ABSTRACT
Two related underutilized plants from breadfruit (Artocarpus altilis) and breadnut (Artocarpus camansi) were explored for their antioxidant and toxicity potential. Maceration with water was employed for the sake of safety and easiness. Various parts of both plants, namely leaves, fruit peel, anthocarp, and breadnut achene, were investigated. The antioxidant assays employed in this study were total phenolic and flavonoid content, total antioxidant capacity, DPPH scavenging activity, power-reducing assay, and linoleic acid peroxidation inhibition. The acute toxicity potential of the extracts was assessed using inhibition of Vibrio harveyi bioluminescence. The EC50 value determined from the toxicity assessment (>2.4 mg/mL) was higher than the concentrations of all extracts used in the various assays of antioxidant property. From all aqueous extracts, the best results in terms of highest antioxidant activity and relatively lowest toxicity were obtained for breadnut leaves, breadnut achene, and breadfruit leaves.
Introduction
Reactive oxygen species (ROS) are unhindered by-products of aerobic metabolism. Overproduction of ROS contributes to oxidative stress which damages lipids, proteins, and nucleic acids (Mitler, Citation2017). It was found that moderate excess of ROS resulted in tumorigenesis while high level of ROS induced various chronic diseases due to physiological aging and apoptosis (Davali et al., Citation2016). Antioxidants are substances that can scavenge the free radicals that result from the retardation of the oxidation processes. Many antioxidant compounds can be found naturally in plants. Their discovery and exploration promoted the interest in research to develop cures for age-related diseases (Zhang et al., Citation2015). Among various plant secondary metabolites, phenolic and flavonoid groups are abundant in almost all plant materials and believed to exhibit good antioxidant activity (Gulcin et al., Citation2010; Jagtap et al., Citation2010; Shahidi and Ambigaipalan, Citation2015).
Among plant sources, Artocarpus genus is known to possess phenolic, flavonoid, and xanthone compounds. Some novel prenylated flavonoids and xanthones have been found in Artocarpus plants (Hashim et al., Citation2010; Jayasinghe et al., Citation2008; Makmur et al., Citation2000; Sidahmed et al., Citation2013).
Breadnut (Artocarpus camansi Blanco) is the wild ancestor of the breadfruit (Artocarpus altilis [Parkinson] Fosberg) (Luzuriaga-Quichimbo et al., Citation2018). These two plants that originated in Papua and Maluku islands were domesticated to serve as traditional staple plants in areas of the Pacific, South East Asia, and South America (Hakim et al., Citation2006; Luzuriaga-Quichimbo et al., Citation2019; Zerega et al., Citation2015). The main difference between these two plants is that the breadnut fruit has a soft spine on the surface and breadfruit does not contain achene (usually referred to as seedless) due to abortion while breadnut possesses numerous achene (usually called seeds) (Zerega et al., Citation2005). The breadnut seeds are edible. They are composed of 4.87% protein, 3.48% fat, 1.2% fiber, 3.43% ash, 60.96% water, and 26.11% carbohydrate. The fat is also rich in unsaturated fatty acids (Adeleke and Abiodun, Citation2010). Breadfruit anthocarp (commonly referred as fruit flesh) has an approximate composition of 69% water, 1% protein, and 29% dietary fiber, resulting in about 121 Cal per 100 g. Breadfruit also contains vitamins A, B, and C (Ragone and Cavaletto, Citation2006). The breadfruit fruit yields a good quality protein due to its essential amino acid content (Liu et al., Citation2015). Overall, various intrinsic (genetics, cultivars, etc.) and extrinsic factors such as microclimate and other environmental factors contribute to the difference in composition (Jagtap et al., Citation2010). Ethnopharmacologically, breadnut and the fruit of breadfruit are known to treat liver diseases, while the leaves can be used to heal cirrhosis, diabetes, and hypertension (Jagtap and Bapat, Citation2010).
