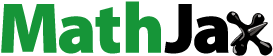
ABSTRACT
Pomegranate (Punica granatum L.) is one of the most important Iranian horticultural products, which is usually cultivated in arid and semi-arid regions. Climate change has led to drought stress and it’s a threat to the agricultural products as well as pomegranate. This experiment was conducted on two-year-old rooted pomegranates of eight commercial cultivars namely ‘Malase Saveh’ (‘M-Saveh’), ‘Malase Isfahan’ (‘M-Isfahan’), ‘Robabe Ghermeze Shiraz’ (‘Robab’), ‘Gabrie Yazd’ (‘G-Yazd’), Gabrie Torshe Yazd’ (‘GT-Yazd’), ‘Zaghe Sefide Yazd’ (‘ZS-Yazd’), ‘Zaghe Torshe Yazd’ (‘ZT-Yazd’) and ‘Malase Torshe Pishva’ (‘M-Pishva’). Irrigation treatments were 50% of maximum allowable deficiency (without stress) and 80% of maximum allowable deficiency (stress). This research carried out as a factorial experiment based on randomized complete block design. Increased proline content was variable in pomegranate cultivars exposed to stress, so that, the highest increase was observed in the ‘M-Saveh’ with a 20.27% increase compared to the control treatment. ‘Robab’ showed the lowest increase in proline content. The highest increase in superoxide dismutase and peroxidase activity was 8.49 and 16.84%, respectively, in ‘ZS-Yazd’. The activity of superoxide dismutase in ‘Robab’, which was exposed to drought stress, decreased. This cultivar showed the highest electrolyte leakage with an average of 83.58%. The lowest electrolyte leakage was belonged to ‘ZS-Yazd’ (24.1%), and then ‘GT-Yazd’ (18.66%). Cluster analysis divided the genotypes into four main groups. ‘ZS-Yazd’ and ‘M-Saveh’ were in the same group. These two cultivars were more tolerant to drought stress than other cultivars in the physiological aspects.
Introduction
Iran has the richest genetic resources of pomegranate cultivars in the world so that more than at least 760 pomegranate cultivars have been collected from different provinces of this country (Behzadi Shahrbabaki, Citation1998). This product has a special place in traditional medicine due to its pharmacological properties (Bagri et al., Citation2009) and the minimum consumption of insecticide and fertilizer in pomegranate orchards. Different organs of pomegranate such as fruit, leaf, flower, skin, branch, and root have many medicinal properties (Miguel et al., Citation2010).
One of the most important limiting factors for plant growth, especially fruit trees in arid and semi-arid regions, is the limitation of water resources. Global warming has been exacerbated the negative effects of drought stress (Xoconostle-Cazares et al., Citation2010). To reduce the effects of drought stress and the optimal use of water resources, the recognition of drought-tolerant plants, along with understanding the drought tolerance mechanisms, is very important (Cheruth et al., Citation2009). Plants can withstand drought stress by the application of multiple mechanisms. These mechanisms are drought escape and tolerance, which vary between species and cultivars (Cheruth et al., Citation2009). It is very important to identify the physiological markers that help the breeders to recognize cultivars with more tolerance to drought stress in the seedling phase. Ion leakage, proline content and antioxidant enzymes are some of these physiological markers (Gholami et al., Citation2012; Sircelj et al., Citation2007).
One of the osmoprotectant compounds is proline. Proline accumulation is associated with increased tolerance to abiotic stresses, especially salinity and drought stress (Mahajan and Tuteja, Citation2005). One of the most important mechanisms of drought tolerance in plants is to increase the osmotic potential of the cells and consequently to increase the water potential of the plant (Waseem et al., Citation2011). Drought stress disrupts cell membrane activity, and consequently, the cell membrane stability index decreases in plants and ions leak out from the inside to the outside of the leaf cells. Measurement of electrolyte leakage in plant tissues is a suitable method for estimating the health of the membrane after environmental stresses such as drought (Medrano et al., Citation2002). On the other hand, damage to the membrane results in the accumulation of reactive oxygen species (ROS), which also affects the permeability of the membrane (Foyer and Noctor, Citation2005). It has been reported that water shortage leads to oxidative stress and can be neutralized by the antioxidant system in plants (Andre et al., Citation2009; Roby et al., Citation2004). Therefore, osmotic regulation and maintenance of turgor pressure, as well as ROS scavengers (enzymatic and non-enzymatic antioxidants), are involved in reducing damage to biological membranes. These strategies depend on the plant cultivar and the severity of drought stress (Pourghayoumi et al., Citation2017).
