ABSTRACT
Effect of canopy position on rind biochemical properties of ‘Marsh’ grapefruit during postharvest non-chilling cold storage was evaluated on fruit harvested from different orchard locations. Inside canopy (IC) and outside canopy (OC) fruits were harvested at commercial maturity from two commercial farms located at KwaZulu-Natal (KZN) and Limpopo (LMP), South Africa, during two seasons. Rind biochemical analyses including color indices, total phenolic concentration, and sugars were performed on harvested grapefruit after Weeks 0, 3, 6, and 9 of cold storage at 7.5 ± 0.5°C. Canopy position showed a high significant (p < .001) effect on rind biochemicals while significant (p < .05) influence of orchard locations was observed on the same parameters. Reducing sugars were more concentrated in IC fruit harvested from both orchard location than OC fruit at Weeks 0 and 3, but inverse results occurred at Weeks 6 and 9. Orchard location significantly (p < .001) influenced vitamin C (14.4, 14.2, 14.4, 14.8 g kg−1) and (12.3, 16.1, 15.9, 14.6 g kg−1) of fruit rind at Weeks 0, 3, 6, and 9 from KZN and LMP, respectively. This study revealed that canopy position can influence rind biochemical properties of ‘Marsh’ grapefruit during non-chilling postharvest cold storage. However, further studies are required to formulate and provide the citrus industries with adequate advice.
Introduction
‘Marsh’ grapefruit (Citrus paradisi Macfadyen) is an economically important citrus cultivar grown in South Africa and is mostly cultivated for exportation to international markets. The fruit is globally grown for its eminent medicinal and nutritional values (Kiani and Imam, Citation2007). Many countries including South Africa, the United States of America, and Israel contribute largely to the availability of the fruit at international markets (Vacante, Citation2009). However, incidence of rind physiological disorder at non-chilling temperatures such as rind pitting causes severe economic losses to fruit growers because the disorder affects the sub-epidermal cells of the fruit surface which invariably compromises the external appearance of the fruit (Agustí et al., Citation2001; Alférez et al., Citation2003, Citation2005; Lafuente and Sala, Citation2002), thus reducing consumer acceptability of fruit in the market. Fruit appearance plays a crucial role in the fresh fruit market because it influences consumer’s buying decision (Pathare et al., Citation2013). That is, a fruit without rind pitting disorder is largely preferred compared to fruit with disorder. More importantly, rind-disordered fruit is often rejected by consumers in the market even though its internal quality is not compromised by the disorder (Agustí et al., Citation2001). Usually, biotic and abiotic factors during preharvest and postharvest life of a fruit induce rind disorders, but it has been problematic to associate a definite inductive factor to a postharvest rind disorder since different factors can induce similar symptomology in fruit (Alférez and Burns, Citation2004). Despite various scientific studies by researchers to understand the primary factor triggering the disorder, the subject is still unknown. Meanwhile, rind biochemical properties such as carbohydrates and phytochemicals such as total phenolic and total flavonoids concentrations, and vitamin C have been reported to play critical roles in the response of citrus fruit rind to postharvest physiological stresses (Cronje, Graham H. Barry, et al. 2011; Khalid et al., Citation2012; Magwaza, Citation2013). These stresses manifest as various types of physiological disorders on different citrus cultivars under various postharvest cold storage, such as peel or rind pitting (Alférez and Burns, Citation2004), rind breakdown (Magwaza et al., Citation2013, Citation2013a, Citation2013b), and chilling injury (Alférez et al., Citation2005). Similarly, canopy position, which determines varying intensity of sunlight and other abiotic factors reaching different positions of tree canopy, affects the physiological activities and biochemical properties of fruit rind (Cronje et al., Citation2011; Magwaza et al., Citation2014a). Canopy positions, exposure to high (outside) or low (inside) sunlight in fruit tree canopy, have been found to affect the biochemical properties of citrus fruit rinds such as ‘Nules clementine’ mandarin fruit, thereby influencing its outward appearance (Cronje et al., Citation2013; Magwaza, Citation2013; Olarewaju et al. Citation2017). Therefore, the possible link among canopy position, rind soluble sugars, and emergence of rind breakdown of mandarin fruit have been suggested (Cronje, Graham H. Barry, et al. Citation2011). However, limited research has been conducted to investigate the effect of canopy position on rind biochemical properties of ‘Marsh’ grapefruit. In view of this, the aim of this study was to investigate the effect of canopy position and orchard location on the biochemical properties of ‘Marsh’ grapefruit rind during postharvest cold storage.