The currently low use of these two plants is due to urbanization of agricultural land, although these plants have been recognized for their importance for food security (Jones et al., Citation2013; Nnam and Nwokocha, Citation2003). No report have been found that compares the secondary metabolite profiles from both plants, and only very few reports can be found that deal with the antioxidant potential of these plants (Jalal et al., Citation2015; Salleh and Jinis, Citation2016; Vianney et al., Citation2018). The purpose of this study was to investigate the antioxidant potential from breadnut and breadfruit employing water as solvent and maceration as an extraction technique. Comparison of phenolic content and antioxidant activity of extracts from each tested organs are also discussed. Various methods were used to assess the antioxidant properties of the products. Acute toxicity of these plant parts was also studied to verify the safety of these extracts as functional herbal foods. The ease of the extraction technique and the GRAS properties of water add to the application potential of the described study.
Materials and Methods
Plant Materials
Breadnut and breadfruit leaves and fruits (Supp. 1) were collected in Surabaya City, East Java, Indonesia. Breadnut (voucher specimen No. 1223/D.T/II/2017) and breadfruit (voucher specimen No. 1343/D.T/VIII/2018) have been identified and deposited at the Center of Information and Development of Traditional Medicine, Faculty of Pharmacy, University of Surabaya.
Chemicals
All chemicals were of analytical grade. Sodium hydroxide (NaOH), aluminum(III) chloride (AlCl3), sodium nitrite (NaNO2), Folin-Ciocalteu’s reagent, sodium dihydrogen phosphate (NaH2PO4), disodium hydrogen phosphate (Na2HPO4), potassium ferricyanide (K3Fe(CN)6), trichloroacetic acid, iron(III) chloride (FeCl3), concentrated sulfuric acid (H2SO4), ammonium molybdate, potassium thiocyanate (KSCN), hydrochloric acid (HCl), iron(II) chloride (FeCl2), zinc(II) sulfate (ZnSO4), sodium chloride (NaCl), tryptic soy agar, and tryptic soy broth were obtained from Merck (Darmstadt, Germany). Catechin and 1,1-diphenyl-2-picrylhydrazil (DPPH) were acquired from Sigma-Aldrich (Steinheim, Germany). Sodium carbonate anhydrous was from Mallinckrodt Baker (Kentucky, United States). Gallic acid was from MP (Ohio, United States). Ascorbic acid was obtained from Amresco (Ohio, United States). Absolute ethanol was from J.T. Baker (Kentucky, United States) and linoleic acid was purchased from Tokyo Chemical Industry (Tokyo, Japan). Vibrio harveyi culture for the toxicity assay was obtained from the culture collection of Faculty of Biotechnology, University of Surabaya.
Maceration of Breadnut and Breadfruit Samples
Breadnut leaves (500 g), breadfruit leaves (200 g), breadnut fruit peel (720 g), breadfruit fruit peel (260 g), breadnut fruit flesh (1200 g), breadfruit fruit flesh (1536 g), and breadnut seed (1000 g) were washed with tap water. Fruit samples were taken from the ripened one. Leaves and fruit peel were wiped with 70% ethanol. Leaves and fruit peel were torn while fruit flesh and seeds were cut and then dried for two days at 40°C. All dried materials were ground to powder and filtered through 70-mesh filter, yielding 141 g of dry breadnut leaves, 72 g of dry breadfruit leaves, 120 g of dry breadnut fruit peel, 58 g of dry breadfruit fruit peel, 190 g of breadnut fruit flesh, 346 g of breadfruit fruit flesh, and 115 g of breadnut seed.