One of the most reliable and permanent ways to prevent inappropriate consumption of water and save water resources in agriculture is the use of tolerant cultivars and species to drought stress in arid and semi-arid regions (Kafi et al., Citation2010). Pomegranate cultivars are very diverse and are cultivated on the edge of the desert due to its relative tolerance to water shortage, but pomegranate orchards have been damaged in recent years due to severe drought stress and reduce groundwater resources in Iran. An increase in fruit splitting or cracking and a decrease in vegetative growth and yield in pomegranate orchards is due to drought stress (Jain et al., Citation2011). Although in recent years, considerable scientific information has been obtained on the different aspects of this fruit, however, there is no accurate information on the tolerance of cultivars to drought stress because of the diversity of cultivars. The aim of this research is screening eight Iranian commercial pomegranates against drought stress based on some physiological markers. The result of this experiment will lead to the introduction of the most tolerant and sensitive studied pomegranate cultivar or cultivars to drought stress.
Materials and Methods
Plant Material and Drought Stress Applications
This research was carried out in Isfahan Agricultural Research Center with latitude and longitude coordinates 32.36° N and 51.36° E. in 2017 and 2018. This research was conducted as a factorial experiment based on a randomized complete block design with two factors, including pomegranate cultivars and irrigation treatment with three replicates and 10 plants in each replication. Two-year-old rooted pomegranates of eight commercial cultivars namely ‘Malase Saveh’ (‘M-Saveh’), ‘Malase Isfahan’ (‘M-Isfahan’), ‘Robabe Ghermeze Shiraz’ (‘Robab’), ‘Gabrie Yazd’ (‘G-Yazd’), Gabrie Torshe Yazd’ (‘GT-Yazd’), ‘Zaghe Sefide Yazd’ (‘ZS-Yazd’), ‘Zaghe Torshe Yazd’ (‘ZT-Yazd’) and ‘Malase Torshe Pishva’ (‘M-Pishva’) were planted outdoors at a distance of 1 × 2 meters and irrigated by a tape system. Age, diameter and size of pomegranate cultivars were the same. The physical and chemical properties of the soil mixture were analyzed at the beginning of the experiment ().
Table 1. Some physical and chemical properties of the soil mixture at the beginning of the experiment
The experiment began in June 2017, which coincided with the beginning of the heat and increase water consumption in Isfahan, and continued until the end of September. The experiment was also repeated in 2018. The moisture treatments were based on maximum allowable deficiency and included 50% (without stress) and 80% (stress treatment) of maximum allowable deficiency. The moisture treatments were carried out by measuring the moisture content of the soil using a TDR humidifier (Trase6050X1). During this time, the rainfall did not happen or it was very small so that it was not included in the calculations.
To achieve the amount of irrigation (irrigation volume), some factors, such as net and gross irrigation depth, root development depth, amount of leaching and irrigation efficiency were considered (Doorenbos and Pruitt, Citation1977). The volume of water was controlled by the installed counter on the pipe before the irrigation plot. For the irrigation of cultivars, leaked tubes with drippler at 10 cm intervals were used. The outlet flow of each dripper was measured in one liter per hour at a standard pressure.
Evaluated Traits
The height of cultivars was measured before stress and after the end of the stress using bar meter and the height difference before and after stress was calculated.
Cell membrane stability was determined as electrolyte leakage (EL). For EL analysis, whole fully-expanded leaves (0.1 g from each pot) were incubated in 15 ml distilled water on a shaker for 24 h. The conductance of the incubation solution was measured as the initial level of EL (Ci) using a conductance meter (YSI-3100, Guangzhu, China). Leaf tissue in the incubation solution was killed in an autoclave at 120°C for 30 min. The conductance of the incubation solution with killed tissues (Cmax) was determined following 24 h incubation on a shaker. EL was calculated as (Ci/Cmax) × 100 (Blum and Ebercon, Citation1981).
The proline content was determined by the method given by Bates et al. (Citation1973). For this, 10 ml of 3% (w/v) sulphosalicylic acid was added to 0.4 g leaf materials. The homogenate was filtered through filter paper. Two ml acetic acid and 2 ml acidic ninhydrin regent were added to a 2 ml aliquot. The mixture was thoroughly stirred and incubated in a boiling water bath for 1 h and then the reaction was stopped by using an ice bath and warmed to room temperature. The mixture was extracted with toluene and the absorbance of the fraction with toluene extracted from the liquid phase was read at 518 nm. The concentration of proline in the sample was computed from a standard curve of proline.
For peroxidase (POD), the oxidation of guaiacol was measured by the increase in absorbance at 470 nm for 1 min. The reaction solution contained 450 µl of 225 mM guaiacol, 100 µl of 50 mM phosphate buffer (pH 7.0) and 100 µl enzyme extract. The reaction was started with 450 µl of 45 mM H2O2 (Zhang, Citation1992).
