ABSTRACT
The cultivar ‘G × N-9’ is an almond x peach hybrid rootstock, the main characteristic of which is resistance to several root-knot nematodes (Meloidogyne spp.). Although its propagation by hardwood cutting in the fall is recommended, optimization of ‘G × N-9’ in vitro propagation is currently needed. Thus, the objective of this study was to evaluate the effect of the cytokinins [6-benzylaminopurine (BAP) and Kinetin (KIN)], and the carbohydrate sources (sucrose and sorbitol) on shoot proliferation and elongation, as well as the influence of indole-3-butyric acid (IBA) on in vitro rooting and acclimatization of ‘G × N-9’ rootstock. This study reports enhanced on shoot proliferation and elongation based on the interaction between BAP and KIN. When comparing the effects of sorbitol and sucrose, sorbitol at 15 and 30 mg L−1 was the most effective for the induction of shoot proliferation (5.75 ± 0.19 and 6.39 ± 0.21 shoots per explant, respectively). The highest number of roots per explant was in QL medium supplemented with 0.6 and 1.2 mg L−1 IBA. After 30 days of acclimatization, the treated-plants with 1.2 mg L−1 IBA showed the smallest root length, but the higher number of roots per explant (8.35 ± 0.41). The successful acclimatization of plantlets depended on the use of IBA during in vitro culture, to induce a healthy and well-developed root system.
Introduction
In the last decade, there has been a tendency to reduce the area planted with peach trees (Prunus persica L. Bastch – Rosaceae) in Brazil, while production and consumption have remained constant (219,59 thousand tons). The Rio Grande do Sul (RS) state is the largest peach producer in the country, producing 146,43 thousand tons, in 2018 (IBGE Citation2020), however, compared to other Brazilian states, the average productivity in RS is the lowest among them, mainly due to the low genetic quality of the rootstocks used by murseries (Radmann et al., Citation2011).
In the main fruit crops, propagation is usually carried out vegetatively by grafting a desired cultivar onto a rootstock. At global level, there is a tendency to use rootstocks propagated vegetatively. In Brazil, peach seedlings have traditionally been used as rootstocks, and generally, they are derived from a mixture of peach stones discarded by the canning industry, resulting in seedlings with low quality for use as rootstocks, which added to other biotic and abiotic stresses, cause an imbalance between orchard plants (Oldoni et al., Citation2019; Radmann et al., Citation2011).
Rootstock quality has a fundamental influence on the growth, productivity, and phytosanitary status of the tree. The success of one peach orchard is directly related to the use of rootstocks with ameliorated characteristics such as disease-free rootstocks; efficiency to uptake water and nutrients; resistance to soil-borne diseases and pests (Gainza et al., Citation2015). Some peach rootstocks have been tested in Brazil for their response to soil pests, and the cultivar ‘G × N-9’ (Prunus dulcis Mill. Bastsch × Prunus persica L.) has potential to be used in our country, especially because of its resistance to root-knot nematodes (Meloidogyne spp.) (Rossi et al., Citation2002), that are obligate endoparasite considered the most damaging nematode group occurring in the warmer peach-growing regions in the world, causing significant production losses each year (Esmenjaud et al., Citation2009). ‘G × N-9’ is also well adapted to the climatic conditions of the RS. Furthermore, it displays complete compatibility with numerous almond, peach, and nectarine cultivars (Felipe, Citation2009).
‘G × N-9’ can be propagated by hardwood cuttings with excellent results (Felipe, Citation2009). However, researchers have further contributed to the development of propagation techniques for Prunus species (Alanagh et al., Citation2014; Cheong, Citation2012; Gainza et al., Citation2015). In vitro propagation, or ‘micropropagation’, has several advantages over traditional propagation methods: it is independent of seasonal variation; produces genetically equal plants with high phytosanitary qualities; and can result in a large number of plants in a short period of time (Fenning, Citation2019; García-Gonzáles et al., Citation2010; Phillips and Garda, Citation2019). Many fruit crops already have well-defined protocols for in vitro multiplication (Abbasi et al., Citation2019; Surakshitha et al., Citation2019), but some woody plants, such as plums and peach trees, remain unstudied. There are a limited number of reports on the micropropagation of ‘G × N’ Series rootstock (Arab et al., Citation2016, Citation2014; Radmann et al., Citation2009b) and as far we know no protocol has been developed for the micropropagation of the ‘G × N-9’ peach rootstock.
Many factors can influence the success of in vitro culture of peach rootstocks. Beyond the basic medium, the choice of the Plant Growth Regulators (PGR) and source of the carbohydrates are key factors for the growth and development of in vitro cultured plants (Arab et al., Citation2014; Phillips and Garda, Citation2019). The cytokinins are PGR that promote cell division and stimulate shoot development in vitro, and the most used cytokinins are 6-benzylaminopurine (BAP) and kinetin (KIN) (Phillips and Garda, Citation2019). In its turn, auxins are mainly able to induce the explants rooting. For this reason, the natural occurring auxin indoleacetic acid (IAA) and synthetic auxin analogs, such as indole-butyric acid (IBA) and 1-naphthylacetic acid (NAA), are commonly used (Mansseri-Lamrioui et al., Citation2011; Zhou et al., Citation2010).
In the early stages of in vitro cell, tissue, and organ culture, the explants are heterotrophic, and they are unable to produce their own sugars (Nowak et al., Citation2004; Phillips and Garda, Citation2019). Therefore, an exogenous source of carbohydrate in the culture medium supplies the required energy for biosynthetic metabolic reactions and as osmotic agents (Eckstein et al., Citation2012; Pahnekolayi et al., Citation2019; Phillips and Garda, Citation2019; Yaseen et al., Citation2012; Zhang et al., Citation2017). Several carbohydrates have been used as an exogenous source of energy in in vitro condition, including sucrose (Naik et al., Citation2010; Siwach et al., Citation2011), sucrose-derived hexoses (glucose and fructose) (Bahmani et al., Citation2009; Cheong and An, Citation2015; Eckstein et al., Citation2012; Gubis et al., Citation2005; Kalinina and Brown, Citation2007), and polyols (sorbitol and mannitol) (Nacheva and Gercheva, Citation2009; Pathak and Dhawan, Citation2012; Ruzic et al., Citation2008). Indeed, the results were different according to the source, concentration, species and even within a species.
