ABSTRACT
In the autumn of 2010, in a replanted orchard in the research garden of the University of Natural Resources and Life Sciences in Vienna, the apple cultivar ‘Topaz’ was planted on the rootstocks M.9 (clone T337) with inter-stem ‘Summerred’, G.11, CG.13, G.16, G.41, G.202, M.7 and MM.111. The trees have been growing according to organic production standards, and were observed for nine years. G.11 and G.41 showed good results, even if tree losses due to crown rot (Phytphthora cactorum) in combination with the highly susceptible cv. Topaz were high, compared to the standard M.9 with inter-stem. G.11 was similar to M.9 in growth but had higher fruit yield. G.41 grows 10% more vigorously with good yield results, and is therefore ideal for replanting orchards. The other tested rootstocks from Geneva did not prove to be suitable.
Introduction
‘Malling 9ʹ (M.9) is the most widely used apple rootstock worldwide, and also the most widely used rootstock for organic growers in Europe, although more tolerant and even vigorously growing alternatives, especially for replanted orchards and extensive production systems, have been discussed (Hornig and Paul, Citation2011; Ruess, Citation2010). M.9 is (1) well adapted to different soil types, (2) induces a dwarfing growth with an early and high yield, and (3) possesses excellent fruit quality. Despite these many advantages, M.9 and its clones all suffer from a number of drawbacks such as poor anchorage in the soil and susceptibility to the apple replant disease (ARD), fire blight (Erwinia amylovora) as well as the woolly apple aphid (Eriosoma lanigerum) (Auvil et al., Citation2011; Fazio et al., Citation2015; Marini et al., Citation2014). In particular, the ARD is a major problem in replanted apple orchards. Reductions in growth and yield are symptomatic and mainly caused by nematodes (Pratylenchus) and soil borne funghi (e.g. Rhizoctonia, Phytophthora, Pythium) (Mazzola, Citation1998). For organic growers, besides bio-fumigation (Yim et al., Citation2016), the use of tolerant rootstocks has been suggested as an efficient way to prevent ARD (Gasser et al., Citation2009; Hewavitharana et al., Citation2019; Robinson and Fazio, Citation2019; Thalheimer, Citation2016). Some of the rootstocks from Cornell Geneva (USA), which were bred for resistance to fire blight (Erwinia amylovora) and Phytophthora-crown rot resistance, are also highly tolerant to ARD (Aldwinckle et al., Citation2004; Auvil et al., Citation2011; Hewavitharana et al., Citation2019; Robinson and Fazio, Citation2019). There have been some publications about the performance of Geneva rootstocks in Mid Europe (Höller and Guerra, Citation2017; Kockerols et al., Citation2009) but only very few (Pfeiffer, Citation2016; Wurm, Citation2018) from organic production systems so far.
The purpose of this study is the evaluation of some important rootstocks from Geneva in an organically treated replanted apple orchard, drawing comparisons with the standard M.9 and with the stronger rootstocks M.7 and MM.111.
Materials and Methods
We established the trial in the research orchard of our University in the northeast of Vienna. The average yearly temperature at the site is around 10°C, the yearly rainfall about 550 mm. The soil is a chernozem with a pH value of 7.6 and a high share of lime (15%).
‘Topaz’, currently one of the mainly used cultivars in organic production in Austria and Germany, was chosen for the trial and grafted on the following rootstocks: M.9 (clone T337) with inter-stem ‘Summerred’ between 20 and 65 cm, Geneva 11 (G.11), Cornell Geneva 13 (CG.13), Geneva 16 (G.16), Geneva 41 (G.41), Geneva 202 (G.202), M.7 and MM.111. In the autumn of 2010, the two-year old trees (5 repetitions with 4 trees, in total 20 trees per variant) were planted in a previously cleared apple orchard in the exact same rows as the trees had previously stood on M.9. The planting distance between the rows was 3.5 m, and varied between 1.1 m (M.9, G.11, CG.13 and G.16), 1.3 m (G.41), 1.45 m (G.202), 1.6 m (M.7) and 1.8 m (MM.111) in the row.