Materials and Methods
Reagents and Standards
All chemicals used in this study were procured from Sigma-Aldrich Company Ltd. (Dorset, UK). The chemicals included acetone, 2,6 dichloroindophenol dye, 2,2-diphenyl-1-picrylhydrazyl (DPPH), ethanol (HPLC grade), folin-ciocalteu reagent, gallic acid, metaphosphoric acid (MPA), quercetin, sodium carbonate, sodium hydroxide (NaOH), sugars standards (sucrose, D-glucose, and D-fructose), and vitamin C. Phenomenex® column (Rezex RCM – Monosaccharide) was used in HPLC for sugar analyses. Ultra-pure water from Milli-Q Integral Water Purification System (Merck Millipore corporation, Billerica, MA, USA; σ = 18 M Ω cm−1) was used.
Plant Materials
‘Marsh’ grapefruits budded on ‘Troyer’ Citrange ((Poncirus trifoliata (L.) Raf.) × (C. sinensis)) and x639 ((Poncirus trifoliata (L.) Raf.) × (C. reshni)) rootstocks were used for the experiment. The trees were planted in 1993 on Bolton Citrus Farm, KwaZulu-Natal (KZN) (31° 34′ 44″ S, 28° 44′ 59″ E) and Olifant Rivers Farm, Limpopo (LMP) (32° 75′ 28″ S, 35° 89′ 31″ E) provinces, respectively. Three fruits per canopy position were harvested from a height of 1–2 m from 50 uniform sized trees, from each farm, at commercial maturity during 2015 and 2016 sessions. The canopy positions were: inside canopy (IC), fruit exposed to less than 80% of full sunlight; and outside canopy (OC), fruit exposed to 90–100% of full sunlight, of a fruit tree according to Cronje et al. (Citation2011). The rainfall (mm), relative humidity (%), and maximum and minimum temperature (°C) registered during the growing season in KZN and LMP provinces are displayed in , respectively. After harvesting, fruits were transported within 48 h at ambient temperature in ventilated cartons to the horticultural research laboratory where fruits were washed and sorted for blemishes and fruit damage. Upon arrival at the laboratory, fruits were left for 24 h at room temperature (20 ± 1ºC) to equilibrate after which each fruit was labeled, weighed, and transferred into cold storage (7.5 ± 0.5ºC) for 9 weeks. Ten individual fruits were analyzed at 3 weeks interval for 9 weeks (Weeks 0, 3, 6, and 9).
Figure 1. Effect of canopy position (inside canopy (IC) and outside canopy (OC)) and orchard location (KwaZulu-Natal (KZN) and Limpopo (LMP)) on luminosity (a), greenness (b), yellowness (c), and color index (d) of ‘Marsh’ grapefruit rind harvested over two seasons during postharvest non-chilling storage (Weeks 0, 3, 6, and 9)
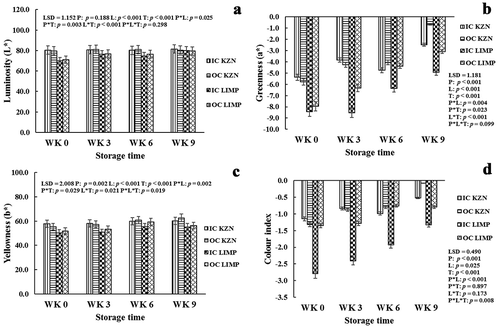
Sample Preparation for Analyses of Biochemicals
The rind was manually peeled off the fruit using table knife, snap frozen in liquid nitrogen, and stored at −80°C before freeze-drying over a period of 3 days using Virtis Benchtop freeze drier system (ES Model, SP Industries Inc., Warmister, USA) at 0.015 kPa and −75°C. Dried samples were then milled into a fine powder using pestle and mortar and stored at −20°C for further analyses.
Determination of Rind Biochemical Properties
Rind biochemical properties including total carotenoids, radical-scavenging activities, chlorophyll a, chlorophyll b, soluble sugars, total phenolics, and total flavonoids concentrations were determined as described elsewhere (Olarewaju et al. 2017). Rind color of the fruit was measured as described by Olarewaju et al. (2018).
Extraction Procedure for Determination of Vitamin C
Extraction was performed using a methodology explained elsewhere (Olarewaju et al. 2017). A lyophilized sample of 0.15 g was dissolved in a 3% aqueous MPA (5 mL). The test tube containing the mixture was incubated on ice-cubes for 6 min. The extract was centrifuged using GenVac ® (SP Scientific, Genevac LTD., Suffolk, UK) for 20 min while the supernatants were cold-preserved at −20°C for future purposes.
Determination of Vitamin C
Vitamin C was quantified according to Olarewaju et al. (2017). Briefly, supernatant (0.5 mL) was measured into a test tube followed by the addition of 2.5 mL of 0.005% of 2,6 dichloroindophenol dye. The mixture was incubated in the dark for 10 min at room temperature. Absorbance was read in triplicate at 515 nm against a 3% MPA solution in the dark using Ultraspec UV-1800 Spectrophotometer (Shimadzu Scientific Instruments, Inc., Columbia, USA) and the amount of vitamin C was calculated from a linear standard curve (0.00–100.00 g kg−1; R2 = 0.96).