Sucrose is the source of carbon traditionally used for in vitro propagation (Ahmad et al., Citation2007; Phillips and Garda, Citation2019; Yaseen et al., Citation2012), because it is the predominant carbohydrate in the phloem sap of the majority of plant species (Pathak and Dhawan, Citation2012). However, sorbitol is the predominant sugar produced by source-leaves and translocated in the phloem of Rosaceae plants (Ahmad et al., Citation2007; Kanayama et al. 2009; Kim et al., Citation2015). Therefore, studying the best carbohydrate source for the in vitro propagation of Prunus spp. can be a powerful lever to successfully manage the growth and development of these plants, under controlled conditions.
Large-scale in vitro propagation of some peach rootstocks has been deployed in several countries. However, in Brazil, this method has not been successful for peach rootstock propagation, and information in the literature is limited. Thereby, developing protocols for the micropropagation of the G × N Series, and other peach rootstocks, is an important challenge yet to overcome in this country. The objective of this study was to evaluate the effect of two cytokinins (BAP and KIN) and two carbohydrates (sucrose and sorbitol) on the induction of shoot proliferation, as well as the influence of IBA concentrations on the rooting and acclimatization of the ‘G × N-9’ peach rootstock.
Materials and Methods
Plant Material and In Vitro Culture of ‘G × N-9’ Peach Rootstocks
Juvenile plants of the ‘G × N-9’ peach rootstock were produced by grafting and cultured in a greenhouse for 1 year. Vegetative buds for grafting were taken from plants maintained as genetic resources since 1998, in the Germplasm Collection of Peach Rootstocks of the Universidade Federal de Pelotas, Brazil. In our laboratory, one-node explants of the peach rootstocks ‘G × N-9’ (0.5 cm lenth) were taken in spring and established in vitro on QL medium (Quoirin and Lepoivre, Citation1977) supplemented with mio-inositol (100 mg L−1), sucrose (30 g L−1), and agar (7 g L−1), at pH 5.8 (QL medium to establishment), without PGRs. Thereafter, the explants were transferred to the growth-room and kept at 25°C ± 2°C, in the dark for 7 days, and then transferred to a photoperiod of 16 hours and a light flux density of 48 μmol m−2 s− 1. After 4 weeks, shoots that arose from nodes were transferred to a fresh MS medium with 0.5 mg L−1 BAP, 0.01 mg L−1 IBA, pH 5.8 (MS medium to shoot proliferation), in order to obtain the enough amount of explants in vitro to establish the subsequent experiments.
Effect of Source and Concentration of Cytokinins on Shoot Multiplication and Elongation
Shoots approximately 2.0 cm long (4-week-old in vitro-grown) were placed into flasks containing 40 mL of medium (QL medium to establishment) and supplemented with different combinations of 6-Benzylaminopurine (BAP) and kinetin (KIN), according to the treatment described in . For all treatments, 0.01 mg L−1 indole-3-butyric acid (IBA) was added to the medium. Flasks were incubated under the same light and temperature conditions described above. After 4 weeks of incubation, the multiplication and elongation rates were measured, based on the number of shoots produced per explant and the length of shoots.
Table 1. Number of shoots per explant and shoot length of ‘G × N-9’ cultivated for 30 days in QL (Quoirin and Lepoivre) medium with different combinations of cytokinin concentrations
Effect of Source and Carbohydrate Concentration on Shoot Multiplication and Elongation
Flasks containing 40 mL of medium (QL medium to establishment), constituting the following treatments: sucrose (15, 30 and 45 g L−1); and sorbitol (15, 30 and 45 g L−1) were used for testing the impact by using 2.0 cm long shoots (without apex) as explants. For all treatments, 0.4 mg L−1 BAP and 0.01 mg L−1 IBA were included in the medium. Flasks were incubated under the same light and temperature conditions as in the in vitro establishment. After 4 weeks of incubation, the multiplication and elongation rates were measured, based on the number of shoots produced per explant and the length of shoots.
Effects of Different Auxin (IBA) Concentrations in In Vitro Rooting and Acclimatization
Shoots were placed into flasks (the same as above) and supplemented with IBA concentrations (0.0, 0.3, 0.6, and 1.2 mg L −1). The flasks remaining in the dark for 5 days and then kept for 10 days in a photoperiod of 16 hours. At the end of the 15-day cultivation in rooting medium, the number roots per explant, length of roots and shoots (cm), and percentages of rooting were evaluated. Afterward, all the rooted explants were transferred to plastic trays (1 L capacity), containing vermiculite of medium granulometry, and kept in a greenhouse at 25°C and 80% relative humidity (RH). After 30 days of acclimatization, the number of roots per explant, length of roots and shoot, and percentage of surviving plants were evaluated.
Statistical Analysis
The experiment was arranged with a completely randomized factorial design. All experiments were constituted by four repetitions per treatment, each one represented by a flask with five explants. Results correspond to mean ± standard deviation. Data were analyzed by ANOVA and the significant differences were detected using Tukey, HSD at p ≤ 0.05, using the WinStat 2.0 software (Machado and Conceição 2005).
Results and Discussion
Effect of Source and Concentration of Cytokinins on Shoot Multiplication and Elongation
The demand for new and different Prunus rootstocks, including G × N Series, is increasing, due to their recognized agronomical advantages. However, current propagating techniques need to be optimized to fully exploit the potential of these new genotypes. In vitro culture can improve the multiplication efficiency of these rootstocks, which may save up much time in the production of a grafted tree (Nasri et al., Citation2019). The growth and multiplication of shoots in vitro are affected by several factors, such as salt composition, plant growth regulators, and carbon sources of the medium (Phillips and Garda, Citation2019). In this sense, the source and optimal concentrations of cytokinins for multiplication is genotype-dependent, and a small shift of the optimal can cause adverse effects (Radmann et al., Citation2011). In addition to its role on shoot multiplication rates, cytokinins also influence rooting, acclimatization, and the plant quality obtained in vitro (Lata et al., Citation2016).
In line with the previous statements, Experiment 1 was performed. In relation to the number of shoots per explant, the highest mean values were observed when the highest concentrations of BAP (without KIN) were used, i.e., 3.68 ± 0.50 (M5). However, there was no significant difference in shoot number between Medium 5 and Mediums 3 and 4, which showed 3.16 ± 0.25 and 2.61 ± 0.22 shoots per explant, respectively (). KIN alone was not very efficient in inducing shoot multiplication in the ‘G × N-9’ peach rootstock.