Leaf powders (18 g) were macerated in distilled water and stirred (270 mL) for 3 days at room temperature, while fruit peel, flesh, and seed powder were macerated with stirring for 2 days. The optimization of maceration time can be found in Supp. 2. For the optimization process two parameters were tested, namely total phenolic content (TPC) and total flavonoid content (TFC) using methods described in 2.4 and 2.5, in which aliquots of 0.5 mL (unknown concentration) of the macerated extracts were sampled daily (n = 3). Aqueous macerates were dried under reduced pressure at 50°C; drying was continued by oven drying until it reached constant mass. Dry extracts were resuspended in distilled water to a final concentration of 100 mg mL−1. Samples were stored in 4°C and kept in the dark.
Total Phenolic Content (TPC) Analysis
The protocol followed Folin-Ciocalteu assay (Loizzo et al., Citation2010). An aliquot of 0.5 mL of various aqueous extracts (1 mg mL−1), each mixed with 0.2 mL Folin-Ciocalteu’s reagent, 2 mL of distilled water, and 1 mL of sodium carbonate anhydrous solution (15%) was incubated for 1 h at room temperature in the dark. The absorbance of the mixture was measured at 765 nm. The standard curve was made using gallic acid as standard compound in the concentration range of 10–100 mg L−1 with r2 = 0.9995. The results were expressed as mg of gallic acid equivalent/100 g dry weight (mg GAE 100 g−1 DW).
Total Flavonoid Content (TFC) Analysis
The protocol followed the complexation of AlCl3 under basic conditions. To each 0.5 mL of various aqueous extracts (1 mg mL−1), 0.15 mL of NaNO2 solution (150 g L−1) and 0.15 mL of AlCl3 solution (100 g L−1) were added. The mixture was incubated at room temperature for 6 min. 2 mL of 1 M NaOH and 2.2 mL distilled water were added and the mixture was incubated for 15 min. The absorbance of the solution was measured at λ = 510 nm. Catechin was used to prepare the standard curve in the concentration range of 10–90 mg L−1 with r2 = 0.995. The parameter was determined as mg catechin equivalent/100 g dry weight (mg CE 100 g−1 DW).
Total Antioxidant Capacity (TAC) Assay
The method was adopted from that reported in Ahmed et al. (Citation2015). 0.3 mL of extract (1 mg mL−1) was mixed with 3 mL of phosphomolybdate reagent (0.6 M H2SO4 + 28 mM Na3PO4 + 4 mM ammonium molybdate). The mixture was incubated in the dark at 95°C for 90 min. After cooling down, the solution was measured at 765 nm to obtain the antioxidant capacity with the unit of mg ascorbic acid equivalent/100 g dry weight (mg AAE 100 g−1 DW). The concentration range of ascorbic acid was 25–250 mg L−1 and the r2 value for the standard curve was 0.9968.
DPPH Scavenging Activity
The DPPH scavenging activity method was adopted from Li et al. (Citation2012). The DPPH stock solution was dissolved in methanol (100 mg L−1). 50 µL of DPPH stock solution was added to 200 µL of various aqueous extracts at serial dilution concentrations of 1000, 500, 250, 125, 100, 50, 25, and 12.5 mg L−1 in a 96-well microplate. The microplate was agitated at 700 rpm for 30 min in the dark and the absorbance was measured at 517 nm. The percentage of DPPH radical scavenging activity was determined with the following equation (Eq.1):
% of DPPH scavenging activity = (A0 – A1) x 100/A0 (Eq. 1)
Where A0 is the absorbance of the control with only solvent added and A1 is the sample absorbance. The parameter used to compare the radical scavenging activity between extracts was IC50, which was the concentration of extracts that could reduce 50% of DPPH color absorbance. Ascorbic acid was used as a positive control in the concentration range of 2 to 20 mg L−1.
