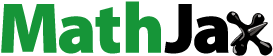
ABSTRACT
Changes in physical and chemical profiles during spontaneous fermentation of olives from the Moroccan Picholine cultivar at two degrees of ripeness (green and purple stages) were investigated in both olive flesh and brine. Purple olives exhibited the highest sugar, total phenolic and flavonoids content. Spontaneous fermentation led to an important loss of total phenolic, flavonoids and sugar content, particularly in green olives with reductions rate of 43%, 65% and 73%, respectively. The loss of phenolic compounds through fermentation was accompanied by a decrease of the antioxidant activity in olive flesh. The chromatographic analysis showed qualitative and quantitative differences among the olive flesh and brine studied. By the end of fermentation, an increase in hydroxytyrosol and caffeic acid concentrations and a decrease of protocatechuic acid, ferulic acid, p-coumaric acid and quercetine amounts were observed in both olive fleshes. The main phenolic compound identified and quantified in both tested brines was hydroxytyrosol. The purple-olive flesh and green olives brine were found to have the highest antioxidant activity after 66 days of natural fermentation.
Introduction
Accounting for almost 98% of the world’s olive tree plantation, the Mediterranean region is the major international olive-growing area. Moroccan olive plantations cover 1 million ha and produce approximately 1.4 million tons of raw olives (MAPM (Citation2016), 2015–2016 season). O. europaea L. cv. Moroccan Picholine is the most abundant olive variety, which represents more than 96% of the total olive trees in Morocco. It is a dual-purpose variety used for table olives and olive oil production. It has an average oil content of 20–24% with a high content of oleic acid, phenolic compounds, tocopherols and a good resistance to oxidation (Mahhou et al., Citation2012).
Table olives are considered as the most popular fermented crop worldwide. World production reached 2.87 million tons for the 2017–2018 season, and about 4% of this amount was produced in Morocco (IOC, Citation2018). There are three main trade preparations of table olives: the Spanish (or Sevillian)-style green olives in brine, Californian-style black olives in brine, and natural black or Greek-style olives in brine. The green or Spanish-style olives consist of treating the olives with lye (2–3%) in order to remove the fruit bitterness by hydrolysis of glucoside oleuropein and to increase skin permeability, which, in turn, facilitates the release of nutrients. Then, debittered olives are washed with water (2 or 3 times), to eliminate the excess of alkali. Finally, washed olives are immersed in brine (9–12%), where they undergo a spontaneous fermentation (Arroyo-López et al., Citation2008; Hurtado et al., Citation2012). Purple or black olives are directly stored in brine where they undergo spontaneous fermentation and can be preserved by addition of acidifying agent. These latter preparations do not include the alkali treatment of fruits and thus the final product retains fruity flavor and slightly bitter taste (Lanza, Citation2012).
Lactic acid bacteria (LAB) and yeasts are the main microbial groups involved in table-olive fermentation (Hurtado et al., Citation2012; Lanza, Citation2012), the relative population of which determines the quality and flavor of the final product. Thus, when LAB outnumber yeasts, lactic acid fermentation is favored rendering a more acidic product with a lower pH, which is very desirable in Spanish-style green olive fermentation. The opposite occurs for undebittered table olives, when LAB growth is usually inhibited by the high concentration of polyphenols, in particular, oleuropein and consequently yeasts become the prevailing group, resulting in a final product characterized by a fruity flavor with slightly bitter taste (Lanza, Citation2013).
Sugars are the main soluble components in olive tissues. Glucose, fructose and sucrose are the principal sugars found in the olive flesh. Sugar composition varies from green to black stage due to a series of enzymatic and chemical reactions during the maturation phase such as hydrolysis of polysaccharides by glycosidases and polymerization of free sugar (Conde et al., Citation2008). During the fermentation process, sugars act as carbon source for fermentative microorganisms, which provide organic acids, mainly lactic acid responsible for the stability and preservation of table olives.
Phenolic compounds are well known for their great nutritional and dietetic importance. Actually, phenolic compounds have a redox potential, which enable them to act as hydrogen donators, singlet oxygen quenchers and reducing agents (Arslan et al., Citation2011).
Olive polyphenols composition is variable and depends on many factors such as cultivar (Kiai and Hafidi, Citation2014; Malheiro et al., Citation2011; Tura et al., Citation2007), ripening stage (Bouaziz et al., Citation2004; Menz and Vriesekoop, Citation2010), environmental factors (Ninfali et al., Citation2008), and processing method (Sahan et al., Citation2013). Oleuropein, the bitter secoiridoid, is the major compound in fresh green olives; its concentration decreases during ripeness, while hydroxytyrosol concentration increases (Gutierrez-Rosales et al., Citation2010).