Previous studies with Prunus rootstocks have used multiplication media with BAP concentrations ranging from 0.1 to 6.0 mg L−1 (Aghaye and Yadollahi, Citation2012; Arab et al., Citation2014; Teixeira et al., Citation2004; Wagner et al., Citation2003). There is a clear positive effect of BAP at higher concentrations, combined with low concentrations of KIN, on the proliferation of shoots in ‘G × N-9’ peach rootstocks (). In contrast, Srivastava and Joshi (Citation2009) demonstrated that the concentration of KIN must be higher than BAP for the optimum multiple shoot formation and elongation in Portulaca grandiflora, suggesting that the optimum concentration of PGR for inducing shoot proliferation is species-dependent.
Changes in proliferation rates under different concentrations of BAP for Prunus rootstocks were reported by Radmann et al. (Citation2009a), mainly for the Tsukuba 1 peach rootstock, whose presence of 1.0 mg L−1 BAP in the culture medium provided the highest number of shoots (1.25 per explant). Similar results were obtained by Aghaye and Yadollahi (Citation2012) in the GF 677 rootstock using 1 mg L−1 BAP (2.98 per explant). By comparing the effects of different cytokinin sources, Radmann et al. (Citation2011) demonstrated that BAP is the best one, and 4.0 mg L−1 is the optimal BAP concentration for multiplication of the ‘Flordaguard’ peach rootstock. On the other hand, Rodrigues et al. (Citation2003) reported that 0.6 mg L−1 BAP (a lower concentration than normally tested) increased the number of shoots in the Prunus rootstock Marianna 2624, Myrobalan 29-C, Mr.S. 2/5 and GF 677.
There are conflicting reports on the optimal BAP concentration for shoot proliferation in peach rootstocks. There is a wide variability in the responses to BAP and each genotype is likely to have a different ideal type and concentration. Phillips and Garda (Citation2019) attribute these differences to the interaction of endogenous phytohormones within the tissues, with the exogenously supplied PGR affecting each genotype distinctly. The results of our study (BAP induced a higher shoot number than KIN at a concentration of 4.0 mg L−1, reaching the average values of 3.68 ± 0.50 shoots per explant) showed how much these requirements vary when comparing the two cytokinins. It is relevant that increasing the BAP concentration does not always increase the shoot number but may lead to physiological disorders, such as hyperhydricity, and to the decrease in shoot formation and growth over repeated subculturing (Radmann et al., Citation2009a).
The maximum shoot length was obtained with Mediums 2 and 3, with values ranging from 0.62 ± 0.09 to 0.72 ± 0.15, respectively, whereas lower values were found at higher concentration of KIN (M1), as well as with Medium 4 (0.1 mg L−1 KIN + 0.3 mg L−1 BAP). This indicates that BAP at higher concentrations enhances shoot number, but is less effective on shoot elongation. Less shoot length was also observed with the increase in BAP concentration in other cultivars of the Prunus, as such ‘CARELLI’ and ‘G × N-15’ peach rootstocks (Arab et al., Citation2014; Teixeira et al., Citation2004). The reduced length of shoots is related to competition among them. Another possible reason is the increase in the endogenous production of ethylene, caused by the exogenous application of high concentrations of cytokinins in in vitro culture (Saha et al., Citation2007). Many authors have reported a higher production of ethylene by explants grown in culture medium supplemented with a high BAP concentration (Ashraf et al., Citation2014), due, in part, by an increase in ACC synthase enzyme activity (Saha et al., Citation2007). Moreover, ethylene production also increases in in vitro culture as consequence of explant wounding (Gammoudi et al. 2019). Unfortunately, most studies to date have focused on the role of ethylene biosynthesis inhibitors in in vitro culture, without investigating the effect of ethylene levels on shoot proliferation (Park et al., Citation2016). Indeed, more studies are necessary to understand the role of ethylene, and its interaction with other PGRs, in plant tissue culture.
Regarding the length of shoots, although concentrations of Medium 2, 3, and 4 were higher than other media (), the mean values were low compared to results obtained by other authors (Radmann et al., Citation2011; Rocha et al., Citation2009; Teixeira et al., Citation2004). Small shoots are extremely detrimental in in vitro multiplication and may present a low percentage of rooting, which causes poor growth (Rocha et al., Citation2009). In this sense, further research is needed on how to meet the demand for Prunus rootstocks, including G × N Series, with high genetic and sanitary quality. The aim is to achieve not only a high rate of shoot proliferation, but also better elongation of shoots and more subcultures, by optimizing the concentrations of cytokinins and other factors in growth media.
Effect of Source and Concentration of Carbohydrate on Shoot Multiplication and Elongation
To evaluate the source and concentration effects of carbohydrate in QL medium on shoot proliferation of the peach rootstock ‘G × N-9’, Experiment 2 was proposed. Results showed there were significant interactions between factors for the number of shoots per explant, after 4-week of culture only, while for shoot length, we only observed differences for the mean factor concentration, as shown in . When comparing the effect of the different carbohydrate sources (sucrose and sorbitol) at the same concentration, the QL media supplemented with 15 and 30 g L−1 sorbitol produced the highest mean number of shoots per explant (5.75 ± 0.39 and 6.39 ± 0.31, respectively). This result demonstrates that sorbitol plays an effective role in the in vitro multiplication of the rootstock ‘G × N-9’, possibly because it is the main carbohydrate translocated in the phloem (Ahmad et al., Citation2007). This behavior may also be associated with the greater activity of enzymes associated with sorbitol synthesis and degradation. According to Kadota et al. (Citation2001), the preferential use of sorbitol by some stone fruit rootstocks is due to the availability of enzymes that help to hydrolyze this carbohydrate. Research findings point to the importance of sorbitol as an exogenous source of carbohydrate for species from the genera Malus, Pyrus and Prunus, both belonging to the Rosaceae family (Cheong and An, Citation2015; Nacheva and Gercheva, Citation2009).
Table 2. Number and length of shoots obtained with the ‘G ×ᵡ N-9’ rootstock in vitro-grown for 30 days in QL medium with different concentrations of sucrose and sorbitol
During the in vitro multiplication of the peach rootstock ‘GF-677’, Ahmad et al. (Citation2007) observed that sorbitol was a more effective source of carbon than sucrose, producing the most shoots at a concentration of 30 g L−1. However, when studying the multiplication of another Prunus cultivar ‘Scharttenmorelle’, Borkowska and Szczerba (1991) found that sucrose resulted in twice the number of shoots compared to sorbitol. However, for the ‘North Star’ cultivar, the authors did not observe a significant difference in the number of shoots between the two sources of carbohydrate. This variation in source and carbohydrate concentration effect demonstrates that the results are genotype-dependent; although some studies present sorbitol as the best carbohydrate source (Ahmad et al., Citation2007; Kadota et al., Citation2001; Nacheva and Gercheva, Citation2009), others report that sorbitol is not the best exogenous source of carbohydrate (Yaseen et al., Citation2009).