Reducing Power Assay
The reducing power of extracts was measured by using the formation of Prussian Blue color from the reaction of Fe3+ and K4Fe(CN)6 (Adjimani and Asare, Citation2015). The working solution was 2.5 mL of plant aqueous extracts prepared in various concentrations (100, 200, 300, 500, 1000, 2000, 2500, 5000, and 10000 mg L−1, respectively), mixed with 2.5 mL of 0.2 M sodium phosphate buffer (pH 6.6), and 2.5 mL 1% K3Fe(CN)6. The mixture was incubated at 50°C for 20 min. To the mixture, 2.5 mL of 10% trichloroacetic acid were added and the mixture was centrifuged at 1000 rpm for 10 min. 5 mL of supernatant was transferred into a separate tube and 5 mL distilled water and 1 mL of 0.1% FeCl3 were added. The absorbance of the mixture was measured at 700 nm. Higher values of absorbance indicated enhanced reducing power. EC50 was used to compare the reducing power of extracts. It is defined as the concentration of extract obtained by intrapolation of concentration values that resulted in 0.5 units of absorbance (Fernandez-Agullo et al., Citation2013).
Lipid Peroxidation Inhibitory Assay
The method was adopted from the method described by Loganayaki et al. (Citation2013) with modification. The working solution was obtained by mixing 1 mg of plant aqueous extracts (0.1 mL of extract with the concentration of 10 mg mL−1) with 0.5 mL of 2.51% linoleic acid in absolute ethanol, and 1 mL of 0.2 M phosphate buffer (pH 7). The volume was adjusted with distilled water to 2 mL. The mixture was incubated for 25 min at 40°C to activate the oxidation process. It was kept at room temperature in the dark. Every 24 h for 4 days, an aliquot of 0.1 mL was mixed with 9.7 mL 75% ethanol, and 0.1 mL 30% KSCN and the mix agitated for 3 min. Then, 0.1 mL FeCl2 (0.02 M in 3.5% HCl) was added to the mixture and the mix incubated at room temperature for 10 min, during which the absorbance reached a maximum and stable reading. 1 mg of vitamin A supplement (retinol acetate) was used as a positive control. The negative control was made without the addition of plant extract. Both controls were incubated along with the samples. A blank sample was made by exchanging the working solution with distilled water and treating the mixture in the same way as described above. The absorbance was measured at 500 nm. The antioxidant activity (AA) was determined as shown in Eq. 2:
AA (%) = (1- (Sample absorbance after 96 h/negative control absorbance after 96 h)) x 100 (Eq. 2)
Determination of Acute Toxicity Using V. Harveyi Bioluminescence Inhibition
V. harveyi was grown in TSB + 2% NaCl at 28°C. The method was adopted from Westlund et al. (Westlund et al., Citation2018) with modification. The V. harveyi suspension after 20 h incubation (100 µL with OD600 ~ 1–1.3) was mixed with 100 µL of plant extracts with final concentrations of 10000, 5000, 2500, 1000, 500, 250, and 100 mg L−1, respectively, and diluted with NaCl solution so as to create approximately equal concentrations of NaCl in the sample well as in the growing medium of V. harveyi (2%). V. harveyi light emission was detected after 15 and 30 min incubation in dark, respectively, as suggested by Microtox®. The working solution was microplated in the dark and the microplate was incubated and read with a microplate reader with a lens without wavelength filter (Fluostar Omega BMG Labtech). Toxicity power was measured as shown in Eq. 3
Toxicity power (%) = (A0 – A1) x 100/A0 (Eq.3)
Where A0 is the absorbance of the blank solution (2% NaCl) and the A1 the sample absorbance. EC50 was defined as the concentration of plant extracts that could reduce 50% light absorbance which indicated 50% inhibition of bioluminescence. ZnSO4 in the concentration range of 0 to 200 mg L−1 was used as positive control while 2% NaCl solution was used as negative control. Both controls were incubated along with the sample. OD600 value and lens sensitivity (4095) were adjusted as to place the luminescence value of 100 µL cell suspension +100 µL 2% NaCl within the range of 15000–25000.