The superoxide dismutase (SOD) activity was assayed by monitoring its ability to inhibit the photochemical reduction of nitroblue tetrazolium (NBT). One unit of SOD was defined as the amount of enzyme necessary to cause 50% inhibition of the rate of NBT reduction at 560 nm. In order to 1 ml reaction mixture contained 75 µM NBT, 2 µM riboflavin, 13 mM methionine, 0.1 mM EDTA, 50 mM phosphate buffer (pH 7.0) and 50 µl of enzyme extraction. The test cells containing the mixture were placed on a shaker under light at 78 µmol photons s−1 m−1 for 15 min and absorbance at 560 nm was recorded. A non-irradiated reaction mixture that did not develop color served as the control and its absorbance was subtracted from A 560 of the reaction solution (Giannopolities and Rise, Citation1977).
Qualitative traits such as leaf abscission, necrosis, and dryness were visually recorded at the end of the stress period. Necrosis, chlorosis, and dryness of 0 to 10%, 10% to 30%, 30% to 50%, 50% to 70% and 70 to 90% were considered as very low, low, medium, high and very high, respectively (Acosta-Motos et al., Citation2017). Viability percentage of plants was calculated by comparing the number of plants at the beginning and the end of the experiment according to the following formula:
Data Analysis
Data analysis was performed using SAS software (version 9.1) and mean comparison by the LSD method. Cluster analysis (Ward method based on Squared Euclidean Distance) was done using SPSS software (version 15) (Ghaderi-Ghahfarokhi et al., Citation2016).
Results
The Height Difference and Proline Content
The average height difference at the beginning and the end of stress in control and under stress plants were 32.09 and 30.53 cm, respectively. The height difference of plants in cultivars exposed to stress significantly was reduced (). This decrease in height was 4.79%.
According to , proline content was changed from 1.93 µMg−1 FW in ‘M-Saveh’ under drought stress to 1.49 µMg−1 FW in ‘GT-Yazd’ under normal conditions. In general, in all cultivars, drought stress increased leaf proline content, but this increase was variable in pomegranate cultivars. The highest increase in proline content was observed in ‘M-Saveh’ which increased by 20.72% in drought stress conditions compared to control treatment. After that, ‘M-Pishva’ produced the highest proline content in drought stress conditions. ‘Robab’ showed the lowest increase in proline content, which was 1.22%. This cultivar in the control treatment had a higher proline content than ‘M-Saveh’, but under stress conditions, its proline content was lower than other cultivars.
SOD and POD Activity
The highest activity of the SOD enzyme was observed in ‘ZS-Yazd’ exposed to stress with an average of 2.002 unit mg−1 protein. The lowest activity of this enzyme was also observed in ‘G-Yazd’ control treatment (1.79 unit mg−1 protein). In both ‘M-Saveh’ and ‘ZT-Yazd’ cultivars, the average of SOD activity in the control and stress conditions was similar. In ‘Robab’ under drought stress, the activity of this enzyme was 3.2% lower than the control treatment. In other cultivars, drought stress led to an increase in the activity of this enzyme. The highest increase in SOD activity was observed in ‘ZS- Yazd’ which was 8.49% ().
‘ZS-Yazd’ showed the highest POD activity with an average of 0.95 μm min−1 mg−1 protein under stress conditions. This cultivar showed the lowest activity of this enzyme in the control condition (0.79 μm min−1 mg−1 protein). The activity of this enzyme in ‘G-Yazd’ was similar in both control and stress treatments. ‘GT-Yazd’ and ‘M-Pishva’ cultivars showed a low reduction in the activity of POD under stress compared to control treatment, but this decrease was not significant. Other cultivars showed an increase in POD activity. The highest and lowest increases belonged to ‘ZS-Yazd’ (16.84%) and ‘ZT-Yazd’ (1.17%), respectively ().
El and Viability Percentage
The effect of drought stress and pomegranate cultivars on EL was not significant, but EL had a significant effect on each of these factors separately. ‘Robab’ showed the highest EL with an average of 58.83%. The lowest EL was observed in ‘ZS-Yazd’ (24.1%) and then in ‘GT-Yazd’ (26.18%) (). Other cultivars had no significant difference with each other.
Drought stress resulted in increased EL (from 27.84 to 50.27%) and decreased chlorophyll index (from 56.93 to 46.48). Drought stress led to an increase of 44.61% in EL and a decrease of 18.35% in the chlorophyll index ().
The viability percentage of cultivars, as well as qualitative traits in control and stress treatment, are recorded in . According to this table, plant dryness was observed in three cultivars including ‘GT-Yazd’, ‘Robab’ and ‘M-Isfahan’. Other cultivars did not have drought losses. The lowest viability percentage of the plant (60%) belonged to ‘Robab’. In the stress treatment, the highest leaf abscission, necrosis, and dryness belonged to ‘Robab’ and ‘M-Pishva’. Under these conditions, ‘M-Isfahan’, ‘M-Saveh’ and ‘ZT-Yazd’ had the lowest leaf abscission, necrosis, and dryness.