The orchard management was performed according to organic production standards. After the second year, an organic fertilizer (Biofert, Wilfinger, Austria or Bioagenasol, Agrana, Austria) was distributed in the tree strip (30 kg N/ha, y) every March. The tree strip was mechanically kept free, and the alleyways were mulched three to four times per year. All plant treatments were in line with organic production rules, using Neem and, in some years, Quassia in springtime, Granulosevirus during summer, and mainly sulfur and small amounts of copper for fungal disease control. During the vegetation period, the trees were irrigated by over crown irrigation, when required.
Weight and number of fruits were counted for each tree at harvest from 2012 to 2019. The trunk circumference was measured with a tape at 50 cm height from soil line in winter from 2011 to 2019. We calculated the trunk cross-sectional area (TCSA) every year as well as the cumulative yield efficiency (CYE) as the ratio between the cumulative yield in kilograms per tree (from 2012 to 2019) per final TCSA. In 2018 and 2019, the presence and amount (rating scale from 1 = few to 5 = many) of root suckers were observed. As many trees did not come into leaf or were showing wilting symptoms due to Phytophthora cactorum infestation in the zone of the grafting point of the trunk in spring 2020, we conducted a final assessment of tree survival and stem damage in May 2020. The infestation with rosy apple aphid (Dysaphis plantaginea) was observed annually in late June or the or early July, using a rating scale from 1 (no infestation) to 9 (extremely high infestation). In 2017, 2018 and 2019 all harvested fruits were assessed for commercial fruit quality.
In the years of 2015 and 2017, 15 fruits per variant and repetition were stored at 4°C in a cool chamber after harvest. Once in November and once in January, five fruits per repetition (i.e. 25 per variant in total) were analyzed in the laboratory. We measured the index of fruit shape (calculated with the formula: fruit length2/fruit width* fruit thickness), flesh firmness (penetrometer force-analyzer AFG 500 N, Mecmesin, Australia) and the soluble dry matter by a digital refractometer (Atago, Japan) in °Brix at 20°C (Khazaei et al., Citation2008). Furthermore, the titratable acid was measured with the instrument TitroLinealpha plus (Schott, Germany) in line with OECD standards (Citation2005), by titrating 5 ml of sample volume to a pH value of 8,1 with 0.1 mol/L NaOH (Thybo et al., Citation2006). We calculated the sugar/acid ratio with the following formula: °Brix * 10/[g/L] malic acid (OECD, Citation2005). In the years of 2017 and 2019, one box of 20 kg per plot was put into a cold storage house after harvest. After storage at the end of January, we measured weight loss, counted the percentage of rotten fruits, and assessed the covering color in the following categories: < 50%, 50–75%, 75–90%, >90%.
The statistical analysis was done with SPSS (Version 24, IBM, Austria). After a test of homogeneity of variance and normal distribution, we used ANOVA to assess differences between the means with the S-N-K-test on a significance level of p < .05.
Results and Discussion
Tree Losses
By the end of 2019, some trees on G.202 (20%), G.41 (15%), G.11 (10%) and MM.111 and G.16 (each 5%) were dead, mainly due to crown rot (Phytophthora cactorum), to which Topaz is very sensitive. On CG.13, M.7 and M.9 with inter-stem no tree losses have been registered so far (data not shown). In the following spring of 2020, we observed a massive increase in dead trees and trees with symptoms of crown rot on almost all variants (). We found the most severe losses on G.11 (35% dead + 25% damaged), G.202 (25% dead + 30% damaged), G.41 and CG.13 (each 15% dead + 40% damaged). Notably, also MM.111 (5% dead + 35% damaged), G.16 (5% dead + 25% damaged) and M.7 (25% damaged) showed Phytophthora-infestations, though to a lesser extent. Only on M.9 with inter-stem 100% of trees were still healthy.