Statistical Analysis
All experiments were performed in triplicate. Results were expressed in average ±S.D. and analyzed with the help of SPSS 20 (IBM Corporation, New York, United States). Shapiro-Wilk normality test and Levene variance homogeneity test (P < .05) were employed to analyze the distribution of the sample. The data of the normally distributed assays were compared with one-way ANOVA and the significance of the differences was analyzed with Duncan’s Multiple Range Test with the significant value being P < .01. The data that did not exhibit a normal distribution or did not pass the Levine homogeneity or Shapiro-Wilk tests were evaluated with the Kruskal–Wallis test followed by multiple comparison Dunn–Bonferroni test with the significant value of P < .05.
The IC50 from DPPH and the EC50 of the acute toxicity test were obtained by plotting the non-linear curve between log(concentration) and normalized response with 1000 iteration points with the help of GraphPad Prism 6 (GraphPad Software, Inc., California, United States). Significant variance test was employed with one-way ANOVA followed by Tukey’s Honest Significant Difference with a significant level of P < .001.
Results and Discussion
Total Phenolic and Flavonoid Content
Phenolic and flavonoid content are important parameters that are frequently correlated with antioxidant activity, especially due to the reducing activity of the hydroxyl groups (Mierziak et al., Citation2014; Sahreen et al., Citation2010). The amount of extracted phenolic and flavonoid compounds depends on the polarity of the solvent. Polar solvents are preferable for extracting phenolic and flavonoid compounds (Jagtap et al., Citation2010; Roby et al., Citation2013). Various methods have been employed to extract phenolic compounds from plant materials, ranging from conventional methods such as maceration, or modern methods such as ultrasonic or microwave-assisted extraction (Khoddami et al., Citation2013). Maceration was chosen due to the simplicity of the apparatus needed for extraction and application. It was reported that maceration of chokeberry at ambient temperature yielded a higher phenolic content compared to that of ultrasonic-assisted extraction (Cujic et al., Citation2016; Khoddami et al., Citation2013).
Statistical analysis clearly showed that breadnut leaf gave the highest TPC and TFC when macerated for 3 days, while breadnut and breadfruit fruit peel, breadfruit fruit flesh, and breadnut seed were macerated for 2 days. For breadfruit leaf, although TPC peaked on 2nd day of extraction, TFC was extracted on 3rd day of extraction for the best result. Higher flavonoid content was associated with better antioxidant activity due to the fact that flavonoids contain more hydroxyl groups compared to simple phenolic compounds which are readily available to radicals (Mierziak et al., Citation2014). Meanwhile, TPC and TFC data for breadnut fruit flesh did not differ significantly between 2 days and 3 days of extraction. Considering the time efficiency, the optimal extraction time was chosen as 2 days. The duration of maceration was comparable to other study (Santos et al., Citation2018).
The extraction yield, total phenolic (TPC) and total flavonoid content (TFC) values are shown in . The TPC ranking for the various aqueous extracts was breadnut seed > breadnut leaf > breadnut fruit flesh > breadfruit fruit peel > breadfruit leaf > breadfruit fruit peel > breadnut fruit flesh. Specifically, with a P < .01, breadnut seed displayed a TPC of 528.193 mg GAE 100 g−1 DW, followed by breadnut leaf (496.115 mg GAE 100 g−1 DW). The order of the TFC from various aqueous extracts was breadnut seed > breadfruit leaf > breadnut leaf > breadnut fruit flesh > breadfruit fruit peel > breadnut fruit peel > breadfruit fruit flesh. The results of TPC from all tested parts of A. altilis and A. camansi were higher than those of the aqueous extracts of A. heterophyllus fruit pulp (25 mg GAE 100 g−1 DW for TPC); however, the TFC might not be comparable due to the different standards used in this study (Jagtap et al., Citation2010). Similar values of TPC (280–1220 mg GAE 100 g−1 DW) were reported in herbal infusions of six Argentine Patagonia, but the TPC values of tested A. altilis and A. camansi were lower than commercial green tea extracted by boiling water (Gastaldi et al., Citation2018).