Table 2. The viability percentage and some qualitative traits in different cultivars of pomegranate in control and drought stress treatments
Cluster Analysis
Cluster analysis was classified cultivars into four groups at five squared Euclidean distance (). In the first group, ‘Robab’ cultivar was placed. This cultivar showed lower tolerance to drought stress than other cultivars. More decrease of proline content and more increase of EL in drought stress conditions are characteristics of this cultivar. In the fourth group, ‘ZS-Yazd’ and ‘M-Saveh’ were placed. These two cultivars showed higher drought stress tolerance because of proline production and increase antioxidant activity and decrease EL. ‘G-Yazd’ and ‘GT-Yazd’ were placed in the second group. In this group, the proline content and peroxidase activity were slightly higher than the first group. ‘ZT-Yazd’, ‘M-Isfahan’ and ‘M-Pishva’ were located in the third group. The characteristics of cultivars in the second and third groups were the middle of characteristics of cultivars in the first and fourth groups.
Discussion
The Height Difference and Proline Content
Breeders are looking for new sources of diversity to find drought-tolerant plants with appropriate characteristics, but stress tolerance is very complex and is influenced by morphological, physiological, biochemical and molecular responses of the plant (Giancarla et al., Citation2012). One of the traits that influenced by drought stress is plant height. In this research, the height of plants slightly decreased with drought stress (). Drought stress has different effects on plant growth parameters (Xu et al., Citation2007). Jinying et al. (Citation2007) and Wu and Xia (Citation2006) showed that drought stress harmed seedling growth by studying the effect of drought stress on the height of jujube and orange seedlings, respectively. The plant growth phenomenon is the result of vital activities in the presence of enough water. If the required water of the plant is not provided, the growth of the plant decreases due to decreasing the turgor pressure of growing cells and effect on the cell length (Mirzaei and Karamshahi, Citation2015).
One of the most important factors in drought tolerance in pomegranate is the improvement of the cell osmolality conditions through the accumulation of suitable compounds such as proline (Rodriguez et al., Citation2012). They have introduced pomegranate as one of the drought-compatible species. In all cultivars, drought stress increased leaf proline content, but this increase was variable in pomegranate cultivars (). Increase proline content was reported in the pomegranate cultivars from 0.41% to 1.08% due to drought stress (Khattab et al., Citation2011), which the increase in proline content in the present study was more than reported values. The highest increase in proline content was observed in ‘M-Saveh’ and then ‘MT-Pishva’ and the lowest increase was in ‘Robab’ under drought stress conditions. Rad et al. (Citation2015) reported that proline content in pomegranate leaves of ‘Robab-e-Niriz cultivar was 2.49 µMg−1 FW in moderate drought stress, which was higher than the rate of reported proline in current research for ‘Robab’ cultivar in severe drought stress (1.63 µMg−1 FW). The cultivar, drought intensity, age of trees and experiment conditions can be affected by this difference. ‘Robab’ had a higher proline content than that of ‘M-Saveh’ in control treatment, but under stress conditions, its the proline content was lower than other cultivars. It seems that an increase in proline content is more important than the rate of proline for screening tolerant cultivars.
SOD and POD Activity
The damage caused by reactive oxygen species (oxidative stress) that may occur after biotic and abiotic stresses such as drought stress is the important limiting factors for the plant (Mittler, Citation2006). Plants for oxidative stress have a high-performance defense system that can limit or neutralize the production and efficiency of free radicals. This defense system involves the activity of antioxidant enzymes such as SOD and POD (Blokhina et al., Citation2003). Superoxide dismutase as the first defensive barrier resists against the attack of the oxygen radicals and catalyzes the reaction of superoxide to oxygen and hydrogen peroxide (Ahmad et al., Citation2009). The highest activity of SOD was observed in ‘ZS-Yazd’. Drought stress in ‘GT-Yazd’, ‘G-Yazd’, ‘M-Isfahan’, ‘M-Pishva’ and ‘ZS-Yazd’ resulted in an increase activity of this enzyme (). Similarly, Tavousi et al. (Citation2015) reported that the activity of antioxidant enzymes in pomegranate cultivars significantly increased under stress conditions. Increasing antioxidant activity of SOD, CAT, POD, APX and GPX enzymes in peach leaves under drought stress has also been reported (Wang et al., Citation2019). Unlike the mentioned cultivars, the activity of this enzyme in ‘Robab’ cultivar in stress conditions was less than the control treatment. Similarly, in tomato, SOD levels significantly decreased in the drought treatment (Feng et al., Citation2016). In peach cultivars exposed to moderate drought stress, the activity of antioxidant enzymes increased and in severe stress, their activities decreased (Wang et al., Citation2019). It is possible that the increase in the produced superoxide in this cultivar in drought stress conditions, inactivate this enzyme and lead to cell death and plant dryness.