During the fermentation process, several changes in polyphenols quantity and quality occur. In Spanish-style green olive processing, the hydrolysis of glucoside oleuropein into hydroxytyrosol and elenolic acid glucoside occurs resulting in abundant hydroxytyrosol release. The formation of caffeic acid has also been reported, due to verbascoside hydrolysis (Ben Othman et al., Citation2009). However, during natural fermentation of black olive, the main changes that take place are the acid hydrolysis of oleuropein and polymerization of anthocyanins which contribute to the final color of the olives (Romero et al., Citation2004).
Changes in chemical profile during olive processing were studied for several cultivars (cv.) such as Itrana, Peranzana, Cellina di Nardo, Nocellara del Belice and Bella di Cerignola cv. (Tofalo et al., Citation2012), Brandofino, Castriciana, Nocellara del Belice and Passa-lunara cv. (Aponte et al., Citation2010), Chétoui variety (Ben Othman et al., Citation2009), Kalamata and Semidana cv. (Piga et al., Citation2005), Manzanilla, Hojiblanca and Gordal cv. (Montaño et al., Citation2003) and many other olive varieties. However, to the best of our knowledge, there is no published work on the chemical changes in olives from the Moroccan Picholine cultivars at different degrees of ripeness during spontaneous fermentation. Thus, the aim of the present study was to investigate in both olive flesh and brine the main physical and chemical changes occurring during spontaneous fermentation of Moroccan olives from the “Moroccan Picholine” cultivar at two degrees of ripeness (green and purple stages).
Material and Methods
Chemicals and Standards
Ethyl acetate, methanol, acetonitrile and phosphoric acid were of HPLC grade. Vanillic acid, caffeic acid, ferulic acid, gallic acid, tyrosol, p-coumaric acid, catechin, quercetine, 2,2-Diphenyl-1-picrylhydrazyl (DPPH), trichloroacetic acid, potassium ferricyanide, ferric chloride, glucose, Folin-Ciocalteu reagent, sodium carbonate, sodium hydroxide, sodium nitrite, aluminum chloride, sulfuric acid and phenol were purchased from Sigma Aldrich (Germany). All standards used were purchased with minimum purity of 95% and all other reagents were of analytical grade.
Sampling
Olive and brine samples of Moroccan Picholine cultivar were kindly provided locally by a table-olive company. Samples were collected at different durations of fermentation (1, 5, 10, 17, 28, 46 and 66 days after brining), transported to the laboratory and stored in the freezer until their testing for the physicochemical analysis (pH and free acidity) and chemical components (sugar, total phenolic and flavonoid contents).
Extraction
The olive fruits were crushed with hammer crusher in bath of ice (4°C). A quantity (l0 g) of the obtained paste was extracted for 15 min with 20 ml of methanol/water 80:20 (v/v) at room temperature. The mixture was centrifuged at 4000 g for 10 min and the supernatant was recovered. The residues were re-extracted under identical conditions for further two times. The supernatants were combined, filtered and washed with hexane to remove oil. This extract was used for sugars.
The above extract was concentrated to 20 mL and then extracted three times with ethyl acetate (v/v). The three organic fractions were combined, ethyl acetate was evaporated to dryness under vacuum at 45°C, and the residue was dissolved in 5 mL methanol. The extract obtained was used for total phenolic and flavonoid content, antioxidant activity assessment and HPLC analysis.
A volume of 50 mL of brine samples was extracted three times with ethyl acetate (v/v) at room temperature. The extracts were concentrated to dryness in a rotary evaporator and dissolved in 5 mL methanol for antioxidant activity determination and HPLC analysis.
Physicochemical Analysis
The pH of samples was measured using a Multilab P5 (WTW, Germany). Free acidity was determined by titration of 10 ml of brine with NaOH (0.2 N) in the presence of phenolphthalein as color indicator and expressed as percent (w/v) of lactic acid.
Sugar Content
Sugar content was determined according to the method of Rao and Pattabiraman (Citation1989) using glucose as a standard. Sugar content was expressed as g of glucose equivalent (GLE) per liter of brine and as g of GLE per 100 g of flesh dry weight (dw). The sugar content of olive flesh and brines were analyzed in triplicate.
Total Phenolic Content
Total phenolic content was determined colorimetrically using Folin-Ciocalteu reagent according to the method described by Catalano et al. (Citation1999), using tyrosol as a standard. The concentrations were expressed as g of tyrosol equivalent (TYE) per liter of brine and as g of TYE per 100 g of flesh dry weight (dw). The total phenolic content of olive flesh and brines was measured in triplicate.
Flavonoid Content
Flavonoids were quantified by a colorimetric assay developed by (Zhishen et al., Citation1999). The concentrations were expressed as g of catechin equivalent (CAE) per liter of brine and as g of CAE per 100 g of flesh dry weight (dw). The total flavonoid content of olive flesh and brines was analyzed in triplicate.