Table 1. Dry yield, extraction yield, total phenolic, and flavonoid content from the various parts of breadnut and breadfruit aqueous extracts
High level of phenolic and flavonoid content in leaves compared to the fruit peel and flesh, which are the evolution from the flower accessory organ, can be rationalized due to the importance of leaves itself as a source organ. Other than antioxidant purpose, phenolic and flavonoid compounds are often used to scavenge free radical from the by-product of photosynthesis and also to discourage herbivory (Tuominen, Citation2013; Tuominen et al., Citation2013). On the other hand, seed is an important sink organ in the generative period of plant life cycle. High phenolic acid contents can also be found over various species (Hendry et al., Citation1994). Phenolic polymers such as lignin can be used as a physical barrier which contributes to the hardiness of the seeds, to resist drought and various biotic stresses, and to increase persistence to various soil conditions (Hendry et al., Citation1994; Tuominen et al., Citation2013).
Determination of Antioxidant Activity
Total antioxidant capacity can be determined with the help of phosphomolybdate reagent. This assay is based on the reduction of molybdenum (VI) to molybdenum (V) in the presence of antioxidant or reducing agent (Ahmed et al., Citation2015). As shown in , breadnut leaf clearly showed the highest antioxidant capacity, followed by breadfruit leaf and breadnut seed (P < .01). These three parts also contained a high content of phenolic and flavonoid compounds compared to the other aqueous extracts.
Figure 1. Total antioxidant capacity of the aqueous extracts from various parts of breadnut and breadfruit. Different letters are significantly different with Duncan’s Multiple Range Test at P < .01
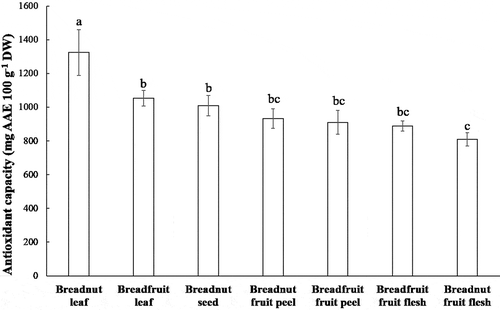
One generally used antioxidant assay is the scavenging of the proton radical using DPPH (Bakar et al., Citation2009; Hannachi et al., Citation2019). DPPH is a stable radical with purple color. A reduction reaction, for instance by the hydroxy groups from the phenolic and flavonoid compounds could stoichiometrically reduce every DPPH molecule, causing the discoloration of DPPH which can be spectrophotometrically quantified (Sahreen et al., Citation2010). Thus, the reduction of 50% of absorbance can be defined as the reduction of 50% of DPPH molecules in the solution. The IC50 values of the aqueous extracts from the various parts of breadnut and breadfruit ranged from 80 to 346 mg L−1 (). Based on the DPPH assay IC50 category of strong (50–100 mg L−1), moderate (101–150 mg L−1), and weak (>150 mg L−1) antioxidants (Fidrianny et al., Citation2018), especially the aqueous extracts of breadnut seed and breadnut leaf displayed strong radical scavenging activity, while the breadfruit leaf, breadnut fruit peel, and breadfruit fruit peel were considered to have moderate radical scavenging activity. This result was comparable to the Artocarpus heterophyllus leaves aqueous extracts where IC50 values were 73.5 mg L−1 (Loizzo et al., Citation2010) while the methanolic extracts of A. heterophyllus fruit pulp were in the range of 400–700 mg L−1 (Jagtap et al., Citation2010). The result was also comparable with the DPPH scavenging activity of six Ficus species fruits by Tamuly et al. (Citation2015). Kasangana et al. (Citation2015) found that the IC50 value of aqueous extract of Myrianthus arboreus root bark was 96.11 mg/L.