Statistical Analysis
Experiments were laid out using a completely randomized design (CRD) with individual fruit as replicate. All statistical analyses were performed using GenStat® 18th Edition (VSN International, Hemel Hempstead, UK). Data were subjected to analysis of variance (ANOVA) with canopy position, season, orchard location, and cold storage time as factors. Season two (2016) data from LMP were treated as missing values, and least significant difference (LSD) at 5% level was considered significant.
Results and Discussion
Effect of Canopy Position and Orchard Location on Rind Color and Pigments
The analysis of variance revealed that canopy position had a highly significant (p < .001) effect on the greenness (a*), yellowness (b*), and color index (CI), while the effect on luminosity of the rind during the entire period of cold storage (L*) was not significant (p = .118). Orchard location showed significant (p < .05) effect on rind color indices during postharvest cold storage. Similarly, canopy position and orchard location significantly (p < .05) affected rind pigments (chlorophylls a and b, and total carotenoid content) of the fruit during the period of cold storage. The a*, CI, and chlorophylls a and b followed a similar pattern during the period of cold storage except at Week 6 where inverse results occurred. These parameters which measure the disappearance of chlorophylls a and b, and hence, greenness (a*) during cold storage showed the consistent transformation of the rind color from green to yellow, a color that encourages purchase of the fruit at the markets. Although not statistically significant, OC fruits (−5.80 and – 4.27) from KZN were greener than IC fruits (−5.38 and −3.86) at Week 0 and after Week 3 of cold storage, respectively (). However, inverse results were obtained after Weeks 6 and 9 of cold storage, with IC fruits (−4.75 and −2.50) been significantly greener in color than OC fruits (−4.06 and −0.71), respectively (). The inconsistent nature of these results could be attributed to color measurements being taken at different points along the equator axis of the fruit. For fruit from LMP, a progressive decline in greenness occurred during cold storage. IC fruits (−8.44, −8.54, −6.37, and −4.96) were significantly greener than OC fruits (−7.96, −6.33, −4.38, and – 3.10) at Weeks 0, 3, 6, and 9, respectively (), while OC fruits were yellower (). This concurred with report by Cronje et al. (Citation2013) where OC fruit developed a more intense orange color (for ‘Nules Clementine’ mandarin) than IC fruit after the color break. This indicates that sunlight contributes to rind color development of the fruit. Like a* of the fruit rind, a significantly higher chlorophyll a pigment occurred in OC fruit (−6.00, −6.53, and −5.49 g kg−1) than in IC fruit (−5.30, −5.00 and −4.51 g kg−1) from KZN at Week 0 and after Weeks 3 and 6, respectively (). Also, lower chlorophyll a pigment occurred in OC fruit (−5.08, −2.73, and −1.98 g kg−1) than in IC fruit (−5.63, −5.01, and −3.02 g kg−1) from LMP at Week 0 and after Weeks 3 and 9 of cold storage, respectively (). These results further indicated that exposure of fruit to sunlight encourages better rind coloration. Generally, the disappearance of the chlorophyll is primarily due to the synthesis of carotenoids (Cronje et al., Citation2013). Total carotenoids, the pigment responsible for the development of the attractive color (yellow) of the rind that encourages consumer purchase of grapefruit, followed an inconsistent pattern during the period of cold storage (). However, significantly higher results occurred in IC fruit (10.86 and 9.25 g kg−1) than in OC fruit (8.37 and 6.76 g kg−1) from KZN at Weeks 0 and 9, respectively (). IC fruits (11.13 and 7.76 g 158 kg−1) from LMP were significantly higher than OC fruits (6.78 and 4.14 g kg−1) at Weeks 3 and 9 after cold storage, respectively (). This is contrary to the results presented in the literature by Cronje et al. (Citation2013) who reported lower total carotenoid concentration of IC fruit than OC fruit. However, this could be because of the differences in eventual color of the fruit investigated.
Figure 2. Effect of canopy position (inside canopy (IC) and outside canopy (OC)) and orchard location (KwaZulu-Natal (KZN) and Limpopo (LMP)) on rind chlorophyll a (a), chlorophyll b (b), and total carotenoid content (c) of ‘Marsh’ grapefruit harvested over two seasons during postharvest non-chilling storage (Weeks 0, 3, 6, and 9)
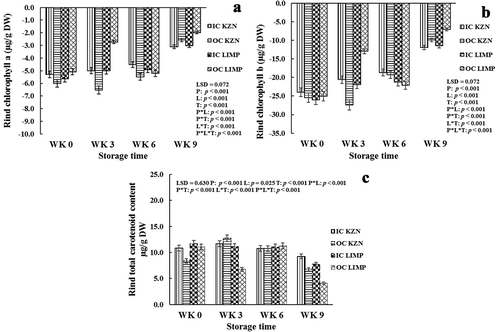
Although not statistically significant, chlorophyll a, b, and total carotenoid of OC fruit became higher than IC fruit from LMP at Week 6 (). The reason for the deviation of trend is not clear.