When comparing the effects of different concentrations of each carbohydrate source, we verified that the number of shoots per explant increases with increasing sucrose concentration, ranging from 2.58 ± 0.72 to 5.95 ± 0.24, in medium supplemented with 15 g L−1 and 45 g L−1 sucrose, respectively. A significant number of researchers advocate the positive impact of 30 g L−1 sucrose on shoot proliferation (Pérez-Jimenez et al., Citation2012). However, in our study, the 45 g L−1 sucrose provided the highest number of shoots. In relation to sorbitol concentration, we found no significant difference in the number of shoots per explant using 15 g L−1 and 30 g L−1 sorbitol, but these concentrations promoted more shoots per explant, compared to 45 g L−1 sorbitol ().
The variance analysis for shoot length showed no significant interaction between concentration and source of carbohydrates, but differences occurred individually for each factor. The mean values for shoot length were higher in the explants cultured in the medium with sucrose (0.74 ± 0.15), compared to sorbitol (0.59 ± 0.07). When considering only for the effect of different concentrations (independent of the source), it was observed that a concentration of 30 g L−1 is the most effective in increasing the length of shoots, only comparing with the concentration of 15 g L−1 ().
Although sorbitol is the preferred sugar for in vitro shoot proliferation (number and length), in some species of the Rosaceae family, a mixture of sugars may be necessary for the elongation of shoots, and this may explain, in part, the low values obtained for the length of shoots. Future researches should test a combination of at least two carbon sources in different proportions until the optimum results are obtained. Thus, the real effects of different carbon sources on the growth of explants during in vitro proliferation will be sufficiently understood with further studies. In fact, the research will not be complete until understand how much sugar is produced endogenously, and how transcription profile of genes encoding key enzymes related to sugar synthesis and degradation are regulated and how these changes are translated into metabolite level.
Plants of the Rosaceae family have enzymatic and molecular apparatus that enable the synthesis, transport, and use of sorbitol – a monosaccharide (sugar-alcohol) synthesized in source-leaves from glucose-6-phosphate, which is converted to sorbitol-6-phosphate by the enzyme sorbitol-6-phosphate dehydrogenase (S6PDH), then dephosphorylated to sorbitol by the enzyme sorbitol-6-phosphatase (S6P) (Kanayama, Citation2009). In sink tissues, the enzyme sorbitol dehydrogenase (SDH) converts sorbitol to fructose, which can rise the carbohydrate metabolism (Kanayama, Citation2009). Several research groups have studied the influence of these sugars on the expression of the genes encoding these enzymes (Cheong and An, Citation2015; Ozudogru et al., Citation2017). However, more studies are needed, especially considering the influence of these sugars on the expression of the genes encoding the enzymes, as well their effect on metabolism in in vitro cultured plants. In this sense, our research group is now working in this field and is going to publish our findings soon. From preliminary findings, we can state that key genes involved in the metabolism of sugars vary their expression pattern depending on the source and dose of carbon used, throughout the growing time.
Effect of IBA Concentration on In Vitro Rooting
Choosing the ideal type and concentration of PGR is one of the most important steps for in vitro culture propagation (Phillips and Garda, Citation2019; Ruzic and Vujovic, Citation2008). In this sense, exogenous auxins are required in the early stages to stimulate rhizogenesis (Radmann et al., Citation2014) and the emergence of numerous roots (Dobranszki and Silva, Citation2010). Various synthetic auxins may be used for this purpose, such as indole-3-butyric acid (IBA) and naphthaleneacetic acid (NAA); and indole-3-acetic acid (IAA) is the main type of the natural occurring auxin in plants (Phillips and Garda, Citation2019). However, for Prunus spp. IBA is reported to be the most efficient for root inducing (Pruski et al., Citation2005; Sadeghi et al., Citation2015; Zhou et al., Citation2010). IBA slow migration and delayed degradation are potential reasons for its better performance compared to NAA and IAA (Mansseri-Lamrioui et al., Citation2011). Obtaining a good root system could have an impact on the ability to clone rootstocks and ensure plant availability regardless of the time of year. Hence, Experiment 3 was performed, aimed at evaluating the effect of IBA concentration on in vitro rooting. For this, the QL medium was supplemented with different levels of IBA (0.0, 0.3, 0.6, and 1.2 mg L−1), and the number of roots per explant, length of roots and shoots (cm), and percentage of rooting were evaluated ().
Figure 1. Effects of different IBA concentrations on root induction and elongation in ‘G × N-9’ peach rootstocks. (a) Mean number of roots per explant, (b) Mean length of roots and shoots, and (c) rooting percentage (%). Formation of roots after 15 days in QL medium, supplemented with (d) 0.0 mg L−1 IBA, (e) 0.3 mg L−1 IBA, (f) 0.6 mg L−1 IBA, and (g) 1.2 mg L−1 IBA. Columns with different uppercase letters indicate significant differences between treatments based on ANOVA followed by Tukey test (P ≤ 0.05)
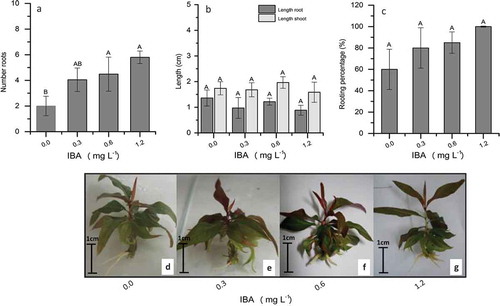
After in vitro cultivation for 15 days, it was observed the highest number of roots in QL medium supplemented with 0.6 and 1.2 mg L−1 IBA, ranging from 4.48 ± 1.83 to 5.80 ± 0.48, respectively (), in comparison with the control. The increased rooting induction response to IBA may be due to the slower oxidization rate by the oxidase (Wolella, Citation2017), becoming IBA more stable when compared to other auxins (Abbasi et al., Citation2019). Several studies have tested IBA concentrations on rooting. Kassaye and Bekele (Citation2015) observed the highest percentage of root induction, and the highest number and length of roots in media supplemented with 1.0 mg L−1 IBA in P. salicina. Similar results were found for other Prunus spp., such as GF305 peach (Kalinina and Brown, Citation2007), Gisela 5 cherry (Vujovic et al., Citation2012), wild cherry (P. avium L.) (Mansseri-Lamrioui et al., Citation2011), P. salicina Lindl. (Bandeira et al., Citation2012), and P. domestica (Wolella, Citation2017). With the peach selection VP411, Rogalski et al. (Citation2003a) obtained the highest numbers of roots with an IBA concentration of 0.9 mg L−1. Although it is well known that high concentrations of auxin induce ethylene synthesis, and this may be highly detrimental to the formation of adventitious roots, must consider that, for some species, a higher concentration is needed for the induction of rooting, as seen for the rootstocks ‘Capdeboscq’ (Rogalski et al., Citation2003a) and ‘GF677’ (Aghaye and Yadollahi, Citation2012).