Figure 2. IC50 values of DPPH radical scavenging activity from the aqueous extracts of various parts of breadnut and breadfruit. Different letters indicate significant differences with Tukey’s Honest Significant Difference (P < .001)
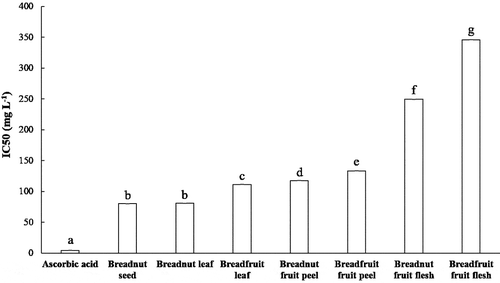
Reducing power determination is based on the ability to reduce the ferricyanide complex (Fe3+) to the Fe2+ ion (Fernández-Agulló et al., Citation2013). The concentration-dependent curve of the ferric reducing power data from various aqueous extracts, related to the absorbance at 700 nm (A700), can be found in Supp. 3. Here, higher A700 values indicate better reducing ability. This curve was used to determine the EC50 values by intrapolation. Low EC50 values imply good reducing ability and antioxidant activity. The EC50 values of the aqueous extracts ranged between 800 and 1600 mg L−1 (). This result was comparable with the EC50 values of walnut (Juglans regia L.) green husk extracts with the combination of water, methanol, and ethanol as solvents, which were in the range of 950–2160 mg L−1 (Fernández-Agulló et al., Citation2013). Clearly (with P < .01), the lowest EC50 was obtained for the breadnut seed, followed by breadnut leaf and breadnut fruit peel. The highest EC50 or weakest reducing power was determined for breadnut fruit flesh, for which a similar trend in DPPH radical scavenging activity was found. The correlation between reducing power EC50 and IC50 values of DPPH radical scavenging activity was in agreement with data reported by Fernandez-Agulló et al. (Citation2013).
Figure 3. EC50 values of reducing power from the aqueous extracts of various parts of breadnut and breadfruit. Different letters indicate significant differences with Duncan’s Multiple Range Test (P < .01)
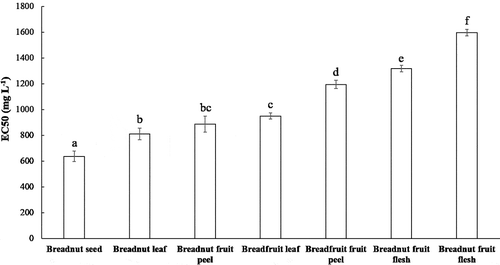
Pearson correlation analysis revealed a strong negative correlation between the IC50 values of DPPH radical scavenging activity and TPC (r = −0.758; p-value = 0.049) and TFC (r = −0.746; p-value = 0.054). Similarly, there was also a strong negative correlation between the EC50 values of reducing power and total phenolic (r = −0.819; p-value = 0.024) and total flavonoid content (r = −0.829; p-value = 0.021). Nonetheless, both phenolic and flavonoid content strongly contributed to the antioxidant activity as expected.
Linoleic acid peroxidation inhibition is an antioxidant assay that utilizes the biomolecule as its substrate. Polyconjugated fatty acids such as linoleic acid are easily oxidized which could propagate the free radical oxidative reaction (Mushtaq et al., Citation2015). The oxidation reaction can be detected with the help of the Fe2+ ion which is oxidized to Fe3+ ion and then complexed with SCN− ion to yield a blood-red color. The higher intensity of the blood-red color indicates the intensity with which the oxidation reaction occurred (Ahmed et al., Citation2015). Amounts as high as 1 mg of sample material were used in this study. Some aqueous extracts began to lose their peroxidation inhibition ability and the absorbance of the red color (500 nm) started to increase during the second day of the incubation. These samples were breadfruit fruit peel, breadfruit fruit flesh, and breadnut fruit peel, followed by breadnut fruit flesh and breadnut seed to a smaller degree. In opposition, the aqueous extracts of breadnut and breadfruit leaf as well as vitamin A as the positive control could retain the linoleic acid peroxidation to a low level (Supp. 4).