Figure 1. Percentage of trees lost to and damaged by crown rot (Phytophthora cactorum) of 8 rootstocks grafted with ‘Topaz’ apple cultivar after 9 years (May 2020)
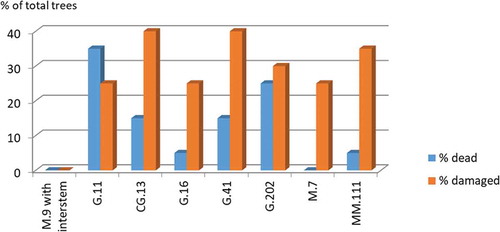
Recent trials in the US with other scions showed different results. Reig et al. (Citation2018) reported no tree losses with Fuji on G.202 in replanted soil. Similarly, Robinson and Fazio (Citation2019) mentioned G.11, G.41 and G.202 as resistant to Phytophthora rot. The reason for the contrary results in our study is most probably the scion used in the experiment. ‘Topaz’ is known to be highly susceptible to crown rot (Phytophthora cactorum), as it has air roots on the stem, which can easily be infected by zoospores of P. cactorum from the soil (Lindner, Citation2009). In combination with this cultivar on M.9 a grafting height, between 40–70 cm over the soil line or the use of a robust cultivar (e.g. Summerred, Rubinola) as inter-grafting are therefore recommended (Lindner, Citation2009). We can see this in a previous trial with ‘Topaz’ grafted on M.9 with and without inter-grafting, M.26, M.7 and MM.111 and seedling at our university site. In that study, only M.9 without inter-grafting showed tree losses (15%) (Spornberger et al., Citation2018).
The observed tree losses (between 5–35%) and damages (between 10–40%) on some rootstocks in this study prove the susceptibility of ‘Topaz’ to crown rot and confirm the recommendation of an inter-grafting for M.9 as an effective prevention method. Following our observations, it is advisable to use an inter-stem or a higher grafting point with ‘Topaz’ also in combination with the tested Geneva-rootstocks (e.g. G.11, G.202 and G.41).
Root Suckers
During the observation period, M.7 showed a significantly higher rate of root suckers (75% of trees in 2018 and 80% in 2019) compared to all other rootstocks. In both years, also M.9 (30%), G.16 (20–25%) and MM.111 (6–29%) had some suckers (). These observations are broadly in line with those of Reig et al. (Citation2018) who reported a high rate of root suckers on M.7 when comparing 48 (33 of them were from Geneva) rootstocks grafted on Fuji apple cultivar. A low generation of root suckers is desirable to prevent highly time-consuming removal work. If infested by fire blight (Erwinia amylovora), root suckers can even lead to the death of the entire tree (Aldwinckle et al., Citation2004). However, the root suckers observed in our trial on M.7 did not correspond with negative effects on growth, yield or a higher share of tree losses.
Table 1. Results of the evaluations of root suckers in the years 2018 and 2019 (% of trees with root suckers and amount of root suckers)
Plant health
Disease and pest infestation (e.g. Dysaphis plantaginea) did not differ between the variants used in the study (data not shown). This is in line with other research projects about ‘Topaz’ on different rootstocks in organic production systems, where no differences in pest and disease could be found either (Spornberger et al., Citation2018; Wurm, Citation2018). In September 2019, we registered an obviously lower leaf vitality on CG.13 during the harvest compared to all other variants (data not shown).
Growth
After the first year (2011), M.7, G.16, MM.111 were growing vigorously, while G.202 was still very weak due to a comparably small tree size at planting. After four years, in 2014, MM.111 already showed the highest TCSA, followed by M.7 and G.16. All other variants grew more weakly at that time. In 2019, after nine years, MM.111 grew highly vigorously (75.0 cm2 TCSA), followed by M.7 (57.1 cm2) and G.202 (46.2 cm2). G.16 (41.9 cm2), CG.13 (42.1 cm2) and G.41 (41.3 cm2) were growing at the same vigor. Only G.11 (36.5 cm2) and M.9 (35.9 cm2) grew more weakly ().