Concerning root and shoot length, the different IBA concentrations did not significantly affect mean values (), probably due to the short maintenance time of the explants in the culture medium. Although there was no statistical difference in rooting percentage, the highest mean values were observed for 1.2 mg L−1 IBA (), but the plants were successfully rooted in all IBA concentrations, including in the control treatment ().
Effect of IBA Concentration on Acclimatization
The number of roots is a significant factor for increased percentages of plant survival during acclimatization; however, many plants derived from in vitro rooting do not survive when transplanted to ex vitro conditions, even when well rooted. Thus, in order to understand how the different IBA concentrations (0.0, 0.3, 0.6, and 1.2 mg L−1, used during in vitro rooting), affected acclimatization, a final experiment (Experiment 4) was performed. After 30 days of acclimatization, we evaluated the number of roots per explant, length of roots and shoots (cm), and the percentage of surviving plants, and the results are shown in . The number of roots increased progressively, reaching mean values of 8.35 roots per explant at an IBA concentration of 1.2 mg L−1 (), while mean root length was the smallest at this concentration (). A similar trend for Gisela 6 rootstock was previously reported by Hossini and Moghadam (Citation2010). The highest concentrations of IBA encouraged tissue lignification, which can lead to inhibited root elongation during the acclimatization phase. Baker and Wetzstein (Citation2004) reported that the effect of auxin on root growth is promotive at low concentrations and inhibitory at high concentrations.
Figure 2. Rooting response of ‘G × N-9’ peach rootstocks to different levels of IBA (0.0–1.2 mg L−1) after a 30 days acclimatization. (a) Mean number of roots per explant, (b) mean length of roots and shoots, and (c) percentage of surviving plants (%). Plantlets grown ex vitro from the (d) control treatment and (e) 0.3 mg L−1 IBA, (f) 0.6 mg L−1 IBA, and (g) 1.2 mg L−1 IBA. Columns with different uppercase letters indicate significant differences between treatments based on ANOVA followed by Tukey test (P ≤ 0.05)
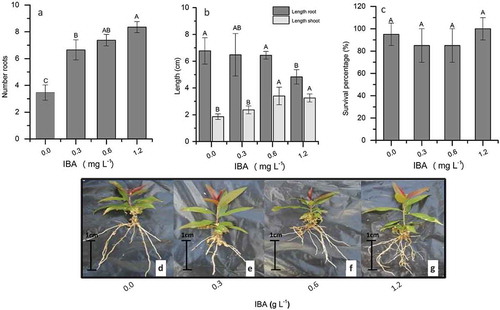
In our study, poor shoot growth and development was observed in the absence of IBA and at an IBA concentration of 0.3 mg L−1. According to Rogalski et al. (Citation2003b), this phenomenon may be directly related to the effect of IBA on explant apex growth and/or the occurrence of competition between explant apex and root growth. In relation to the percentage of surviving plants (), although not statistically differences were observed, the results were encouraging, probably due to good quality aerial parts and root systems formed during in vitro growth (-g). Similar results have been reported previously for most rootstocks (Vujovic et al., Citation2012; Zhou et al., Citation2010), except Gisela 5 (Rogalski et al., Citation2003a, Citation2003b).
Overall, there are a limited number of reports on the micropropagation of the G × N Series peach rootstock. However, some authors have mentioned that these rootstocks can be micropropagated in vitro and by cuttings (Arab et al., Citation2014; Felipe, Citation2009; Silveira et al., Citation2001). In order to fill this gap, this paper is the first to report on the micropropagation of ‘G × N-9’ from the in vitro establishment until obtaining plantlets with high survival rate. In this sense, has outlined the methods and practices adopted and highlighted advances made to the in vitro propagation of this rootstock through a schematic model of the in vitro establishment and shoot proliferation, as well as of the implementation of Experiments 1–4, and the main results found in each of them. These protocols will be helpful for the mass propagation of the G × N-9 rootstock, and they can be used to provide high multiplication rates of uniform plantlets for research purposes. However, the availability of this genotype to nurseries and peach producers can only be made in accordance with the breeder’s rights (Felipe, Citation2009).
Figure 3. Schematic representation of the morphological responses to different concentrations of cytokinins (BAP and KIN) (Experiment 1), and of carbohydrates (sucrose and sorbitol) (Experiment 2) on shoot proliferation (number and length of shoots) and effect of different levels of IBA (0.0–1.2 mg L−1) on rooting (Experiment 3) and acclimatization (Experiment 4) of ‘G × N-9’ peach rootstocks
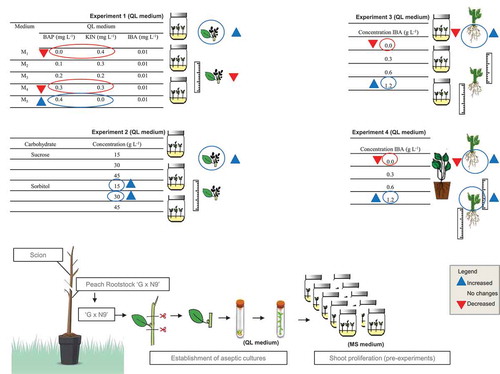
Conclusions
In this study, we concluded that the highest concentrations of BAP enhanced shoot number and, in combination with KIN, working synergistically to increase shoot length. In addition, sorbitol 15 and 30 g L−1 proved to be effective in inducing a higher number of shoot proliferation per explant than sucrose. Regarding in vitro rooting, media containing 0.6 and 1.2 mg L−1 IBA had the optimal combination for the most roots per explant. Moreover, a good result was found for the percentage of surviving plants, probably due to the quality of the aerial part and the root system, formed during in vitro culture. Overall, the results of this study provide a reproducible in vitro propagation method of direct organogenesis for ‘G × N-9’ peach rootstocks
Acknowledgments
The authors gratefully acknowledge the Professor Dr José Antonio Peters for his technical assistance, the Conselho Nacional de Desenvolvimento Científico e Tecnológico (CNPq) for part of the financial support. This study was financed in part by the Coordenação de Aperfeiçoamento de Pessoal de Nível Superior–Brazil (CAPES) — Finance Code 001.