Effect of Canopy Position and Orchard Location on Non-structural Carbohydrates
Sucrose, glucose, and fructose were the three main non-structural carbohydrates evaluated. The carbohydrates were significantly (p ≤ 0.05) affected by canopy position and orchard location. Overall, IC fruits were significantly higher than OC fruits during the entire period of cold storage with some deviations (). In the case of fruit from KZN, reducing sugars (fructose and glucose) were more concentrated in IC fruit (284.5 and 205.9 g kg−1) than in OC fruit (238.9 and.4 g kg−1), respectively, at Week 0 and barely increased during the period of cold storage. This was in contrast with Magwaza (Citation2013) who reported higher concentration of the sugars in ‘Nules Clementine’ mandarin rind from OC than IC but agreed with the results obtained for fruit harvested from LMP, which could indicate the influence of orchard location. These reducing sugars (fructose and glucose) became significantly more concentrated in OC fruit (263.0 and 91.9 g kg−1) than in IC fruit (222.9 and 177.5 g kg−1), respectively, from LMP region after Week 9 of cold storage (). The contrasting results obtained for IC and OC fruits from both orchard locations indicated the influence of either agro-climatic conditions or rootstock used in the respective orchards from which fruits were harvested. Generally, the non-reducing sugar (sucrose) declined regardless of orchard location over the period of cold storage in fruit from both canopy positions. This agreed with the declining trends in sucrose concentration reported in literature for ‘Nules Clementine’ mandarin stored at 7.5°C (Magwaza, Citation2013), ‘Navelate’ (stored at 2°C), Pinalate’ (stored at 12°C) oranges (Holland et al., Citation2002), and peach fruit stored at 5°C (Yu et al., Citation2016). While the difference in concentration of sucrose in IC and OC fruits from both regions is not statistically significant at Week 0, concentration of sucrose in IC fruit, 74.4 and 55.4 g kg−1, became significantly higher than OC fruit, 21.7 and 26.2 g kg−1, from KZN at Weeks 3 and 6 of cold storage, respectively ().
Figure 3. Effect of canopy position (inside canopy (IC) and outside canopy (OC)) and orchard location (KwaZulu-Natal (KZN) and Limpopo (LMP)) on rind fructose (a), glucose (b), and sucrose (c) of ‘Marsh’ grapefruit harvested over two seasons during postharvest non-chilling storage (Weeks 0, 3, 6, and 9)
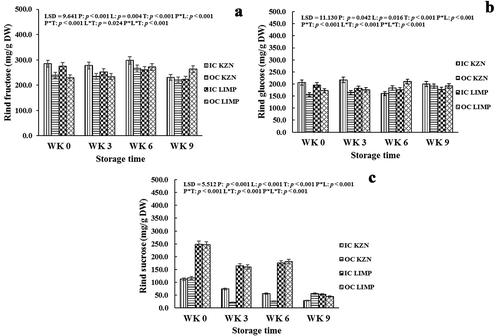
Previous studies have indicated that non-structural carbohydrates such as sucrose, fructose, and glucose are important sources of energy contributing to rind quality (Cai et al., Citation2015) and fruit stress resistance (Der Agopian et al., Citation2011), thereby neutralizing oxidative challenges during abiotic stress in plants (Keunen et al., Citation2013). Further studies have also revealed that sugars play a role in the biosynthesis of phytochemicals such as vitamin C which is a good antioxidant that could protect plants against physiological disorder (Wei et al., Citation2017). Hence, it could be deduced from this study that the high concentration of sugars contributed toward the inhibition of rind pitting development on the fruit during the period of cold storage.
Effect of Canopy Position and Orchard Location on Phytochemicals, Vitamin C, and Radical-scavenging Activities
shows the effect of canopy position and orchard location on phytochemicals, vitamin C, and radical-scavenging activities of grapefruit rind at Week 0 and after Weeks 3, 6, and 9 of non-chilling cold storage. Although orchard location showed significant (p < .05) effects on phytochemicals, vitamin C, and radical-scavenging activities, canopy position had no significant effect on vitamin C (p = .917; ). On the contrary, a higher concentration of vitamin C was reported in OC fruit than in IC fruit by Magwaza et al. (Citation2014b) and Magwaza et al. (Citation2014c) which was suggested to increase the fruit tolerance to rind breakdown of ‘Nules Clementine’ mandarin. Vitamin C is a powerful antioxidant which constitutes a high amount of the antioxidant capacity of citrus fruit (Sdiri et al., Citation2012). In this study, vitamin C was higher in fruit from KZN, 14.41 g kg−1, than fruit from LMP, 12.64 g kg−1 at Week 0 but became lower in fruit from KZN, 14.19 and 14.43 g kg−1, than LMP, 16.13 and 15.85 g kg−1, after cold storage at Weeks 3 and 6, respectively (). The postharvest storage temperature could have influenced the response of fruit rind in the production of vitamin C concentration as observed in fruits from orchard locations. Santos and Silva (Citation2008) suggested that vitamin C is very sensitive and can easily vanish at the exposure of food item to an adverse storage condition. This may explain the inconsistent trends of vitamin C contents of the fruit over the period of storage even though the result showed an overall high vitamin C content.
