. (Citation2012) also observed Cleopatra mandarin, pomelo and Australian sour orange as the most tolerant genotypes to salt stress among 12 genotypes of citrus, while Poncire commun citron and Marumi kumquat were the most sensitive species when treated with 75 mM of NaCl (moderate salt stress) for 12 weeks. At the end of their study, Cleopatra mandarin accumulated the lowest amount of Na+ and Cl–, while Poncire commun citron accumulated the highest amount of these ions. Grieve and Walker (Citation1983) also suggested that Na+ and Cl – exclusion properties should be taken into consideration when plants are screened for salt tolerance because it may vary across species.
Other nutrient contents were also affected by Na+ and Cl – ions, which ultimately showed their impact on plant growth and development. As a result, the seedlings of Cleopatra mandarin showed the best performance in terms of survivability, plant height, shoot diameter, leaf area and dry weights of root and shoot under all levels of salinity, whereas calamansi performed the least for most of these parameters under all levels of salinity. Specific nutrient deficiency might not be the sole factor of growth reduction and injury to citrus rootstocks. Salt injury to citrus plants has been mostly attributed to extreme accumulation of Cl – and Na+ in the leaves (Khoshbakht et al., Citation2015; Zekri, Citation1993). NaCl significantly reduced N, P, and K contents in the leaf tissue of all rootstocks. The degree of water and nutrient uptake by citrus rootstocks under salt stress is species-specific, which largely determines nutrient accumulation ability of the citrus species in question, and subsequently affects plant growth and development. Osmotic stress is induced by low osmotic potential, which reduces absorption of water from soil, and ionic stress is created by the excess-accumulation of lethal salt ions inside plant cells. These stresses along with nutritional inequality affect physiological traits, plant growth, and development.
Chlorophyll content of all rootstocks was decreased, while proline content was increased with an increase in salinity level. Biochemical ingredients play a vital role in plant growth and development; hence, the variation in these parameters has obvious impact on the growth and further development of all rootstocks. Chlorophyll content was higher in all rootstocks at the control (0.75 dS m–1), while it was the lowest at the highest NaCl concentration (16 dS m–1). Balal et al. (Citation2011) reported a reduction in chlorophyll content in citrus rootstocks due to the application of NaCl. Khoshbakht et al. (Citation2015) also reported a reduction in the amount of chlorophyll when the level of salinity increased in the growing medium. Proline is one of the most important biochemical parameters for selecting salt-sensitive or tolerance level of different plants. Many plants synthesize higher amount of proline when exposed to drought (Hare et al., Citation1998) or high salt stress (Munns, Citation2005) compared with other amino acids. Among the three studied rootstocks, the seedlings of Cleopatra mandarin accumulated the maximum amount of proline under all salinity levels and were more tolerant against water salinity. The seedlings of calamansi were more sensitive to water salinity and an increasing amount of proline accumulation was evident up to 6 dS m–1, but proline accumulation was decreased above this salinity level. Plants cope with adverse environmental conditions like high salinity or drought using a variety of mechanisms such as accumulation of inorganic ions and osmolytes like proline. Plants accumulate various types of osmolytes (proline, betaine, amino acids, sugar, and polyols) under salt stress. Huang et al. (Citation2013) observed higher proline accumulation in salt-tolerant plants compared with salt-sensitive plants under salt stress. In another study, Anjum (Citation2008) reported higher accumulation of proline in the seedlings of Cleopatra mandarin at 80 mM salinity compared with Tryor citrange. Balal et al. (Citation2011) reported an increased proline accumulation in Rangpur lime seedlings with an increase in salinity level compared with others. In their study, Rangpur lime accumulated the highest amount of proline (6.6 ± 0.5 mg g–1) among 10 seedlings of citrus rootstocks when treated with the highest level of NaCl salinity (90 mM) at 90 days after treatment. In the present study, the seedlings of Cleopatra mandarin accumulated higher amount of proline when exposed to 16 dS m–1 saline water followed by 12 dS m–1 saline water. Higher proline content induces an instant mechanism for adjusting the osmotic effect, alters the solubility of different proteins and protects those proteins from denaturation under salt stress (Tonon et al., Citation2004). Proline accumulation helps in membrane stability, which in turn mitigates the adverse effect of NaCl on cell membrane disruption (Dondini et al., Citation2001). In another study, Hussain et al. (Citation2018) observed a decrease in total chlorophyll content, but an increase in proline content when citrus rootstocks were exposed to drought stress.