The Antioxidant Activity
Free Radical-Scavenging Assay
The hydrogen-donating or radical-scavenging ability of olive flesh and brines was determined using the free radical 2,2-diphenyl-1-picrylhydrazyl (DPPH) method according to Bondet et al. (Citation1997). DPPH scavenging capacity was calculated as a percentage of DPPH discoloration using the following equation:
Where Acontrol is the absorbance of DPPH without any sample addition. The sample concentration providing 50% inhibition (IC50) was calculated from the graph plotting inhibition percentage against extract concentrations. Ascorbic acid and α-tocopherol were used as antioxidant standards and positive controls.
Reducing Power Assay
The capacity of olive flesh and brine phenolic extracts to reduce Fe3+ was evaluated according to the method of Oyaizu (Citation1986). EC50 was deduced from the absorbance at 700 nm against extract concentrations. Ascorbic acid and α-tocopherol were used as antioxidant standards and positive controls.
HPLC Analysis
Identification and quantification of phenolic monomers was carried out using a high-performance liquid chromatography (Knauer) equipped with a (K-1001) pump and a PDA detector (200–700 UV-Vis) operating at 280 nm. The column was (4.6 × 250 mm) (Eurospher II 100–5), and the temperature was maintained at 25°C. The flow rate was 1 mL/min and the sample volume injected was 10 μL. A mixture of acidified water (A) and acetonitrile (B) was used as mobile phase for a total running time of 60 min. The identification of phenolic compounds was fulfilled by comparison of retention times and UV-VIS spectra with the standards.
Results and Discussion
pH and Free Acidity Evolution in the Brine during Olive Fermentation
pH values of green olive brine increased during the first 5 days of fermentation before decreasing progressively throughout processing and reached a final value of 4.5 units by the end of fermentation (). This slight increase in pH may be related to residual alkali due to inefficient washings. The initial pH of purple-olive brine was lower than that of green ones. It diminishes progressively during fermentation and reaches a minimal value of 4.4 pH units after 66 days of brining.
Figure 1. Evolution of pH and free acidity (% as lactic acid) of green- and purple-olive brines during spontaneous fermentation. BGO: brine of green olives and BGP: brine of purple olives. When error bars are not visible, determinations were within the range of the symbols on the graph
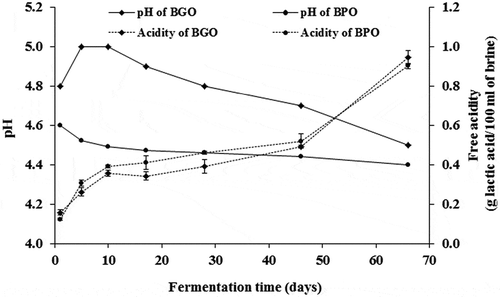
The decrease of pH in both brines during processing is mainly due to the production of lactic acid, which is the main LAB metabolic product as well as the conversion of some phenols on acids such as glucoside elenolic acid released after the hydrolysis of oleuropein. Other organic acids like acetic, malic, citric, formic and succinic also contribute also to the overall pH. Table olives with high pH (>4.5) are considered to be perishable and must undergo a conservative processes that delay the growth of pathogenic microorganisms (Perricone et al., Citation2010).
Excepting a decrease in the acidity rate of green olive brine during the first 5 days, the pattern of the acidity was quite similar for both green and purple olives (). The acidity rate increased rapidly during the first 10 days of brining then a moderate increase followed until the 46th day of brining from which the free acidity rate increased sharply and reached final values of 8.3 and 9 g equivalent lactic acid/L of brine, in green- and purple-olive brines, respectively.
Sugars Contents in Olive Flesh and Brine during Olive Fermentation
Sugars content in both olives followed a similar trend during the brining process (). The initial sugar concentration in purple olives was two folds higher than that in green ones. This may be due to an advanced maturity stage of the purple olives and also to the loss of great amount of sugars during the debittering and washing steps preceding the fermentation of green olives. It is noticeable that the depletion of sugars is very pronounced during the first two weeks of processing with a reduction rate of about 14% and 20% for green and purple olives, respectively. Beyond the 28th day of brining, a relative stabilization of sugars content in the purple and green olives was noticed. Sugars diffusion from olive fruit to the brine is reported to depend on many factors such as skin permeability, fruit to brine ratio, salt concentration and temperature (Rodríguez-Gómez et al., Citation2012). A maximum concentration of sugars in the green olive brines (6.7 g GLE/L) is attained only after 5 days of fermentation, followed by a very fast decrease. After the 30th day of fermentation, the decrease of sugars content become slow and reach a final concentration of 1.1 g GLE/L. The diffusion of sugars from purple olives increased progressively throughout the processing period and reached almost similar maximum concentration (6.66 g GLE/L) but at the 45th day of fermentation and started to decrease thereafter. By the end of fermentation, the sugars loss in olive flesh is estimated to 60% and 73% in purple and green olives flesh, respectively. Purple olives showed fairly high residual sugar levels in the brine by the end of fermentation (close to 6.2 g GLE/L) (). However, the final residual sugar concentrations in green olives were very low (below 1.1 g GLE/L) (). Our results are in line with those reviewed by López-López et al. (Citation2007); showing that the untreated olives exhibit higher residual sugars than do treated green olives. In fact, the numerous lye and washing treatments used in Spanish-style green olives permitted a quick exchange between the brine and the flesh because of the high permeability of its membrane to water-soluble compounds.