Figure 4. Effect of canopy position (inside canopy (IC) and outside canopy (OC)) and orchard location (KwaZulu-Natal (KZN) and Limpopo (LMP)) on rind total phenolic concentration (a), total flavonoid concentration (b), vitamin C (c), and radical scavenging activities (d) of ‘Marsh’ grapefruit harvested over two seasons during postharvest non-chilling storage (Weeks 0, 3, 6, and 9)
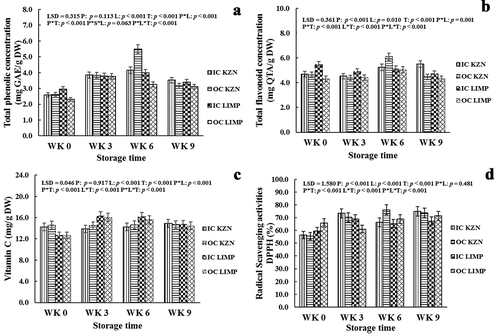
The effects of canopy position on total flavonoid concentration () and radical-scavenging activities () were highly significant (p < .001). The concentration of vitamin C was significantly higher in fruit from KZN (14.41 g kg−1) than in fruit from LMP (12.64 g kg−1) at Week 0 (). However, a reverse trend occurred postharvest with higher concentration occurring in fruit from LMP (16.13 and 15.85 g kg−1) than in fruit from KZN (15.85 and 14.43 g kg−1) at Weeks 3 and 6, respectively. This suggested that storage temperature affected the biological activities leading to various production levels of vitamin C after cold storage at Weeks 3 and 6. Total phenolic and total flavonoid concentrations followed similar trends with significant higher concentrations in IC fruit (2.96 g kg−1 GAE and 5.45 QTE g kg−1) than OC fruit (2.31 GAE g kg−1 and 4.31 QTE g kg−1) from LMP at Week 0 while difference in concentration of IC and OC fruit from KZN was not significant (). The former was in accordance with Magwaza et al. (Citation2014b) who reported a higher concentration of total phenolics in the rind of bagged and IC ‘Nules Clementine’ mandarin fruit than OC fruit but contradicts McDonald et al. (Citation2000) who reported higher levels of phenols and flavanols in the rind of OC ‘Marsh’ grapefruit than IC fruit. The similarity in the result of total phenolic and flavonoid concentrations was not surprising since flavonoid is a major phenolic group contributing to the total phenolic concentration in the rind of a fruit (Fawole et al., Citation2012). The role of sunlight as a contributing factor to the synthesis of phenolics was suggested by Awad et al. (Citation2001). This could indicate that fruits from both canopy positions received enough sunlight during their preharvest life for the synthesis of total phenolic concentrations which could have acted as a defense mechanism against the possible incidence of physiological disorder such as rind pitting. Furthermore, it was expected that total phenolic and total flavonoid concentrations would be higher in the OC fruit since the photosynthetically active radiation in the OC is known to stimulate the synthesis of phenylalanine ammonialyase which induces the production of phenols such as phytoalexins (Ben Yehoshua et al., Citation1992). This supports the findings by Hamadziripi et al. (Citation2014) who reported a significantly higher total phenolics and antioxidant capacity in the OC than IC apple fruit (rind and flesh). This result was consistent for ‘Granny Smith,’ ‘Starking,’ and ‘Golden delicious’ cultivars that were used for the study. Hence, these results suggest that OC fruit tends to resist environmental stress than the IC fruit counterpart.
As previously reported in the literature (Contreras-Oliva et al., Citation2011; Del Caro et al., Citation2004), the concentration of total phenolics and total flavonoids generally increased during the postharvest cold storage of the fruit (). These increased concentrations due to stimulation of phenylalanine ammonialyase activity during cold storage could be responsible for the ability of the grapefruit rind to prevent the incidence of postharvest stress such as rind pitting. At Week 6, the concentration of total phenolics and total flavonoids of OC fruit (5.49 GAE g kg−1 and 6.08 QTE g kg−1) from KZN became significantly higher than IC fruit (4.16 GAE g kg−1 and 5.24 g kg−1) as shown in , respectively. This sudden change in trend was unexpected and possible reason for such is unclear. Using radical-scavenging activities of 50% as a basis for good activity, good radical-scavenging activities were exhibited by IC and OC fruit from both orchard locations (). Although not significant at Weeks 0 and 9, the mean values of IC fruit (56.38% and 74.96%) were higher than OC fruit (55.56% and 73.86%) from KZN while OC fruit (65.90% and 71.49%) were higher than IC fruit (59.49% and 67.32%) from LMP. At Week 3, IC fruits (68.87%) were significantly higher than OC fruits (61.04%) from LMP while IC fruits (68.35%) were significantly lower than OC fruits (76.13%) from KZN at Week 6 (). Previous studies have revealed that the absence or presence of antioxidant species plays a role in the incidence of different postharvest physiological rind disorders such as non-chilling rind pitting in ‘Navelate’ oranges (Cajuste and Lafuente, Citation2007). In retrospect, the lack of physiological rind disorders in IC and OC fruits from both orchard locations suggests that the defense mechanisms of the fruit to stress were sustained during the period of cold storage.