Peroxidase plays a very important role in response to abiotic stresses such as drought. This enzyme is responsible for the removal of excess amounts of hydrogen peroxide. Similar to the SOD enzyme, the highest POD activity under stress conditions belonged to ‘ZS-Yazd’. ‘M-Isfahan’, ‘M-Saveh’, ‘Robab’, ‘ZS-Yazd’ and ‘ZT-Yazd’ had an increase in POD activity under the drought stress conditions (). Similarly, the activity of this enzyme was increased along with the activity of SOD, APX and GR enzymes in leaf and root acacia in drought stress (He et al., Citation2017). ‘GT-Yazd’ and ‘M-Pishva’ showed a slight decrease in the activity of POD under stress treatment compared to control treatment. In ‘G-Yazd’, the activity of this enzyme was similar in both control and stress treatments. The level of chemical and antioxidant compounds in pomegranate is various depending on the different environments and culture conditions (Holland et al., Citation2009) as well as cultivars (Borochov-Neori et al., Citation2009). The increase (Zhang et al., Citation1995), decrease (Zhang and Kirkham, Citation1996) and not change (Fangmeier et al., Citation1994) in POD activity has been shown in response to drought stress in different plants. It is reported that differences in results about antioxidant enzyme activities can be due to differences in cultivars, the severity of stress and the age of trees (Selahvarzi et al., Citation2017).
EL and Viability Percentage
Cell membrane stability is correlated with drought stress tolerance (Premachandra et al., Citation1992). The lowest and highest percentage of EL was observed in ‘ZS-Yazd’ and ‘Robab’, respectively (). Results of a research showed that ‘Shishe Gap’, ‘Ardestanie Mahvalat’, ‘Malase Yazd’ and ‘Shirine Shahvar’ cultivars had no significant difference in ion leakage of leaf electrolytes, and ‘Naderi’ cultivar had the highest EL (18.3%) (Hasani Moghadam et al., Citation2015). In the present study, due to prolonged and severe drought stress, EL was high and it’s varied from 24.1 to 58.83%. ‘Malase Yazd’ is one of the commercial cultivars of Iran, which in a research showed a lower degree of cell membrane stability than other cultivars (Pourghayoumi et al., Citation2017). Drought stress led to an increase in leaf EL (). Similarly, Hasani Moghadam et al. (Citation2015) reported that drought stress increased the percentage of leaf EL.
Drought stress reduced the chlorophyll index of leaves (). Under severe drought stress, the concentration of chlorophyll pigments in pomegranate leaves decreased and led to the reduction of photosynthesis (Khattab et al., Citation2011). Improving water potential or increasing root volume in the plant and absorbing enough water and nutrients such as nitrogen can restore the chlorophyll content to the desirable situation (Xoconostle-Cazares et al., Citation2010).
The highest dryness percentage of the plant belonged to ‘Robab’ under drought stress conditions (). In stress treatment, the highest and lowest leaf dryness, necrosis and abscission were observed in ‘Robab’ and ‘ZS-Yazd’, respectively. It was remarkable that ‘Robab’ did not show any leaf necrosis, dryness, and abscission in the control treatment. In fact, in these traits, ‘Robab’ showed the highest difference between control and stress treatments. The results of these morphological traits were highly consistent with the results of physiological traits in these cultivars and this helped to select more tolerant cultivars.
Cluster Analysis
Cluster analysis divides cultivars into four main groups (). ‘G-Yazd’ and ‘GT-Yazd’ with the same origin were placed in the same group. This relationship between the geographical location and grouping was also found in research carried out by Santos et al. (Citation2011). Two other Yazd cultivars including ‘ZT-Yazd’ and ‘ZS-Yazd’ were placed in other groups. As well as, three cultivars in the third group had different origins. In fact, in some groups, there was no correlation between grouping and the geographical origin of cultivars. This lack of correlation has been reported by Erfani et al. (Citation2014). It seems that there was no relationship between cultivar origin and tolerance to stress.