Figure 2. Trunk circumference evolution (2011–2019) of 8 rootstocks grafted with ‘Topaz’ apple cultivar. Vertical lines indicate LSD (P < .05)
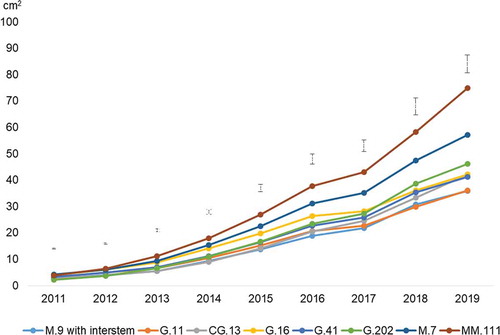
A recent trial with ‘Topaz’ in a replanted apple orchard at Klosterneuburg in Austria showed results that were contrary to those of this study. G.41 grew almost as strongly as M.7; and G.11 was clearly more vigorous compared to M.9 (Wurm, Citation2018). On the other hand, Lordan et al. (Citation2019) found nearly the same TCSA of the cultivar ‘Honeycrisp’ on G.41, G.11 and M.9 while the value was higher on G.202.
Yield
In 2012, we harvested only few fruits. In 2013, the first noteworthy yield was achieved, in the following order: G.11 (6.5 kg), G.16 (5.4), G.41 (4.6), M.9 (3.7), CG.13 (3.5), M.7 (2.9), MM.111 (2.2) and G.202 (1.7). In 2014, the harvest was low on all variants due to late frost. In 2015, the yields were above 10 kg for M.7, MM.111, G.41, G.202 and G.11 and slightly lower on M.9 and CG.13. In 2016, three days of late frost during flowering led to a complete yield loss. In 2017, the yield on M.7 and MM.111 was significantly higher than on the other rootstocks, which were located more closely to one another. After this very high yield, in 2018, we observed an alternate bearing, which was especially distinct on M.9 and MM.111 (). In the year 2019, a frost during flowering led to yield losses once again, especially in the lower tree parts. This led to bitter pit symptoms that were evaluated at harvest.
Figure 3. Yield (kg/tree) on the different rootstocks, 2012–19 (no harvest in 2016 due to frost). Vertical bars indicate the standard error
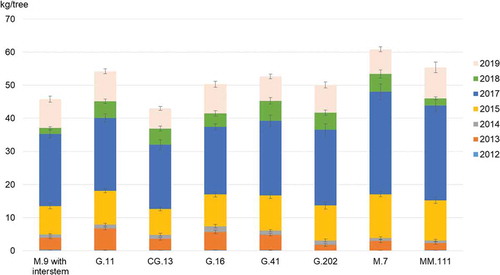
Across the duration of the study, M.7 showed the highest yield per tree, followed by MM.111, G.11 and G.41. Closely behind were G.16, G.202 and M.9, followed by CG.13 (). This corresponds to the results of a previous trial at our site without Geneva-rootstocks where ‘Topaz’ on M.7 showed the highest yields per hectare followed by M.26 and M.9 (Spornberger et al., Citation2018). In the study by Wurm (Citation2018), G.41 had the highest yield per tree, followed by M.7 and G.11, with M.9 at the bottom of the ranking.
If we also account for the planting distance, which varied in the row dependent on rootstocks vigor, G.11 had the highest total yield per hectare, significantly above that of G.16, M.9, G.41, CG.13 and M.7.
The cumulative yield efficiency (CYE) also includes the growth of trees. By CYE, G.11 ranked first, closely followed by M.9. Notably, G.11 was significantly different from G.41, as the latter was higher than CG.13, G.202 and M.7 (). These results are not corresponding to those of Wurm (Citation2018), where ‘Topaz’ on M.9 had the highest CYE, followed by G.41 and G.11. Lordan et al. (Citation2019) carried out a rootstock trial in New York State (USA) with the weaker growing apple cultivar ‘Honeycrisp’ on 31 rootstocks. Their results showed the highest CYE for G.11, closely followed by G.41 and M.9. As in our study, they also found G.202 to be less efficient. M.9 also showed a higher CYE compared to G.202 and M.7 in a rootstock trial with ‘Fuji’ in New York State (Reig et al., Citation2018). In the previous trial with ‘Topaz’ at our site without Geneva-rootstocks M.9 had the highest CYE, distinctly higher than M.7 and MM.111 (Spornberger et al., Citation2018).