Total Phenolic Content Evolution in Olive Flesh and Brine during Olive Fermentation
As shown in , the initial concentration of total phenolic content in olive flesh of green olives was 2.11 g TYE/100 g dw, which is threefold lower than reported in unprocessed olives (5.43 g TYE/100 g dw). The Spanish-style green olive processing implies a series of steps (debittering and washing) prior to fermentation and during which phenolic compounds are leached from fruits. Furthermore, several factors (the cultivar, degree of ripeness, biotic and abiotic stresses, processing method, etc.) are known to affect the quantitative phenolic profiles of olive fruits. It is noteworthy that the Moroccan Picholine cultivar is classified as a very high phenolic content variety (El Qarnifa et al., Citation2019).
Figure 3. Evolution of olive flesh and brine total phenolic content during spontaneous fermentation. GO: green olives; PO: purple olives; BGO: brine of green olives and BPO: brine of purple olives. When error bars are not visible, determinations were within the range of the symbols on the graph
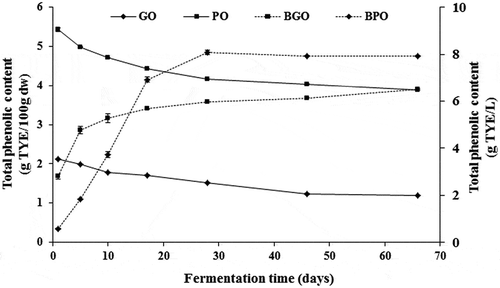
Our results showed a progressive decrease of phenolic contents in both olive types. Total phenolic content decreased from 2.11 to 1.2 g TYE/100 g dw (- 43%) and reduced from 5.43 to 4.0 g TYE/100 g of dw (- 28%) after 66 days of processing of green and purple olives, respectively. Processing styles affected phenolic contents. The phenolic concentration in green olives was fairly low (due to the quick exchange during fermentation). As there is no previous lye treatment, the epidermis of the purple fruits remains intact, and acts as a barrier, so that diffusion of polyphenols is slow. As a consequence, the level of phenolic compounds in the pulp after fermentation is higher in untreated olives than in Spanish-style green olives. This is in agreement with previous studies (Blekas et al., Citation2002; Romero et al., Citation2004; Sahan et al., Citation2013).
The residual phenolic content in purple-olive flesh of the Moroccan Picholine cultivar is four fold higher than that of the Chétoui cultivar (4 vs. 1 g/100 g dw) (Ben Othman et al., Citation2009). However, the residual polyphenols in green olives of the Moroccan Picholine cultivar are 20 times lower than that of the Gemlik cultivar (0.35 vs. 7 g/100 g fw) (Sahan et al., Citation2013). As a result of the diffusion exchange between olive and brine, total phenolic content in both brines increased rapidly during the first 30 days of fermentation (), followed by a moderate rise in green olives brine and a steady state in purple olives brine. Final values reached 6.5 and 7.9 g TYE/L for green- and purple-olive brines, respectively.
Flavonoids Content Evolution in Olive Flesh and Brine during Olive Fermentation
At the beginning of fermentation, the flavonoids content amounted for about 1.17–2.47 g CAE/100 g dw in green and purple olives, respectively (). In general, about 21% and 44% of flavonoids content disappeared after only 17 days of brining in purple and green olives, respectively. Flavonoids are water-soluble compounds, so they diffused easily into the brine that assumed a color similar to olives, especially in purple olives. It is noticeable that the depletion followed a quick kinetic in the first 17 days and a slower one thereafter. By the end of fermentation, flavonoids loss is estimated to about 25% and 65% in purple and green olives flesh, respectively. In the opposite side, flavonoids diffusion into the brine increased progressively throughout the fermentation process and reached final concentrations of about 3.31 and 4.1 g CAE/L in green- and purple-olive brines, respectively.