Conclusion
Pomegranate is a drought-tolerant tree that can grow in arid and semi-arid regions. This tree can also be grown on the edge of the desert (Aseri et al., Citation2008). Drought tolerant pomegranate cultivars can adapt to deficit irrigation strategies better than other cultivars without any negative effect on physiological and morphological properties (Parvizi et al., Citation2016). The study of some physiological parameters such as EL, proline content and antioxidant enzymes can be used as a good criterion for selecting drought-tolerant cultivars. Therefore, it can be concluded that ‘ZS-Yazd’ and ‘M-Saveh’ compared to other cultivars were more tolerant to drought stress, so using these cultivars in drought stress conditions is recommended. ‘Robab’ showed the most susceptibility to drought stress, so the cultivation of this cultivar in areas with severe drought stress is not recommended.
References
- Acosta-Motos, J.R., M.F. Ortuno, A. Bernal-Vicente, P. Diaz-Vivancos, M.J. Sanchez-Blanco, and J.A. Hernandez. 2017. Plant responses to salt stress: Adaptive mechanisms. Agronomy 7:18.
- Ahmad, P., C.A. Jaleel, M.M. Ozooz, and G. Nabi. 2009. Generation of ROS and non-enzymatic antioxidants during abiotic stress in plants. Bot. Res. Inter. 2:11–20.
- Andre, C.M., R. Schafleitner, C. Guignard, M. Oufir, C.A. Alvarado-Aliaga, G. Nomberto, L. Hoffmann, J.F. Hausman, D. Evers, and Y. Larondelle. 2009. Modification of the health-promoting value of potato tubers field grown under drought stress: Emphasis on dietary antioxidant and glycol alkaloid contents in five native Andean cultivars (Solanum tuberosum L.). J. Agric. Food Chem. 57:599–609. doi: 10.1021/jf8025452.
- Aseri, G.K., N. Jain, J. Panwar, A.V. Rao, and P.R. Meghwal. 2008. Biofertilizers improve plant growth, fruit yield, nutrition, metabolism and rhizosphere enzyme activities of pomegranate (Punica granatum L.) in Indian Thar Desert. Sci. Hort. 117:130–135. doi: 10.1016/j.scienta.2008.03.014.
- Bagri, P., M. Ali, V. Aeri, M. Bhowmik, and S. Sultana. 2009. Antidiabetic effect of Punica granatum flowers: Effect on hyper lipidemia pancreatic cells lipid peroxidation and antioxidant enzymes in experimental diabetes. Food Chem. Toxicol. 47:50–54. doi: 10.1016/j.fct.2008.09.058.
- Bates, L.S., R.P. Waldren, and I.D. Teare. 1973. Rapid determination of free proline for water stress studies. Plant Soil 39:205–207. doi: 10.1007/BF00018060.
- Behzadi Shahrbabaki, H. 1998. Genetic diversity of pomegranate genotypes in Iran, p. 265. Tehran, Iran: Publication of Agricultural Education.
- Blokhina, O., E. Virolainen, and K.V. Fagerstedt. 2003. Antioxidants, oxidative damage and oxygen deprivation stress: A Review. Ann. Bot. 91:149–179. doi: 10.1093/aob/mcf118.
- Blum, A., and A. Ebercon. 1981. Cell membrane stability as a measure of drought and heat tolerance in wheat. Crop Sci. 21:43–47. doi: 10.2135/cropsci1981.0011183X002100010013x.
- Borochov-Neori, H., S. Judeinstein, E. Tripler, M. Harari, A. Greenberg, I. Shomer, and D. Holland. 2009. Seasonal and cultivar variations in antioxidant and sensory quality of pomegranate (Punica granatum L.) fruit. J. Food Compos. Anal. 22:189–195. doi: 10.1016/j.jfca.2008.10.011.
- Cheruth, A.J., P. Manivannan, A. Wahid, M. Farooq, H. Al-Juburi Somasundaram, and R. Panneerselvam. 2009. Drought stress in plants: A review on morphological characteristics and pigments composition. Int. J. Agric. Biol. 11:100–105.
- Doorenbos, J., and W.H. Pruitt. 1977. Crop water requirements. FAO Irrigation and Drainage, paper No. 24, Romme, Italy.
- Erfani, J., H. Abdollahi, and M.R. Fattahi Moghadam. 2014. Evaluation of genetic diversity of some pear genotypes and species using morphological traits. Iranian Hortic. Sci. J. 1:11–21.
- Fangmeier, A., S. Brunschon, and H.J. Jager. 1994. Time course of oxidant stress bio-markers in flag leaves of wheat exposed to ozone and drought stress. New Phytol. 126:63–69. doi: 10.1111/j.1469-8137.1994.tb07530.x.
- Feng, K., J. Yu, C. Yuan, M. Ruan, R. Wang, Q. Ye, G. Zhou, Z. Li, Z. Yao, Y. Yang, et al. 2016. The SOD gene family in tomato: Identification, phylogenetic relationships, and expression patterns. Front. Plant Sci. 7:1279. doi: 10.3389/fpls.2016.01279.