Disclosure Statement
The authors declare that they have no conflict of interest.
References
- Abbasi, F., A. Khadivi, M. Taghizadeh, and B. Valizadehkaji. 2019. Micropropagation of Prunus scoparia, a suitable rootstock for almond under drought conditions. Int. J. Fruit Sci. 19:221–230. doi: 10.1080/15538362.2018.1539695.
- Aghaye, R.N.M., and A. Yadollahi. 2012. Micropropagation of GF 677 Rootstock. J. Agric. Sci. 4:131–138. doi: https://doi:10.5539/jas.v4n5p131.
- Ahmad, T., N.A. Abbasi, I.A. Hafiz, and A. Ali. 2007. Comparison of sucrose and sorbitol as main carbon energy sources in micropropagation. Pak. J. Bot. 39:1269–1275.
- Alanagh, E.N., G. Garoosi, R. Haddad, S. Maleki, M. Landin, and P.P. Gallego. 2014. Design of tissue culture media for efficient Prunus rootstock micropropagation using artificial intelligence models. Plant Cell Tissue Organ Cult. 117:349–359. doi: 10.1007/s11240-014-0444-1.
- Arab, M.M., A. Yadollahi, A. Shojaeiyan, and H. Ahmadi. 2016. Artificial neural network genetic algorithm as powerful tool to predict and optimize in vitro proliferation mineral medium for G ᵡ N15 rootstock. Front. Plant Sci 7:1526.
- Arab, M.M., A. Yadollahi, A. Shojaeiyan, S. Shokri, and M. Ghojahs. 2014. Effects of nutrient media, different cytokinin types and their concentrations on in vitro multiplication of G ᵡ N15 (hybrid of almond ᵡ peach) vegetative rootstock. J. Genet. Eng. Biotechnol. 12:81–87. doi: 10.1016/j.jgeb.2014.10.001.
- Ashraf, M.F., M.A. Aziz, N. Kemat, and I. Ismaili. 2014. Effect of cytokinin types, concentrations and their interactions on in vitro shoot regeneration of Chlorophytum borivilianum Sant. & Fernandez. Electron J Biotechn. 17:275–279. doi: 10.1016/j.ejbt.2014.08.004.
- Bahmani, R., O. Karami, and M. Gholami. 2009. Influence of carbon sources and their concentrations on rooting and hyperhydricity of apple rootstock MM.106. World Appl. Sci. J 6:1513–1517.
- Baker, C.M., and H.Y. Wetzstein. 2004. Influence of auxin type and concentration on peanut somatic embryogenesis. Plant Cell Tissue Organ Cult. 36(3):361–368. doi: 10.1007/BF00046094.
- Bandeira, J.D.M., L.B. Thurow, E.J.B. Braga, J.A. Peters, and V.J. Bianchi. 2012. Rooting and acclimatization of the Japanese plum tree, cv. América. Revista Brasileira De Fruticultura 34(2):597–603. doi: 10.1590/S0100-29452012000200035.
- Cheong, E.J. 2012. Biotechnological approaches for improvement and conservation of Prunus species. Plant Biotechnol. Rep. 6:17–28. doi: 10.1007/s11816-011-0195-y.
- Cheong, E.J., and C. An. 2015. Effect of Carbohydrates on in vitro shoot growth of various Prunus species. Korean J. Plant Res. 28(3):357–362. doi: 10.7732/kjpr.2015.28.3.357.
- Dobranszki, J., and J.A.T. Silva. 2010. Micropropagation of apple — A review. Biotechnol. Adv. 28:462–488.
- Eckstein, A., P. Zięba, and H. Gabryś. 2012. Sugar and light effects on the condition of the photosynthetic apparatus of Arabidopsis thaliana cultured in vitro. J. Plant Growth Regul. 31(1):90–101. doi: 10.1007/s00344-011-9222-z.
- Esmenjaud, D., R. Voisin, C.V. Ghelder, N. Bosselut, B. Lafargue, M. Di Vito, E. Dirlewanger, J.L. Poessel, and M. Kleinhentz. 2009. Genetic dissection of resistance to root-knot nematodes Meloidogyne spp. in plum, peach, almond, and apricot from various segregating interspecific Prunus progenies. Tree Genet. Genomes 5(2):279–289. doi: 10.1007/s11295-008-0173-x.
- Felipe, A.J. 2009. ‘Felinem’, ‘Garnem’, and ‘Monegro’ Almond × Peach hybrid rootstocks. Hortscience 44(1):196–197. doi: 10.21273/HORTSCI.44.1.196.
- Fenning, T.M. 2019. The use of tissue culture and in-vitro approaches for the study of tree diseases. Plant Cell Tissue Organ Cult. 136:415–430. doi: 10.1007/s11240-018-01531-0.
- Gainza, F., I. Opazo, V. Guajardo, P. Meza, M. Ortiz, J. Pinochet, and C. Muñoz. 2015. Rootstock breeding in Prunus species: Ongoing efforts and new challenges. Chil. J. Agric. Res. 75:6–16. doi: 10.4067/S0718-58392015000300002.
- García-Gonzáles, R., K. Quiroz, B. Carrasco, and P. Caligari. 2010. Plant tissue culture: Current status, opportunities and challenges. Ciencia E Investigación Agraria 37(3):5–30. doi: 10.4067/S0718-16202010000300001.
- Gubis, J., Z. Lajchová, and L. Klčová. 2005. The effect of carbon source on plant regeneration in tomato. Hortic. Sci. 32:1–8. doi: 10.17221/3759-HORTSCI.
- Hossini, A.D., and E.G. Moghadam. 2010. Effects of media cultures and plant growth regulators in micropropagation of Gisela 6 rootstock. Ann. Biol. Res. 1:135–141.
- IBGE, 2020. Área colhida e quantidade produzida da lavoura permanente. Instituto Brasileiro de Geografia e Estatística, Rio de Janeiro.http://www.ibge.gov.br/estadosat/.
- Kadota, M., K. Imizu, and T. Hirano. 2001. Double-phase in vitro culture using sorbitol increases shoot proliferation and reduces hyperhydricity in Japanese pear. Sci. Hortic. 89:207–215.
- Kalinina, A., and D.C.W. Brown. 2007. Micropropagation of ornamental Prunus spp. and GF305 peach, a Prunus viral indicator. Plant Cell Rep. 26:927–935. doi: 10.1007/s00299-007-0315-x.
- Kanayama, Y. 2009. Physiological roles of polyols in horticultural crops. J. Jpn. Soc. Hortic. Sci. 78(2):158–168. doi: 10.2503/jjshs1.78.158.