Conclusion
The results of the present study indicate differential level of tolerance among the three citrus rootstocks to salinity stress. The performance of the seedlings of Cleopatra mandarin was the best followed by pomelo, while the seedlings of calamansi were the most sensitive to water salinity in terms of physical growth, mineral nutrient accumulation and biochemical parameters. It can be concluded that Cleopatra mandarin and pomelo were more tolerant of saline conditions than calamansi. It is suggested that plant breeding programs consider the relative susceptibility of citrus rootstocks to saline conditions for further improvement.
Acknowledgments
The authors thank the NICHE-BGD-156 Project, The Netherlands and the Asian Institute of Technology, Thailand, for providing financial assistance in the form of a scholarship to the first author for graduate studies at the Asian Institute of Technology, Thailand. The authors also acknowledge the Bangladesh Agricultural Research Institute for providing laboratory support for the work.
Additional information
Funding
References
- Abbas, G., M. Saqib, Q. Rafique, M.A. ur-Rahman, J. Akhtar, M.A. Ul- Haq, and M. Nasim. 2013. Effect of salinity on grain yield and grain quality of wheat (Triticum aestivum L.). Pak J. Agric. Sci 50:185–189.
- Anjum, M.A. 2008. Effect of NaCl concentrations in irrigation water on growth and polyamine metabolism in two citrus rootstocks with different levels of salinity tolerance. Acta Physiol. Plant. 30:43–52. doi: 10.1007/s11738-007-0089-3.
- Arbona, V., M.F. Lopez-Climent, J. Mehouachi, R.M. Perez-Clement, S.R. Abrams, and A. Gomez-Cadenas. 2006. Use of persistent analogues of abscisic acid as palliatives against salt-stress induced damage in citrus plants. J. Plant Growth Regul. 25:1–9. doi: 10.1007/s00344-005-0038-6.
- Balal, R.M., M.Y. Ashraf, M.M. Khan, M.J. Jaskani, and M. Ashfaq. 2011. Influence of salt stress on growth and biochemical parameters of citrus rootstocks. Pak. J. Bot. 43:2135–2141.
- Bates, L., R.P. Waldren, and I.D. Teare. 1973. Rapid determination of free proline for water-stress studies. Plant Soil. 39:205–207. doi: 10.1007/BF00018060.
- Camara-Zapata, J.M., F. Garcia-Sanchez, V. Martinez, M. Nieves, and A. Cerda. 2004. Effect of NaCl on citrus cultivars. Agronomie. 24:155–160. doi: 10.1051/agro:2004013.
- Dondini, L., S. Bonazzi, S. Del-Duca, A.M. Bregoli, and D. Serafni-Fracassini. 2001. Acclimation of chloroplast transglutaminase to high NaCl concentration in a polyamine deficient variant strain in Dunaliella salina and in its wild type. J. Plant Physiol. 158:185–197. doi: 10.1078/0176-1617-00099.
- Dong, Y., T. Ji, and S. Dong. 2007. Stress responses to rapid temperature changes of the juvenile sea cucumber (Apostichopus japonicus Selenka). J. Ocean Univ. China. 6:275–280. doi: 10.1007/s11802-007-0275-3.
- Gainza, F., I. Opazo, V. Guajardo, P. Meza, M. Ortiz, J. Pinochet, and C. Munoz. 2015. Rootstock breeding in Prunus species: Ongoing efforts and new challenges. Chil. J. Agric. Res. 75:6–16. doi: 10.4067/S0718-58392015000300002.
- Garcia-Sanchez, F., M. Carvajal, M.A. Sanchez-Pina, and V. Martinez. 2000. Salinity resistance of citrus seedlings in relation to hydraulic conductance, plasma membrane ATPase and anatomy of the roots. J. Plant Physiol. 156:724–730. doi: 10.1016/S0176-1617(00)80238-2.
- Grieve, A.M., and R.R. Walker. 1983. Uptake and distribution of chloride, sodium and potassium ions in salt treated citrus plants. Aust. J. Agric. Res. 34:133–143. doi: 10.1071/AR9830133.
- Hare, P.D., W.A. Cress, and J. Van Staden. 1998. Dissecting the roles of osmolyte accumulation during stress. Plant Cell Environ. 21:535–553. doi: 10.1046/j.1365-3040.1998.00309.x.
- Huang, Z., L. Zhao, D. Chen, M. Liang, Z. Liu, H. Shao, and X. Long. 2013. Salt stress encourages proline accumulation by regulating proline biosynthesis and degradation in Jerusalem artichoke plantlets. PLoS ONE 8(4):e62085. doi: 10.1371/journal.pone.0062085.