Identification and Quantification of Simple Phenolic Compounds in the Olive Flesh and Brine
The studied samples showed different profiles. Eleven simple phenolic compounds were identified and quantified ( and ). The main components identified in olive flesh were hydroxytyrosol and quercetine. Tyrosol, protocatechuic acid, p-hydroxybenzoic acid, vanillic acid, caffeic acid, p-coumaric acid, ferulic acid, and catechins were also identified. The differences in olive flesh phenolic contents may be attributed to differences in maturation (Bouaziz et al., Citation2004; Menz and Vriesekoop, Citation2010) and processing methods (Marsilio et al., Citation2005).
Table 1. Simple phenolic compounds in olive flesh at the 2nd and the 66th days of spontaneous fermentation of Moroccan Picholine cultivar at green and purple stages, expressed in mg TYE/100 g dry weight
Table 2. Simple phenolic compounds in brine at the 2nd and the 66th day of spontaneous fermentation of Moroccan Picholine cultivar at green and purple stages, expressed in mg TYE/L
Total simple phenolic content in olive flesh decreased after fermentation from 1.19 to 0.99 g/100 g dw in green olives, and from 3.34 to 2.49 g/100 g dw in purple olives. These contents are lower than those obtained by the Folin-Ciocalteu determination which is known to overestimate the phenolic content (El-Abbassi et al., Citation2012). The main changes for both types of olives were the decrease in concentrations of almost all the identified phenolic compounds while the hydroxytyrosol showed a marked increase in both olives flesh after fermentation due to its progressive production through the acid and enzymatic hydrolysis of hydroxytyrosol glucoside and oleuropein. This is in agreement with the results reported by Ben Othman et al. (Citation2009).
Caffeic acid was absent in olive flesh at the beginning and appeared by the end of fermentation in both olives flesh. Brenes-Balbuena et al. (Citation1992) reported that caffeic acid was obtained from verbascoside hydrolysis. The evolution of other phenols depends on the ripeness stage. Gallic and vanillic acids were identified in green olives and their concentration decreased after fermentation. However, they were not detected in purple olives. Catechin was found only in purple olives and its concentration decreased after the brining process.
Only seven of these simple phenolic compounds were identified in brines. In green olives brine relatively high simple phenolic content was noticed, probably due to the degradation of complex phenolic compounds. Actually, Lactobacillus plantarum was reported to depolymerize high molecular weight phenolic compounds to lower molecular weight (Ayed and Hamdi, Citation2003). Hydroxytyrosol was the principal phenol identified in both brines, its percentage varied between 64% and 82% of total simple phenolic compounds in green and purple olives brine, respectively. Parinos et al. (Citation2007) reported that wastewaters resulting from green olive processing (lye, washing wastewaters and brines) contain high hydroxytyrosol concentrations, since this compound constitutes the main hydrolysis product of oleuropein. Romero et al. (Citation2002) propose that it results from acid hydrolysis of hydroxytyrosol-4-b-glucoside. This compound has been shown to be the principal phenol in mature olives (Romero et al., Citation2002). An important increase in tyrosol content was noticed in both brines after fermentation, due to the hydrolysis of tyrosol glucoside as well as the diffusion of tyrosol from olive flesh to the brine. Other phenolic compounds identified in the brine were p-coumaric acid, p-hydroxybenzoic acid and caffeic acid. The appearance of these simple phenolics during table-olive fermentation was also observed by several authors (Ben Othman et al., Citation2009; Blekas et al., Citation2002; Rodríguez et al., Citation2008).
Evaluation of the Antioxidant Activities
shows the DPPH radical-scavenging activity as well as the reducing power capacity of phenolic extracts of olive flesh and brines. The lowest IC50 and EC50 values indicate the highest antioxidant activities. All tested samples showed an antioxidant capacity. At the beginning of the brining process, the IC50 values were between 233 and 307 μg/mL, and the EC50 values varied between 160 and 182 μg/mL for purple and green olives, respectively. By the end of the fermentation, the IC50 values increased to 290 and 351 μg/mL while the EC50 increased to 178 and 209 μg/mL for purple and green olives, respectively. It is well known that the modification of the processing conditions and type of fermentation changed the profile and levels of phenolic compounds, with consequent impact on the antioxidant capacity of the finished product. IC50 and EC50 values were lower in the purple olives flesh, which is in agreement with the results of the total phenolic analysis. This can be attributed to the significant radical inhibition and reducing power ability caused by a high concentration of o-diphenol such as hydroxytyrosol and quercetine. These compounds react with free radicals and produce a new radical, which is stabilized by the resonance effect of the aromatic ring (Bouaziz et al., Citation2004). The present results are in accordance with those reported by Belaqziz et al. (Citation2017) who investigated the antioxidant capacity of brines of table olives with different stages of maturity (black, purple and green) and stated that purple-olive brine with its higher concentration of phenolic compounds exhibited a higher antioxidant potential compared to green- and black-olive brines. The antioxidant capacity using both tests increased after fermentation for green and purple olives brines. However, the IC50 and EC50 values were found to be lower in the green-olive brines with respect to the purple ones.