- Foyer, C.H., and G. Noctor. 2005. Oxidant and antioxidant signalling in plants: Are-evaluation of the concept of oxidative stress in a physiological context. Plant Cell Environ. 28:1056–1071. doi: 10.1111/j.1365-3040.2005.01327.x.
- Ghaderi-Ghahfarokhi, M., M. Barzegar, and M. Nabil. 2016. Geographical discrimination of Iranian pomegranate cultivars based on organic acids composition and multivariate analysis. J. Agr. Sci. Tech. 18:1221–1232.
- Gholami, M., M. Rahemi, and S. Rastegar. 2012. Use of rapid screening methods for detecting drought tolerant cultivars of fig (Ficus carica L.). Sci. Hortic. 143:7–14. doi: 10.1016/j.scienta.2012.05.012.
- Giancarla, V., E.S. Madosa, R. Umalan, and S. Ciulca. 2012. Evaluation of some indirect indices to identify drought tolerance in barley. J. Hortic. Forest. Biotechnol. 16:239–241.
- Giannopolities, C.N., and S.K. Rise. 1977. Superoxide dismutases. I. Occurrence in higher plants. Plant Physiol. 59:304–309.
- Hasani Moghadam, A., M. Esna Aashari, and A. Rezaei Nejad. 2015. Effect of drought stress on some physiological characteristics in six commercial Iranian pomegranate (Punica granatum L.) cultivars. Plant Prod. Tech. 15:1–11.
- He, F., M. Sheng, and M. Tang. 2017. Effects of rhizophagus irregularis on photosynthesis and antioxidative enzymatic system in robinia pseudoacacial. Under drought stress. Front. Plant Sci. 8:183. doi: 10.3389/fpls.2017.00183.
- Holland, D., K. Hatib, and I. Bar-Yaakov. 2009. Pomegranate: Botany, horticulture, breeding. Hortic. Rev. 35:127–191.
- Jain, S.M., J.M. Al-Khayri, and D.V. Johnson. 2011. Date palm biotechnology, p. 743. Springer Science and Business Media, Heidelberg, London, New York.
- Jinying, L., L. Min, M. Yongmin, and S. Lianying. 2007. Effects of vesicular arbuscular mycorrhizae on the drought resistance of wild jujube (Zizyphs spinosus Hu) seedlings. Front. Agric. China 1:468–471. doi: 10.1007/s11703-007-0077-9.
- Kafi, M., A. Borzooei, M. Salehi, A. Kamandi, A. Masoumi, and J. Nabati. 2010. Physiology of environmental stresses in plants, p. 502. Jihad Daneshghahi of Mashhad Press, Iran.
- Khattab, M., A.E. Shaban, A.H. El-shrief, and A.S. El-Deen Mohamed. 2011. Growth and productivity of pomegranate trees under different irrigation levels. III: Leaf pigments, proline and mineral content. J. Hortic. Sci. Ornam. Plant 3:265–269.
- Mahajan, S., and N. Tuteja. 2005. Cold, salinity and drought stresses: An overview. Arch. Biochem. Biophys. 444:139–158. doi: 10.1016/j.abb.2005.10.018.
- Medrano, H., J.M. Escolana, J. Bota, J. Gulias, and J. Flexas. 2002. Regulation of photosynthesis of 3C plants in response to progressive drought: Stomatal conductance as a reference parameter. Ann. Bot. 88:895–905. doi: 10.1093/aob/mcf079.
- Miguel, M.G., M.A. Neves, and M.D. Antunes. 2010. Pomegranate (Punica granatum L.): A medicinal plant with myriad biological properties- A short review. J. Med. Plants Res. 4:2836–2847.
- Mirzaei, J., and A. Karamshahi. 2015. Effects of drought stress on growth and physiological characteristics of Pistacia atlantica seedlings. J. Wood Forest Sci. Technol. 22: 31-43.
- Mittler, R. 2006. Abiotic stress, the field environment and stress combination. Trends Plant Sci. 11:15–19. doi: 10.1016/j.tplants.2005.11.002.
- Parvizi, H., A.R. Sepaskhah, and S.H. Ahmadi. 2016. Physiological and growth responses of pomegranate tree (Punica granatum (L.) cv. Rabab) under partial root zone drying and deficit irrigation regimes. Agric. Water Manag. 163:146–158. doi: 10.1016/j.agwat.2015.09.019.
- Pourghayoumi, M.R., D. Bakhshi, M. Rahemi, A.A. Kamgar-Haghighi, and A. Aalami. 2017. The physiological responses of various pomegranate cultivars to drought stress and recovery in order to screen for drought tolerance. Sci. Hortic. 217:164–172. doi: 10.1016/j.scienta.2017.01.044.