- Kassaye, E., and B.D. Bekele. 2015. In vitro optimization of the protocol for micropropagation of plum (Prunus salicina L. Var. methley) from nodal explants. Biotechnol. Int. 8:137–148.
- Kim, H., M. Farcuha, Y. Cohenb, C. Crisostoa, A. Sadka, and E. Blumwald. 2015. Non-climacteric ripening and sorbitol homeostasis in plum fruits. Plant Sci. 231:30–39. doi: 10.1016/j.plantsci.2014.11.002.
- Lata, H., S. Chandra, N. Techen, I. Khan, and M.A. Elsohly. 2016. In vitro mass propagation of Cannabis sativa L.: A protocol refinement using novel aromatic cytokinin meta-topolin and the assessment of eco-physiological, biochemical and genetic fidelity of micropropagated plants. J. Appl. Res. Med. Aroma. 3:18–26. doi: 10.1016/j.jarmap.2015.12.001.
- Mansseri-Lamrioui, A., A. Louerguioui, J. Bonaly, S. Yakoub-Bougdal, N. Allili, and S. Gana-Kebbouche. 2011. Proliferation and rooting of wild cherry: The influence of cytokinin and auxin types and their concentration. Afr. J. Biotechnol. 10:8613–8624. doi: http://dx.doi.10.5897/AJB11.450.
- Nacheva, L., and P. Gercheva. 2009. Micropropagation of Gisela 5 (cherry dwarf rootstock): The effect of the type and the concentration of the carbohydrates in the nutrient medium. Acta Hortic. 825(825):261–268. doi: 10.17660/ActaHortic.2009.825.41.
- Naik, P.M., S.H. Manohar, N. Praveen, and H.N. Murthy. 2010. Effects of sucrose and pH levels on in vitro shoot regeneration from leaf explants of Bacopa monnieri and accumulation of bacoside A in regenerated shoots. Plant Cell Tissue Organ Cult (PCTOC). 100:235–239.
- Nasri, A., E. Baklouti, A.B. Romdhane, M. Maalej, H.M. Schumacher, N. Drira, and L. Fki. 2019. Large-scale propagation of Myrobolan (Prunus cerasifera) in RITA® bioreactors and ISSR-based assessment of genetic conformity. Sci. Hortic.. 245:144–153. doi: 10.1016/j.scienta.2018.10.016.
- Nowak, B.K., K. Miczynski, and L. Hudy. 2004. Sugar uptake and utilisation during adventitious bud differentiation on in vitro leaf explants of ‘Wegierka Zwykła’ plum (Prunus domestica). Plant Cell Tissue Organ Cult. 76(3):255–260. doi: 10.1023/B:TICU.0000009247.94819.02.
- Oldoni, H., V.S.S. Terra, L.C. Timm, C.R. Júnior, and A.B. Monteiro. 2019. Delineation of management zones in a peach orchard using multivariate and geostatistical analyses. Soil Till Res. 191:1–10. doi: 10.1016/j.still.2019.03.008.
- Ozudogru, E.A., C. Benelli, G. Dradi, and M. Lambardi. 2017. Effect of culture container and carbohydrate content on in vitro slow growth storage of the cherry rootstock ‘Gisela®5’. Acta Physiol. Plant. 39(4):1–8. doi: 10.1007/s11738-017-2372-2.
- Pahnekolayi, M.D., A. Tehranifar, L. Samiei, and M. Shoor. 2019. Optimizing culture medium ingredients and micrografting devices can promote in vitro micrografting of cut roses on different rootstocks. Plant Cell Tissue Organ Cult. (PCTOC) 137(2):265–274. doi: 10.1007/s11240-019-01567-w.
- Park, J.S., A.H. Naing, and C.K. Kim. 2016. Effects of ethylene on shoot initiation, leaf yellowing, and shoot tip necrosis in roses. Plant Cell Tissue Organ Cult. (PCTOC) 127(2):425–431. doi: 10.1007/s11240-016-1066-6.
- Pathak, H., and V. Dhawan. 2012. Influence of different carbohydrate sources on in vitro shoot proliferation of apple (Malus × domestica Borkh.) rootstocks M 7 AND MM 111. Acta Hortic. 961(961):311–317. doi: 10.17660/ActaHortic.2012.961.41.
- Pérez-Jimenez, M., A. Carrillo-Navarro, and J. Cos-Terrer. 2012. Regeneration of peach (Prunus persica L. Batsch) cultivars and Prunus persica × Prunus dulcis rootstocks via organogenesis. Plant Cell Tissue Organ Cult. (PCTOC) 108(1):55–62. doi: 10.1007/s11240-011-0011-y.
- Phillips, G.C., and M. Garda. 2019. Plant tissue culture media and practices: An overview. In Vitro Cell. Dev. Biol. - Plant 55(3):242–257. doi: 10.1007/s11627-019-09983-5.
- Pruski, K.W., T. Astatkie, and J. Nowak. 2005. Tissue culture propagation of Mongolian cherry (Prunus fruticosa) and Nanking cherry (Prunus tomentosa). Plant Cell, Tissue and Organ Culture 82(2):207–211. doi: 10.1007/s11240-004-7836-6.
- Quoirin, M., and P. Lepoivre. 1977. Improved media for in vitro culture of Prunus sp. Acta Hortic. 78(78):437–442. doi: 10.17660/ActaHortic.1977.78.54.
- Radmann, E.B., C.M. Gallo, C.W. Ritterbusch, V.J. Bianchi, J.A. Fernando, and J.A. Peters. 2014. In vitro rooting and acclimatization of ‘Mr. S. 2/5’ plum rootstock. Plant Cell & Micropropagation 10:21–31.
- Radmann, E.B., V.J. Bianchi, J.C. Fachinello, L.V. Ferreira, and R.P. Oliveira. 2011. In vitro multiplication of ‘Flordaguard’ rootstock: Cytokinin source and concentration effects, explants orientation and period of permanence in the cultive medium. Braz. Arch. Biol. Technol. 54:25–34. doi: http://dx.doi.10.1590/S1516-89132011000100004.
- Radmann, E.B., V.J. Bianchi, R.P. Oliveira, and J.C. Fachinello. 2009a. Multiplicação in vitro e alongamento das brotações micropropagadas do porta-enxerto ‘Tsukuba 1’ (Prunus pérsica L.). Rev. Bras. Frutic. 31:656–663. doi: http://dx.doi.10.1590/S0100-29452009000300006.