Table 3. IC50 (DPPH assay) and EC50 (RP assay) in olive flesh and brine at the 2nd and the 66th days of spontaneous fermentation of Moroccan Picholine cultivar at green and purple stages. Ascorbic acid and α-tocopherol are positive controls
Conclusion
For the first time, a study on fermentation chemistry of olives from the Moroccan Picholine cultivar at two degrees of ripeness has been performed. The pH, free acidity, sugars, total phenolic and flavonoid contents as well as the antioxidant capacity of olives flesh and brines throughout fermentation period were carried out. After fermentation, the purple-olive brine showed a lower pH and thus the higher free acidity. The obtained results showed differences in total phenolic, simple phenolic composition and antioxidant capacity. These differences can be explained by the variation of maturation stage as well as the process used. Eleven simple phenolic compounds were identified in olive flesh. The main components were hydroxytyrosol, tyrosol and quercetine. Olive processing induced an important phenolic loss (28–43%) in olives flesh, leading to a reduction in antioxidant values. The green olive brine of the Moroccan Picholine cultivar was found to have the highest simple phenolics content and antioxidant activity.
It is worthy to note that olive brine can be considered as natural source of high-added value products; in particular lactic acid and phenolic compounds (hydroxytyrosol and ortho-diphenols) with high antioxidant activity and nutritional properties (Kiai et al., Citation2018). These compounds are usually synthesized by chemical methods, which are responsible for their high price. Whence, if phenols and lactic acid could be collected from table-olive processing wastewaters, this may lead to economic benefits.
Acknowledgments
The authors wish to thank the FRAMACO Company for their help and kindness during all the sampling period. This work has benefited from support within the SATRPES Project “Valorization of Bio-resources based on Scientific Evidence in Semi- and Arid Land for Creation of New Industry”. The authors would like to thank the JICA and JST (Japan) for their financial support.
References
- Aponte, M., V. Ventorino, G. Blaiotta, G. Volpe, V. Farina, G. Avellone, C.M. Lanza, and G. Moschetti. 2010. Study of green Sicilian table olive fermentations through microbiological, chemical and sensory analyses. Food Microbiol. 27:162–170. doi: 10.1016/j.fm.2009.09.010.
- Arroyo-López, F.N., A. Querol, J. Bautista-Gallego, and A. Garrido-Fernández. 2008. Role of yeasts in table olive production. Int. J. Food Microbiol. 128:189–196. doi: 10.1016/j.ijfoodmicro.2008.08.018.
- Arslan, D., M.M. Özcan, and M.M. Özcan. 2011. Phenolic profile and antioxidant activity of olive fruits of the Turkish variety “Sarıulak” from different locations. Grasas Y Aceites. 62:453–461. doi: 10.3989/gya.034311.
- Ayed, L., and M. Hamdi. 2003. Fermentative decolorization of olive mill wastewater by Lactobacillus plantarum. Process Biochem. 39:59–65. doi: 10.1016/S0032-9592(02)00314-X.
- Belaqziz, M., S.P. Tan, A. El-Abbassi, H. Kiai, A. Hafidi, O. O’Donovan, and P. McLoughlin. 2017. Assessment of the antioxidant and antibacterial activities of different olive processing wastewaters. PLoS ONE. 12:e0182622. doi: 10.1371/journal.pone.0182622.
- Ben Othman, N., D. Roblain, N. Chammen, P. Thonart, and M. Hamdi. 2009. Antioxidant phenolic compounds loss during the fermentation of Chétoui olives. Food Chem. 116:662–669. doi: 10.1016/J.FOODCHEM.2009.02.084.
- Blekas, G., C. Vassilakis, C. Harizanis, M. Tsimidou, and D.G. Boskou. 2002. Biophenols in table olives. J. Agric. Food Chem. 50:3688–3692. doi: 10.1021/jf0115138.
- Bondet, V., W. Brand-Williams, and C. Berset. 1997. Kinetics and mechanisms of antioxidant activity using the DPPH. Free radical method. LWT Food Sci. Technol. 30:609–615. doi: 10.1006/FSTL.1997.0240.
- Bouaziz, M., M. Chamkha, and S. Sayadi. 2004. Comparative study on phenolic content and antioxidant activity during maturation of the olive cultivar chemlali from Tunisia. J. Agric. Food Chem. 52:5476–5481. doi: 10.1021/jf0497004.