- Premachandra, G.S., H. Saneoka, K. Fujita, and S. Ogata. 1992. Leaf water relations osmotic adjustment, cell membrane stability, epicuticular wax load and growth as affected by increasing water deficits in sorghum. J. Exp. Bot. 43:1569–1576. doi: 10.1093/jxb/43.12.1569.
- Rad, M.H., M.R. Asghari, and M.H. Asare. 2015. The effects of drought stress on growth, yield and fruit quality of pomegranate (Punica granatum L.) cv. Rababe-Niriz under dry climate condition. Seed Plant Prod. 31:75–90(In Persian).
- Roby, G., J.F. Harbertson, D.A. Adams, and M. Matthews. 2004. Berry size and vine water deficits as factors in wine grape composition: Anthocyanins and tannins. Aust. J. Grape Wine Res. 10:100–107. doi: 10.1111/j.1755-0238.2004.tb00012.x.
- Rodriguez, P., C.D. Mellisho, W. Conejero, Z.N. Cruz, M.F. Ortuno, A. Galindo, and A. Torrecillas. 2012. Plant water relations of leaves of pomegranate trees under different irrigation conditions. Environ. Exper. Bot. 77:19–24. doi: 10.1016/j.envexpbot.2011.08.018.
- Santos, A.R.F., A.M. Ramos-Cabrer, M.B. Diaz-Hernandez, and S. Pereira-Lorenzo. 2011. Genetic variability and diversification process in local pear cultivars from northwestern Spain using microsatellites. Tree Genet. Genomes 7:1041–1056. doi: 10.1007/s11295-011-0393-3.
- Selahvarzi, Y., Z. Zamani, R. Fatahi, and A.Z. Talaei. 2017. Effect of deficit irrigation on flowering and fruit properties of pomegranate (Punica granatum cv. Shahvar). Agric. Water Manag. 192:189–197. doi: 10.1016/j.agwat.2017.07.007.
- Sircelj, H., M. Tausz, D. Grill, and F. Batic. 2007. Detecting different levels of drought stress in apple trees (Malus domestica Borkh.) with selected biochemical and physiological parameters. Sci. Hortic. 113:362–369. doi: 10.1016/j.scienta.2007.04.012.
- Tavousi, M., F. Kaveh, A. Alizadeh, H. Babazadeh, and A. Tehranifar. 2015. Effect of salinity and deficit irrigation on quantity and quality of pomegranate (Punica granatum L.). J. Mater. Environ. Sci. 6:1975–1980.
- Wang, X., Y. Gao, Q. Wang, M. Chen, X. Ye, D. Li, X. Chen, L. Li, and D. Gao. 2019. 24-Epibrassinolide-alleviated drought stress damage influences antioxidant enzymes and autophagy changes in peach (Prunus persica L.) leaves. Plant Physiol. Biochem. 135:30–40. doi: 10.1016/j.plaphy.2018.11.026.
- Waseem, M., M. Ali Asghar, M.A. Tahir, M. Nadeem, A.T. Ayub, R. Ahmad, and M. Hussain. 2011. Mechanism of drought tolerance in plant and its management through different methods. J. Agric. Sci. 5:10–25.
- Wu, Q.S., and R.X. Xia. 2006. Arbuscular mycorrhizal fungi influence growth, osmotic adjustment and photosynthesis of citrus under well-watered and water stress conditions. J. Plant Physiol. 163:417–425. doi: 10.1016/j.jplph.2005.04.024.
- Xoconostle-Cazares, B., F.A. Ramirez-Ortega, L. Flores-Elenes, and R. Ruiz-Medrano. 2010. Drought tolerance in crop plants. American J. Plant Physiol. 5:241–256. doi: 10.3923/ajpp.2010.241.256.
- Xu, H., D.K. Biswas, W.D. Li, S.B. Chen, L. Zhang, G.M. Jiang, and Y.G. Li. 2007. Photosynthesis and yield responses of ozone-polluted winter wheat to drought. Photosynthetica 45:582–588. doi: 10.1007/s11099-007-0100-7.
- Zhang, J., and M.B. Kirkham. 1996. Antioxidant responses to drought in sunflower and sorghum seedlings. New Phytol. 132:361–373. doi: 10.1111/j.1469-8137.1996.tb01856.x.
- Zhang, J., S. Cui., J. Li, and M.B. Kirkham. 1995. Protoplasmic factors, antioxidants responses, and chilling resistance in maize. Plant Physiol. Biochem. 33: 567-575.
- Zhang, X.Z. 1992. The measurement and mechanism of lipid peroxidation and SOD, POD and CAT activities in biological system.), p. 208–211. In: X.Z. Zhang (ed.). Research methodology of crop physiology. Beijing: Beijing Press.