Correlation Analyses
Correlation tests indicated significantly (p < .05) high relationship between certain investigated parameters and is presented in . A negatively strong relationship was revealed between sucrose and luminosity (r = −0.845) while moderate but negative correlations related sucrose with 268 greenness (r = −0.537), yellowness (r = −0.450), chlorophyll b (r = −0.503), and total carotenoids 269 (r = 0.465). Correlation between reducing sugars (fructose and glucose) was positively strong (r = 0.869) while sucrose had moderate but positive correlation with fructose (r = 0.515) and glucose (r = 0.505) (). Furthermore, sucrose shared a moderate but negative correlation with total phenolic concentration (r = −0.533), vitamin C (r = −0.631), radical-scavenging activities (r = −0.627), and positive correlation with total flavonoid concentration (r = 0.590). Strong negative correlations were found between total flavonoids and vitamin C (r = −0.708), radical-scavenging activities and total carotenoids (r = −0.731), while positive correlations existed between total flavonoids and fructose (r = 0.838), radical-scavenging activities and chlorophyll b (r = 0.744). Radical scavenging activities had poor correlations with vitamin C (r = 0.414), total phenolic (r = 0.369), and flavonoid (r = −0.351) concentrations.
Table 1. Climatological data registered during the growing seasons in KwaZulu-Natal (KZN) and Limpopo (LMP) provinces (Source: South African weather services)
Table 2. Pearson correlation coefficient matrix between biochemical properties measured in ‘Marsh’ grapefruit rind measured in 2015 and 2016 seasons
In conclusion, this study highlighted the effect of canopy position on rind biochemical properties of grapefruit harvested from different orchard location and stored at non-chilling temperature. The nonappearance of rind pitting during the study could suggest that the biochemical properties of the fruit rind were in their optimal levels to defend the fruit against environmental stress. Generally, there was no clear trend in the role of canopy position among the measured parameters as fruit rind from the two orchard locations responded differently during postharvest cold storage. However, non-reducing sugars of IC and OC fruit from the orchard locations followed a similar pattern from Week 0 to Week 6 of cold storage. Correlation tests showed that sucrose is an important biochemical property of fruit rind which could have a direct or indirect impact on the performances of other biochemical properties. Therefore, the role of sucrose in the defense mechanism of the fruit against non-chilling physiological disorder should not be underrated.
Acknowledgments
The authors are grateful to Dr Paul Cronje for his technical assistance. We appreciate the fruit donations from Bolton Citrus Farm from KwaZulu-Natal, and Unifruitti farm from Limpopo towards the success of this study. We also express our gratitude to the South African Weather Services for providing us with weather information.
Disclosure Statement
The authors have no real or potential conflict of interest to declare.
Additional information
Funding
Literature Cited
- Agustí, M., V. Almela, M. Juan, F. Alferez, F.R. Tadeo, and L. Zacarìas. 2001. Histological and physiological characterization of rind breakdown of “Navelate” sweet orange. Ann. Bot. 88:415–422. doi:10.1006/anbo.2001.1482.
- Alférez, F., and J.K. Burns. 2004. Postharvest peel pitting at non-chilling temperatures in grapefruit is promoted by changes from low to high relative humidity during storage. Postharvest Biol. Technol. 32:79–87. doi:10.1016/j.postharvbio.2003.09.018.
- Alférez, F., J.M. Sala, M.T. Sanchez-Ballesta, M. Mulas, M.T. Lafuente, and L. Zacarias. 2005. A comparative study of the postharvest performance of an ABA-deficient mutant of oranges: I. Physiological and quality aspects. Postharvest Biol. Technol. 37:222–231. doi:10.1016/j.postharvbio.2005.05.010.
- Alférez, F., M. Agusti, and L. Zacarías. 2003. Postharvest rind staining in “Navel” oranges is aggravated by changes in storage relative humidity: Effect on respiration, ethylene production and water potential. Postharvest Biol. Technol. 28:143–152. doi:10.1016/S0925-5214(02)00120-5.