- Brenes-Balbuena, M., P. Garcia-Garcia, and A. Garrido-Fernandez. 1992. Phenolic compounds related to the black color formed during the processing of ripe olives. J. Agric. Food Chem. 40:1192–1196. doi: 10.1021/jf00019a023.
- Catalano, L., I. Franco, and M. Nobili. de (Udine U. (Italy). D. di P.V. e T.A., Leita, L. (Istituto S. per la N. delle P.R. (Italy)). 1999. Polyphenols in olive mill waste waters and their depuration plant effluents. A comparison of the Folin-Ciocalteau and HPLC methods. Agrochim, Italy.
- Conde, C., S. Delrot, and H. Gerós. 2008. Physiological, biochemical and molecular changes occurring during olive development and ripening. J. Plant Physiol. 165:1545–1562. doi: 10.1016/J.JPLPH.2008.04.018.
- El Qarnifa, S., A. El Antari, and A. Hafidi. 2019. Effect of maturity and environmental conditions on chemical composition of olive oils of introduced cultivars in Morocco. J. Food Qual. 2019:1–14. doi: 10.1155/2019/1854539.
- El-Abbassi, A., H. Kiai, and A. Hafidi. 2012. Phenolic profile and antioxidant activities of olive mill wastewater. Food Chem. 132:406–412. doi: 10.1016/j.foodchem.2011.11.013.
- Gutierrez-Rosales, F., M.P. Romero, M. Casanovas, M.J. Motilva, and M.I. Mínguez-Mosquera. 2010. Metabolites involved in oleuropein accumulation and degradation in fruits of olea europaea L.: Hojiblanca and arbequina varieties. J. Agric. Food Chem. 58:12924–12933. doi: 10.1021/jf103083u.
- Hurtado, A., C. Reguant, A. Bordons, and N. Rozès. 2012. Lactic acid bacteria from fermented table olives. Food Microbiol. 31:1–8. doi: 10.1016/J.FM.2012.01.006.
- IOC, 2018. World table olive figures - International olive council [WWW document]. 19 Mar. 2018. http://www.internationaloliveoil.org/estaticos/view/132-world-table-olive-figures/
- Kiai, H., and A. Hafidi. 2014. Chemical composition changes in four green olive cultivars during spontaneous fermentation. LWT - Food Sci. Technol. 57:663–670. doi: 10.1016/j.lwt.2014.02.011.
- Kiai, H., J. Raiti, A. El-Abbassi, and A. Hafidi. 2018. Recovery of phenolic compounds from table olive processing wastewaters using cloud point extraction method. J. Environ. Chem. Eng. 6:1569–1575. doi: 10.1016/j.jece.2018.05.007.
- Lanza, B. 2012. Nutritional and sensory quality of table olives, in: Olive germplasm - The olive cultivation, table olive and olive oil industry in Italy. InTech. doi: 10.5772/51723.
- Lanza, B. 2013. Abnormal fermentations in table-olive processing: Microbial origin and sensory evaluation. Front. Microbiol. 4. doi: 10.3389/fmicb.2013.00091.
- López-López, A., A. Jiménez-Araujo, P. García-García, and A. Garrido-Fernández. 2007. Multivariate analysis for the evaluation of fiber, sugars, and organic acids in commercial presentations of table olives. J. Agric. Food Chem. 55:10803–10811. doi: 10.1021/jf0720638.
- Mahhou, A., Y. Nabil, A. Hadiddou, A. Oukabli, and A. Mamouni. 2012. No TitlePerformance of the Arbequina, Haouzia and Menara olive varieties in rainfed conditions in the Meknès region of Morocco. OLIVAE Off. Mag. Int. Olive Counc. 118:3–21.
- Malheiro, R., A. Sousa, S. Casal, A. Bento, and J.A. Pereira. 2011. Cultivar effect on the phenolic composition and antioxidant potential of stoned table olives. Food Chem. Toxicol. 49:450–457. doi: 10.1016/j.fct.2010.11.023.
- MAPM, 2016.Rapports Statistiques - Compagne Agricole 2015-2016 [WWW Document]. Minist. Agric. Sea Fishing, Rural Dev. Waters For. 2 Aug. 2018. http://www.agriculture.gov.ma/pages/rapports-statistiques/campagne-agricole-2015-2016
- Marsilio, V., L. Seghetti, E. Iannucci, F. Russi, B. Lanza, and M. Felicioni. 2005. Use of a lactic acid bacteria starter culture during green olive (Olea europaea L cv Ascolana tenera) processing. J. Sci. Food Agric. 85:1084–1090. doi: 10.1002/jsfa.2066.