Table 2. Sum of yields in t/ha from 2012–19, trunk cross-sectional area in the autumn of 2019 and cumulative yield efficiency per tree of ‘Topaz’, per rootstock
Fruit Size, Color and Fruit Quality after Storage
Over an average of six years, M.9 (172 g) and M.7 (170 g) showed a significantly higher single fruit weight compared to G.41 (161 g), G.11 (160 g), and CG.13 (159 g) while G.202 (169 g) and MM.111 (168 g) showed a slightly higher one. It should be noted that in single years, variants with very low yield showed particularly high values, e.g. G.202 in 2013 or M.9 in 2018 (). In a similar trial with ‘Topaz’, Wurm (Citation2018) did not find important differences in the single fruit weight among M.9, G.11, G.41, M.7 and some other rootstocks, except in single years caused by a low fruit set in some particular variants.
Table 3. Mean single fruit weight from 2013–19 (no harvest in 2016 due to frost) Single fruit weight g/fruit
In both years, the evaluation of fruit and weight losses after storage and the fruit color assessment did not show any relevant differences between rootstocks (data not shown).
The quality evaluation of fruits at harvest in 2017 showed a lower percentage of marketable fruits on CG.13 compared to all other variants (). In 2019, CG.13 also had the lowest share of marketable fruits (51.1%), which was significantly below M.9 (69.3%), MM.111 (67.9%) and G.202 (66.1%). In that year, G.11 had a higher share of bitter pit fruits (26%) compared to MM.111 (12.4%). In 2018, there were no differences between variants.
Table 4. Results of the fruit quality evaluation at harvest, 2017–19.
CG.13 showed a higher flesh firmness compared to all other rootstocks. G.11, G.41 and G.202 had significantly higher values than M.9 and G.16. Moreover, G.11, CG.13, G.16, G.202, MM.111 and M.9 had a higher soluble dry matter content than G.41 and M.7. We measured the highest acidity values on G.202 and M.9 – all other variants were significantly lower. G.202, M.9 and M.7 also had a very low sugar-acid ratio, while that of G.16 was quite high ().
Table 5. Mean values of the fruit quality assessments in 2015 and 2017 (two annual assessments in November and February)
Overall Discussion of the Tested Rootstocks
In this study, the standard M.9 with inter-stem showed good plant health with no tree losses, also in the conditions of replantation. The single-tree-yield was medium, while the specific-yield was excellent.
G.11 grew similarly to M.9 and performed slightly better in terms of yield results with the highest single-tree-yield and the highest CYE. For both metrics, the differences between G.11 and the other Geneva rootstocks were significant. These results conform with those of Kockerols et al. (Citation2009), Pfeiffer (Citation2016), Höller and Guerra (Citation2017) and Robinson and Fazio (Citation2019). In 2018, after the extremely high yield in 2017, we saw much less alternate bearing compared to M.9. The fruits showed a high flesh firmness. However, there were some tree losses on this rootstock, especially in the last year. It may therefore be advisable to use an inter-stem or a higher grafting-height to prevent crown rot for this rootstock in combination with the very sensitive cultivar ‘Topaz’.
The TCSA of CG.13, G.16 and G.41 was about 10% higher than that of M.9 and G.11, which is ideal for replanting orchards (Höller and Guerra, Citation2017). Single-tree-yield and yield efficiency of G.41 were at the same level as those of M.9, but lower than G.11. Wurm (Citation2018) found similar results in Klosterneuburg. However, this rootstock showed considerable tree losses at our site, which may indicate a susceptibility to Phytophtora cactorum in combination with the highly susceptible ‘Topaz’. We also recommend inter-stem-grafting for this rootstock with the cultivar ‘Topaz’.
CG.13 had a lower single tree yield and CYE compared to M.9. It showed a lower leaf-vitality compared to the other variants in September 2019, and many tree losses at the last assessment in May 2020. While the fruits have a high firmness, the share of marketable fruits was low in two years. Overall, it is not recommendable.