- Hussain, S., M.F. Khalid, M. Saqib, S. Ahmad, W. Zafar, M.J. Rao, R. Morillon, and M.A. Anjum. 2018. Drought tolerance in citrus rootstocks is associated with better antioxidant defense mechanism. Acta Physiol. Plant. 40:135. doi: 10.1007/s11738-018-2710-z.
- Hussain, S., F. Luro, G. Costantino, P. Ollitrault, and R. Morillon. 2012. Physiological analysis of salt stress behaviour of citrus species and genera: Low chloride accumulation as an indicator of salt tolerance. S. Afr. J. Bot. 81:103–112. doi: 10.1016/j.sajb.2012.06.004.
- Hussain, S., R. Morillon, M.A. Anjum, P. Ollitrault, G. Costantino, and F. Luro. 2015. Genetic diversity revealed by physiological behavior of citrus genotypes subjected to salt stress. Acta Physiol. Plant. 37:1740. doi: 10.1007/s11738-014-1740-4.
- Khoshbakht, D., A.A. Ramin, and B. Baninasab. 2015. Effects of sodium chloride stress on gas exchange, chlorophyll content and nutrient concentrations of nine citrus rootstocks. Photosynthetica. 53:241–249. doi: 10.1007/s11099-015-0098-1.
- Lauchli, A., and E. Epstein. 1990. Plant responses to saline and sodic conditions, p. 113–137. In: K.K. Tanji (ed.). Agricultural Salinity Assessment and Management. Manuals and Reports on Engineering Practice. American Social Civil Engineering, New York.
- Maas, E.V. 1993. Salinity and citriculture. Tree Physiol. 12:195–216. doi: 10.1093/treephys/12.2.195.
- Moya, J.L., A. Gomez-Cadnes, E. Primo-Millo, and M. Talon. 2003. Chloride absorption in salt-sensitive Carrizo citrange and salt-tolerant Cleopatra mandarin citrus rootstocks is linked to water use. J. Exp. Bot. 54:825–833. doi: 10.1093/jxb/erg064.
- Munns, R. 2005. Genes and salt tolerance: Bringing them together. New Phytol. 167:645–663. doi: 10.1111/j.1469-8137.2005.01487.x.
- Munns, R., and M. Tester. 2008. Mechanisms of salinity tolerance. Annu. Rev. Plant Biol. 59:651–681. doi: 10.1146/annurev.arplant.59.032607.092911.
- Simpson, C.R., S.D. Nelson, J.C. Melgar, J. Jifon, S.R. King, G. Schuster, and A. Volder. 2014. Growth response of grafted and ungrafted citrus trees to saline irrigation. Sci. Hort. 169:199–205. doi: 10.1016/j.scienta.2014.02.020.
- Sirousmehr, A., J. Arbabi, and M.R. Asgharipour. 2014. Effect of drought stress levels and organic manures on yield, essential oil content and some morphological characteristics of sweet basil (Ocimum basilicum). Adv. Environ. Biol. 8:880–886.
- SRDI. 2010. Saline Soils of Bangladesh. Soil Resources Development Institute, Ministry of Agriculture, Dhaka, Bangladesh.
- Tonon, G., C. Kevers, O. Faivre-Rampant, M. Graziani, and T. Gaspar. 2004. Effect of NaCl and mannitol iso-osmotic stresses on proline and free polyamine levels in embryogenic Fraxinus angustifolia callus. J. Plant Physiol. 161:701–708. doi: 10.1078/0176-1617-01084.
- Verslues, P.E., M. Agarwal, S.K. Agarwal, J. Zhu, and J.K. Zhu. 2006. Methods and concepts in quantifying resistance to drought, salt and freezing, abiotic stresses that affect plant water status. Plant J. 45:523–539. doi: 10.1111/j.1365-313X.2005.02593.x.
- Wellburn, A.R. 1994. The spectral determination of chlorophyll a and b, as well as total carotenoids, using various solvents with spectrophotometer of different resolution. J. Plant Physiol. 144:307–3013. doi: 10.1016/S0176-1617(11)81192-2.
- Yokota, S. 2003. Relationship between salt tolerance and proline accumulation in Australian acacia species. J. For. Res. 8:89–93. doi: 10.1007/s103100300010.
- Zekri, M. 1993. Seedling emergence, growth and mineral concentration of three citrus rootstocks under salt stress. J. Plant. Nutr. 16:1555–1568. doi: 10.1080/01904169309364633.