- Menz, G., and F. Vriesekoop. 2010. Physical and chemical changes during the maturation of gordal sevillana olives (Olea europaea L., cv. Gordal Sevillana). J. Agric. Food Chem. 58:4934–4938. doi: 10.1021/jf904311r.
- Montaño, A., A.H. Sánchez, F.J. Casado, A. de Castro, and L. Rejano. 2003. Chemical profile of industrially fermented green olives of different varieties. Food Chem. 82:297–302. doi: 10.1016/S0308-8146(02)00593-9.
- Ninfali, P., M. Bacchiocca, E. Biagiotti, S. Esposto, M. Servili, A. Rosati, and G. Montedoro. 2008. A 3-year study on quality, nutritional and organoleptic evaluation of organic and conventional extra-virgin olive oils. J. Am. Oil Chem. Soc. 85:151–158. doi: 10.1007/s11746-007-1171-0.
- Oyaizu, M. 1986. Studies on products of browning reaction–antioxidative activities of products of browning reaction prepared from glucosamine. Jpn. J. Nutr. Diabetics. 44:307–315. doi: 10.5264/eiyogakuzashi.44.307.
- Parinos, C.S., C.D. Stalikas, T.S. Giannopoulos, and G.A. Pilidis. 2007. Chemical and physicochemical profile of wastewaters produced from the different stages of Spanish-style green olives processing. J. Hazard. Mater. 145:339–343. doi: 10.1016/j.jhazmat.2006.12.061.
- Perricone, M., A. Bevilacqua, M.R. Corbo, and M. Sinigaglia. 2010. Use of lactobacillus plantarum and Glucose to control the fermentation of “Bella di Cerignola” table olives, a traditional variety of Apulian Region (Southern Italy). J. Food Sci. 75:M430–M436. doi: 10.1111/j.1750-3841.2010.01742.x.
- Piga, A., A. Del Caro, I. Pinna, and M. Agabbio. 2005. Anthocyanin and colour evolution in naturally black table olives during anaerobic processing. LWT - Food Sci. Technol. 38:425–429. doi: 10.1016/J.LWT.2004.06.011.
- Rao, P., and T.N. Pattabiraman. 1989. Reevaluation of the phenol-sulfuric acid reaction for the estimation of hexoses and pentoses. Anal. Biochem. 181:18–22. doi: 10.1016/0003-2697(89)90387-4.
- Rodríguez, H., J.M. Landete, B. Rivas, and R. de Las Muñoz. 2008. Metabolism of food phenolic acids by Lactobacillus plantarum CECT 748T. Food Chem. 107:1393–1398. doi: 10.1016/J.FOODCHEM.2007.09.067.
- Rodríguez-Gómez, F., J. Bautista-Gallego, V. Romero-Gil, F.N. Arroyo-López, A. Garrido-Fernández, and P. García-García. 2012. Effects of salt mixtures on Spanish green table olive fermentation performance. LWT - Food Sci. Technol. 46:56–63. doi: 10.1016/j.lwt.2011.11.002.
- Romero, C., M. Brenes, P. García, A. García, and A. Garrido. 2004. Polyphenol changes during fermentation of naturally black olives. J. Agric. Food Chem. 52:1973–1979. doi: 10.1021/jf030726p.
- Romero, C., M. Brenes, P. García, and A. Garrido. 2002. Hydroxytyrosol 4-beta-D-glucoside, an important phenolic compound in olive fruits and derived products. J. Agric. Food Chem. 50:3835–3839. doi: 10.1021/jf011485t.
- Sahan, Y., A. Cansev, and H. Gulen. 2013. Effect of processing techniques on antioxidative enzyme activities, antioxidant capacity, phenolic compounds, and fatty acids of table olives. Food Sci. Biotechnol. 22:613–620. doi: 10.1007/s10068-013-0122-9.
- Tofalo, R., M. Schirone, G. Perpetuini, G. Angelozzi, G. Suzzi, and A. Corsetti. 2012. Microbiological and chemical profiles of naturally fermented table olives and brines from different Italian cultivars. Antonie Van Leeuwenhoek. 102:121–131. doi: 10.1007/s10482-012-9719-x.
- Tura, D., C. Gigliotti, S. Pedò, O. Failla, D. Bassi, and A. Serraiocco. 2007. Influence of cultivar and site of cultivation on levels of lipophilic and hydrophilic antioxidants in virgin olive oils (Olea Europea L.) and correlations with oxidative stability. Sci. Hortic. (Amsterdam). 112:108–119. doi: 10.1016/J.SCIENTA.2006.12.036.
- Zhishen, J., T. Mengcheng, and W. Jianming. 1999. The determination of flavonoid contents in mulberry and their scavenging effects on superoxide radicals. Food Chem. 64:555–559. doi: 10.1016/S0308-8146(98)00102-2.