G.16 showed good yield results. The prevalence of root suckering was higher than on other Geneva rootstocks, but fewer trees were lost. However, G.16 is not recommendable due to its susceptibility to viruses and Eriosoma lanigerum as well as its scarce tolerance for replanting in the US (Auvil et al., Citation2011; Robinson and Fazio, Citation2019).
G.202 is not recommendable either: it grows rather strongly, starts late with yield and, in combination with ‘Topaz’, many trees were lost. Previous studies in Washington State (Auvil et al., Citation2011; Robinson and Fazio, Citation2019) also showed that it is much more vigorous and less productive compared to M.9.
The trees on M.7 grew about 25% more strongly than M.9 and G.11. They had the highest single-tree-yield, but could not compete with G.11, M.9 and G.41 in CYE. In spite of a strong tendency to build root suckers, the plant health of this rootstock was quite positive compared to all other rootstocks without inter-stem, as it suffered no losses. Sotiropoulos (Citation2006) and Spornberger et al. (Citation2018) already mentioned positive yield characteristics of M.7 in rootstock trials in dry sites. As Wurm (Citation2018) has mentioned, it may be an interesting alternative for modern fruit wall systems.
MM.111 showed the strongest growth (almost 50% more than M.9) and a late start of yield. Overall, it was clearly less productive than M.7, and therefore, it is not recommendable for any form of intensive growing (Spornberger et al., Citation2018; Wurm, Citation2018).
Acknowledgments
The authors of the study would like to thank the nursery Deimel in Ottendorf/Rittschein for the free supply of the trees for the trial and the staff of the research center Jedlersdorf for the maintenance of the orchard.
References
- Aldwinckle, H., N. LoGiudice, T.L. Robinson, H.T. Holleran, G. Fazio, W.C. Johnson, and J.L. Norelli. 2004. Resistance of apple rootstocks to fire blight infection caused by internal movement of Erwinia amylovora from scion infections. Acta Hortic. 663:229–234. doi: 10.17660/ActaHortic.2004.663.36.
- Auvil, T.D., T.R. Schmidt, I. Hanrahan, F. Castillo, J.R. McFerson, and G. Fazio. 2011. Evaluation of dwarfing rootstocks in Washington apple replant sites. Acta Hortic. 903:265–271. doi: 10.17660/ActaHortic.2011.903.33.
- Fazio, G., T.L. Robinson, and H.S. Aldwinckle. 2015. The Geneva apple rootstock breeding program. Plant Breed. Rev. 39:379–424. doi: 10.1002/9781119107743.ch8.
- Gasser, S., A. Naef, and P. Monney. 2009. Nachbauprobleme in der Apfelproduktion. Schweiz. Z. Obstbau Weinbau 10:8–10.
- Hewavitharana, S., T. DuPont, and M. Mazzola. 2019. Apple replant disease, WSU tree fruit IPM strategies, Washington State tree fruit extension fruit matters. 9 Dec. 2019. http://treefruit.wsu.edu/crop-protection/disease-management/apple-replant-disease/
- Höller, I., and W. Guerra. 2017. Erfahrungen mit der Apfelunterlage G 11 in Südtirol. Obstbau- Weinbau 10:14–18.
- Hornig, R., and M. Paul. 2011. Sorten-Unterlagen-Kombinationen für den Mostapfelanbau. Erwerbs-Obstbau 53(2):59–67. doi: 10.1007/s10341-011-0130-y.
- Khazaei, J., G. Chegini, and M. Bakhshiani. 2008. A novel alternative method for modelling the effect of air dry temperature and slice thickness on quality and drying kinetics of tomato slices: Superposition technique. Drying Technol. 26(6):759–775. doi: 10.1080/07373930802046427.
- Kockerols, M., S. Egger, P. Monney, B. Duffy, and S. Gasser. 2009. Feuerbrandtolerante Unterlagen. Schweiz. Z. Obstbau Weinbau 2:6–8.
- Lindner, L. 2009. Phytophthora cactorum als Verursacher von Rinden- und Fruchtschäden am Apfel. Obstbau- Weinbau 9:312–315.