- Awad, M.A., A. de Jager, L.H. van der Plas, and A.R. van der Krol. 2001. Flavonoid and chlorogenic acid changes in skin of “Elstar”and “Jonagold”apples during development and ripening. Sci. Hortic. (Amsterdam). 90:69–83. doi:10.1016/S0304-4238(00)00255-7.
- Ben Yehoshua, S., V. Rodov, J.J. Kim, and S. Carmeli. 1992. Preformed and induced antifungal materials of citrus fruits in relation to the enhancement of decay resistance by heat and ultraviolet treatments. J. Agric. Food Chem. 40:1217–1221. doi:10.1021/jf00019a029.
- Cai, X., H. Wang, and G. Pang. 2015. Flux control analysis of a lactate and sucrose metabolic network at different storage temperatures for Hami melon (Cucumis melo var. saccharinus). Sci. Hortic. (Amsterdam). 181:4–12. doi:10.1016/j.scienta.2014.10.040.
- Cajuste, J.F., and M.T. Lafuente. 2007. Ethylene-induced tolerance to non-chilling peel pitting as related to phenolic metabolism and lignin content in “Navelate” fruit. Postharvest Biol. Technol. 45:193–203. doi:10.1016/j.postharvbio.2007.01.019.
- Contreras-Oliva, A., M.B. Pérez-Gago, L. Palou, and C. Rojas-Argudo. 2011. Effect of insecticidal atmosphere and low dose X-ray irradiation in combination with cold quarantine storage on bioactive compounds of clementine mandarins cv. “Clemenules.”. Int. J. Food Sci. Technol. 46:612–619. doi:10.1111/j.1365-2621.2010.02528.x.
- Cronje, P.J.R., G.H. Barry, and M. Huysamer. 2011. Fruiting position during development of “Nules Clementine” mandarin affects the concentration of K, Mg and Ca in the flavedo. Sci. Hortic. (Amsterdam) 130:829–837. doi:10.1016/j.scienta.2011.09.011.
- Cronje, P.J.R., G.H. Barry, and M. Huysamer. 2013. Canopy position affects pigment expression and accumulation of flavedo carbohydrates of “Nules Clementine” mandarin fruit, thereby affecting rind condition. J. Am. Soc. Hortic. Sci. 138:217–224. doi:10.21273/JASHS.138.3.217.
- Del Caro, A., A. Piga, V. Vacca, and M. Agabbio. 2004. Changes of flavonoids, vitamin C and antioxidant capacity in minimally processed citrus segments and juices during storage. Food Chem. 84:99–105. doi:10.1016/S0308-8146(03)00180-8.
- Der Agopian, R.G., F.H.G. Peroni-Okita, C.A. Soares, J.A. Mainardi, J.R.O. Do Nascimento, B.R. Cordenunsi, F.M. Lajolo, and E. Purgatto. 2011. Low temperature induced changes in activity and protein levels of the enzymes associated to conversion of starch to sucrose in banana fruit. Postharvest Biol. Technol. 62:133–140. doi:10.1016/j.postharvbio.2011.05.008.
- Fawole, O.A., N.P. Makunga, and U.L. Opara. 2012. Antibacterial, antioxidant and tyrosinase-inhibition activities of pomegranate fruit peel methanolic extract. BMC Complement. Altern. Med. 12:1178. doi:10.1186/1472-6882-12-200.
- Hamadziripi, E.T., K.I. Theron, M. Muller, and W.J. Steyn. 2014. Apple compositional and peel color differences resulting from canopy microclimate affect consumer preference for eating quality and appearance. HortScience. 49:384–392. doi:10.21273/HORTSCI.49.3.384.
- Holland, N., H.C. Menezes, and M.T. Lafuente. 2002. Carbohydrates as related to the heat-induced chilling tolerance and respiratory rate of “Fortune” mandarin fruit harvested at different maturity stages. Postharvest Biol. Technol. 25:181–191. doi:10.1016/S0925-5214(01)00182-X.
- Keunen, E., D. Peshev, J. Vangronsveld, W. Van Den Ende, and A. Cuypers. 2013. Plant sugars are crucial players in the oxidative challenge during abiotic stress: Extending the traditional concept. Plant, Cell Environ. 36:1242–1255. doi:10.1111/pce.12061.
- Khalid, S., A.U. Malik, B.A. Saleem, A.S. Khan, M.S. Khalid, and M. Amin. 2012. Tree age and canopy position affect rind quality, fruit quality and rind nutrient content of “Kinnow” mandarin (Citrus nobilis Lour×Citrus deliciosa Tenora). Sci. Hortic. (Amsterdam) 135:137–144. doi:10.1016/j.scienta.2011.12.010.
- Kiani, J., and S.Z. Imam. 2007. Medicinal importance of grapefruit juice and its interaction with various drugs. Nutr. J. 6:33. doi:10.1186/1475-2891-6-33.