- Radmann, E.B., V.J. Bianchi, T.J. Souza, J.C. Fachinello, and R.P. Oliveira. 2009b. Influence of culture medium composition and explant type on micropropagation of the rootstock Prunus sp. ‘G ᵡ N-9’. Sci. Agric. 10:95–101.
- Rocha, P.S., M.W. Schuch, V.J. Bianchi, and J.C. Fachinello. 2009. In vitro multiplication and shoot elongation of rootstock of Prunus. Biosci. J. 25:69–74.
- Rodrigues, A.C., C.A.P. Silveira, G.R. Fortes, J.C. Fachinello, and J.B. Silva. 2003. Estabelecimento e multiplicação in vitro de Prunus sp. em diferentes meios de cultura. Rev. Bras. Frutic 25:131–133.
- Rogalski, M., L.K.A. Moraes, R.C. Felisbino, L. Crestani, M.P. Guerra, and A.L. Silva. 2003a. Enraizamento in vitro de porta-enxertos de Prunus sp. Rev. Bras. Frutic. 25:293–296. doi: http://dx.doi.10.1590/S0100-29452003000200028.
- Rogalski, M., L.K.A. Moraes, R.C. Felisbino, L. Crestani, M.P. Guerra, and A.L. Silva. 2003b. Aclimatização de porta-enxertos de Prunus sp. Micropropagados. Rev. Bras. Frutic. 25:279–281.
- Rossi, C.E., L.C.C.B. Ferraz, and P.T. Montaldi. 2002. Resistência de frutíferas de clima subtropical e temperado a Meloidogyne incognita raça 2 e Meloidogyne javanica. Arq. Inst. Biol. 69:43–49.
- Ruzic, D.V., and T.I. Vujovic. 2008. The effects of cytokinin types and their concentration on in vitro multiplication of sweet cherry cv. Hortic. Sci. 35:12–21. doi: 10.17221/646-HORTSCI.
- Ruzic, V.D., T.I. Lazic, and R.M. Cerovic. 2008. Micropropagation of some Prunus and Pyrus genotypes in vitro as affected by different carbon sources. Acta Hortic. 795:413–418. doi: http://dx.doi.10.17660/ActaHortic.2008.795.62.
- Sadeghi, F., A. Yadollahi, M. Kermani, and M. Eftekhar. 2015. Optimizing culture media for in vitroproliferation and rooting of Tetra (Prunus empyrean) rootstock. J. Genet. Eng. Biotechnol. 13:19–23. doi: 10.1016/j.jgeb.2014.12.006.
- Saha, S., H. Mori, and K. Hattor. 2007. Synergistic effect of kinenin and benzyl adenine plays a vital role in high frequency regeneration from cotyledon explants of bottle gourd (Lagenaria siceraria) in relation to ethylene production. Breed. Sci. 57:197–202. doi: 10.1016/j.ejbt.2014.08.004.
- Saha, S., H. Mori, and K. Hattor. 2007. Synergistic effect of kinenin and benzyl adenine plays a vital role in high frequency regeneration from cotyledon explants of bottle gourd (Lagenaria siceraria) in relation to ethylene production. Breed. Sci. 57:197–202. doi: 10.1016/j.ejbt.2014.08.004.
- Silveira, C.A.P., J.C. Fachinello, G.R.L. Fortes, I. Citadin, A.C. Rodrigues, A.C. Quezada, and J.B. Silva. 2001. Multiplicação in vitro de porta-enxertos do gênero Prunus sob diferentes concentrações de BAP em dois meios de cultura. Rev. Bras. Frutic. 23:488–492. http://dx.doi.org/10.1590/S0100-29452001000300007.
- Siwach, P., A.R. Gill, and K. Kumari. 2011. Effect of season, explants, growth regulators and sugar level on induction and long term maintenance of callus cultures of Ficus religiosa L. Afr. J. Biotechnol. 10:4879–4886. doi: http://dx.doi.10.5897/AJB10.2119.
- Srivastava, A., and A.G. Joshi. 2009. In vitro behavior of nodal explants of Portulaca grandiflora under the influence of cytokinins. Acta Univ. Latviensis 753:43–48.
- Surakshitha, N.C., K. Soorianathasundaram, M. Ganga, and M. Raveendran. 2019. Alleviating shoot tip necrosis during in vitro propagation of grape cv. Red Globe. Sci. Hortic. 248:118–125. doi: 10.1016/j.scienta.2019.01.013.
- Teixeira, P.T., A.L. Silva, J.P.H.J. Ducroquet, and M.P. Guerra. 2004. Multiplicação in vitro do porta-enxerto de Prunus spp. ‘Carelli’. Rev. Bras. Frutic. 26:377–379. doi: http://dx.doi.10.1590/S0100-29452004000200050.
- Vujovic, T., D. Ruzic, and R. Cerovic. 2012. In vitro shoot multiplication as influenced by repeated subculturing of shoots of contemporary fruit rootstocks. Hortic. Sci. 39:101–107. doi: 10.17221/208/2011-HORTSCI.
- Wagner, J.A., M. Couto, and A.C. Quezada. 2003. Multiplicação in vitro do porta-enxerto de ameixeira Julior. Revista Brasileira De Agrociência. 9:121–124. doi: http://dx.doi.10.18539/CAST.V9I2.587.
- Wolella, E.K. 2017. Surface sterilization and in vitro propagation of Prunus domestica L. cv. Stanley using axillary buds as explants. J. Biotech Res. 8(1) 18–26.
- Yaseen, M., T. Ahmad, A.N. Abbasi, and I.F. Hafiz. 2009. In vitro shoot proliferation competence of apple rootstocks M. 9 and M. 26 on different carbon sources. Pak. J. Bot 41:1781–1795.
- Yaseen, M., T. Ahmad, G. Sablok, A. Standardi, and I.F. Hafiz. 2012. Review: Role of carbon sources for in vitro plant growth and development. Mol. Biol. Rep. 40:2837–2849. doi: http://dx.doi.10.1007/s11033-012-2299-z.
- Zhang, W.B., X.C. Piao, J.R. Li, Y.H. Jin, and M.L. Lian. 2017. Optimized culture medium for the production of flavonoids from Orostachys cartilaginea V.N. Boriss. callus cultures. In Vitro Cell Dev. Biol. Plant. 53:1–11. doi: 10.1007/s11627-017-9799-4.
- Zhou, H., M. Li, X. Zhao, X. Fan, and A. Guo. 2010. Plant regeneration from in vitro leaves of the peach rootstock ‘Nemaguard’ (Prunus persica x P. davidiana). Plant Cell Tissue Organ Cult. 101:79–87. doi: 10.1007/s11240-010-9666-z.