- Lordan, J., G. Fazio, P. Francescatto, and T.L. Robinson. 2019. II. Horticultural performance of ‘Honeycrisp’ grown on a genetically diverse set of rootstocks under Western New York climatic conditions. Sci. Hortic. 257:108686. doi: 10.1016/j.scienta.2019.108686.
- Marini, R.P., B. Black, R.M. Crassweller, P.A. Domoto, C. Hampson, R. Moran, T.L. Robinson, M. Stasiak, D.L. Ward, and D. Wolf. 2014. Performance of Golden Delicious apple on 23 rootstocks at eight locations: A ten-year summary of the 2003 NC-140 dwarf rootstock trial. J. Am. Pomol. Soc. 68(2):54–68.
- Mazzola, M. 1998. Elucidation of the microbial complex having a causal role in the development of apple replant disease in Washington. Phytopathology 88:930–938. doi: 10.1094/PHYTO.1998.88.9.930.
- OECD. 2005. Internationale Normung von Obst und Gemüse, Leitfaden zu objektiven Testmethoden zur Bestimmung der Qualität von Obst und Gemüse sowie Trocken- und getrockneten Erzeugnissen. https://www.ble.de/DE/Themen/Ernaehrung-Lebensmittel/Vermarktungsnormen/Obst-Gemuese/Vermarktungsnormen-Hilfen-zur-Anwendung/OECD-Leitfaden.html
- Pfeiffer, B. 2016. Comparison of rootstocks Geneva 16, M.9 and G.11 under organic cultivation at the LVWO Weinsberg - actualized results 2009–2015. Proceedings of the 17th international conference on organic fruit-growing from 15–17 February 2016, pp. 144–148. Hohenheim, Germany.
- Reig, G., J. Lordan, G. Fazio, M.A. Grusak, S. Hoying, L. Cheng, P. Francescatto, and T.L. Robinson. 2018. Horticultural performance and elemental nutrient concentrations on ‘Fuji’ grafted on apple rootstocks under New York State climatic conditions. Sci. Hortic. 227:22–37. doi: 10.1016/j.scienta.2017.07.002.
- Robinson, T.L., and G. Fazio. 2019. Picking the right rootstock for fresh and processing apple orchards. N Y State Fruit Q. 28:5–10.
- Ruess, F. 2010. Intensiver Mostobstanbau. Obstbau Garten 10:168–171.
- Sotiropoulos, T.E. 2006. Performance of the apple cultivar ‘Golden Delicious’ grafted on five rootstocks in Northern Greece. Arch. Agron. Soil Sci. 52:347–352. doi: 10.1080/03650340600612532.
- Spornberger, A., E. Schuller, E. Videki, and G. Vegvari. 2018. Influence of rootstocks on vegetative and generative characteristics of the apple cultivar ‘Topaz’ according to organic production conditions in Eastern Austria. Erwerbsobstbau 60(3):231–238. doi: 10.1007/s10341-017-0363-5.
- Thalheimer, M. 2016. Nachbau beim Apfel - Wie kann die Wüchsigkeit der Jungbäume verbessert werden. Obstbau/Weinbau 1:9–12.
- Thybo, K., M. Edelenbos, L. Christensen, J. Sørensen, and K. Thorup-Kristensen. 2006. Effect of organic growing systems on sensory quality and chemical composition of tomatoes. LWT Food Sci. Technol. 39:835. doi: 10.1016/j.lwt.2005.09.010.
- Wurm, L. 2018. Einfluss von feuerbrandresistenten Unterlagen auf vegetative und generative parameter bei der Apfelsorte ‘Topaz’ auf Nachbauflächen unter biologischen Bedingungen. Mitt. Klosterneuburg 68:293–301.
- Yim, B., F.S. Hanschen, A. Wrede, H. Nitt, M. Schreiner, K. Smalla, and T. Winkelmann. 2016. Effects of biofumigation using Brassica juncea and Raphanus sativus in comparison to disinfection using basamid on apple plant growth and soil microbial communities at three field sites with replant disease. Plant Soil 406:389–408. doi: 10.1007/s11104-016-2876-3.