- Lafuente, M.T., and J.M. Sala. 2002. Abscisic acid levels and the influence of ethylene, humidity and storage temperature on the incidence of postharvest rindstaning of “Navelina” orange (Citrus sinensis L. Osbeck) fruit. Postharvest Biol. Technol. 25:49–57. doi:10.1016/S0925-5214(01)00162-4.
- Magwaza, L.S. 2013. Non-destructive prediction and monitoring of postharvest quality of citrus fruit. Stellenbosch University.
- Magwaza, L.S., S. Landahl, P.J.R. Cronje, H.H. Nieuwoudt, A.M. Mouazen, B.M. Nicolaï, L.A. Terry, and U.L. Opara. 2014a. The use of Vis/NIRS and chemometric analysis to predict fruit defects and postharvest behaviour of ‘Nules Clementine’ mandarin fruit. Food Chem. 163:267–274. doi:10.1016/j.foodchem.2014.04.085.
- Magwaza, L.S., U.L. Opara, L.A. Terry, S. Landahl, P.J.R. Cronje, H.H. Nieuwoudt, A. Hanssens, W. Saeys, and B.M. Nicolaï. 2013b. Evaluation of Fourier transform-NIR spectroscopy for integrated external and internal quality assessment of Valencia oranges. J. Food Compos. Anal. 31(1):144–154. doi: 10.1016/j.jfca.2013.05.007.
- Magwaza, L.S., U.L. Opara, P.J.R. Cronje, S. Landahl, H.H. Nieuwoudt, A.M. Mouazen, B.M. Nicolaï, and L.A. Terry. 2014b. Assessment of rind quality of ‘Nules Clementine’ mandarin during postharvest storage: 1. Vis/NIRS PCA models and relationship with canopy position. Scientia Horticult. 165:410–420. doi:10.1016/j.scienta.2013.09.035.
- Magwaza, L.S., U.L. Opara, P.J.R. Cronje, S. Landahl, H.H. Nieuwoudt, A.M. Mouazen, B.M. Nicolaï, and L.A. Terry. 2014c. Assessment of rind quality of ‘Nules Clementine’ mandarin fruit during postharvest storage: 2. Robust Vis/NIRS PLS models for prediction of physico-chemical attributes. Scientia Horticulturae 165:421–432. doi:10.1016/j.scienta.2013.09.050.
- Magwaza, L.S., U.L. Opara, P.J.R. Cronje, S. Landahl, and L.A. Terry. 2013a. Canopy position affects rind biochemical profile of ‘Nules Clementine’ mandarin fruit during postharvest storage. Postharvest Biol Technol. 86:300–308. doi:10.1016/j.postharvbio.2013.07.029.
- McDonald, R.E., W.R. Miller, and T.G. McCollum. 2000. Canopy position and heat treatments influence gamma-irradiation-induced changes in phenylpropanoid metabolism in grapefruit. J. Am. Soc. Hortic. Sci. 32(3):364–369. doi: 10.21273/JASHS.125.3.364.
- Olarewaju, O. O., Magwaza, L. S., Fawole, O. A., Tesfay, S. Z., & Opara, U. L. (2017). Role of canopy positions on rind biochemical concentrations and radical-scavenging activities in relation to rind breakdown of ‘Nules Clementine’ mandarins stored at non-chilling temperature. Scientia Horticulturae, 226, 231–240. doi: 10.1016/j.scienta.2017.08.048.
- Pathare, P.B., U.L. Opara, and F.A.-J. Al-Said. 2013. Colour measurement and analysis in fresh and processed foods: A review. Food Biopro. Technol. 6(1):36–60. doi: 10.1007/s11947-012-0867-9.
- Santos, P.H., and M. Silva. 2008. Retention of vitamin C in drying processes of fruits and vegetables — A review. Drying Technol. 26(12):1421–1437. doi: 10.1080/07373930802458911.
- Sdiri, S., P. Navarro, A. Monterde, J. Benabda, and A. Salvador. 2012. Effect of postharvest degreening followed by a cold-quarantine treatment on vitamin C, phenolic compounds and antioxidant activity of early-season citrus fruit. Postharvest Biol. Technol. 65:13–21. doi:10.1016/j.postharvbio.2011.10.010.
- Vacante, V. 2009. Citrus mites: Identification, bionomy and control. CABI, Wallinford, Oxfordshire, UK. doi:10.1079/9781845934989.0000.
- Wei, Y., F. Xu, and X. Shao. 2017. Changes in soluble sugar metabolism in loquat fruit during different cold storage. J. Food Sci. Technol. 54(5):1043–1051. doi: 10.1007/s13197-017-2536-5.
- Yu, L., H. Liu, X. Shao, F. Yu, Y. Wei, Z. Ni, F. Xu, and H. Wang. 2016. Effects of hot air and methyl jasmonate treatment on the metabolism of soluble sugars in peach fruit during cold storage. Postharvest Biol. Technol. 113:8–16. doi:10.1016/j.postharvbio.2015.10.013.