By comparing the inhibition of the linoleic acid peroxidation after 96 h incubation at ambient temperature and in the dark (), the order from the strongest to the weakest inhibition was: retinol acetate (+ control) > breadfruit leaf > breadnut leaf > breadnut fruit flesh > breadnut seed > breadnut fruit peel > breadfruit fruit flesh > breadfruit fruit peel. The inhibition of the peroxidation reaction of the aqueous extracts of breadfruit and breadnut leaf did not differ significantly (P < .01) from the positive control. The linoleic acid peroxidation inhibition was also caused by the hydrogen donating ability of the phenolic compound and its derivative (Loganayaki et al., Citation2013). Likewise, the methanolic and hexane extract of Adiantum caudatum leaf (0.5 mg of extracts, respectively) that was rich in phenolic and flavonoid content could inhibit the linoleic peroxidation up to 48–72 h (Ahmed et al., Citation2015), while Helicteres isora fruit and Ceiba pentandra seed extracts (1 mg of extracts, respectively) could inhibit the linoleic peroxidation for 60 h (Loganayaki et al., Citation2013).
Figure 4. Percentage of the inhibition of linoleic acid peroxidation from the aqueous extracts of various parts of breadnut and breadfruit after 96 h incubation. Different letters indicate significant differences with Duncan’s Multiple Range Test (P < .01)
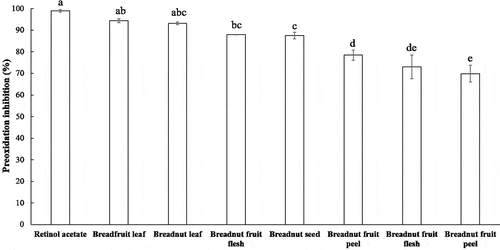
Artocarpus genus is well known for its unique flavonoid constituents derived from chalcone. Most of the flavonoid compounds are prenylated (Jagtap and Bapat, Citation2010). Unique compounds from A. altilis which has been reported is artoindonesianin F, along with other compounds which can be found in other species such as artonol, artocarpin, cycloartocarpin, artoindonesianin B, and also morusin, a well-known antioxidant compound from the Moraceae family (Hakim et al., Citation2006). However, antioxidant activities from both plant extracts, especially the breadnut, are less-studied compared to other close relative plants, such as A. heterophyllus.
Using various in vitro antioxidant assays, it could be deduced that the three best aqueous extracts that showed promising antioxidant activity were breadnut leaf, breadfruit leaf, and breadnut seed. The breadnut and breadfruit trees are perennial plants that can continue their life cycles after flowering. Although breadnut and breadfruit fruits are usually abundant during harvesting season (Luzuriaga-Quichimbo et al., Citation2019), both are still renewable resources. However, the leaves are available at any time of the year, thus making it easier to continuously acquire raw materials than the fruits. Besides, both breadnut and breadfruit leaves could be obtained in high yield after the drying process, indicating more mass of dry matter can be gathered compared to fruits.
Acute Toxicity Determination
Herbal medications may occasionally produce toxic effects (Meabed et al., Citation2018). V. harveyi is one of the marine bacteria that can emit bioluminescence. One developed method to determine the toxicity level of molecules is Microtox assay utilizing V. fischeri (Skotti et al., Citation2014). However, it is reported that the use of V. harveyi is more sensitive than Microtox assay (Thomulka et al., Citation1993) although physiological differences between two species may also play a role in the discrepancy between EC50 values. The acute toxicity assay utilizing the reduction of V. harveyi‘s bioluminescence is fast and inexpensive. Bacteria amount and species, which are related to the intensity of the luminescence, affect the EC50 values. Higher OD600 and initial bioluminescence give higher EC50 values. Originally, the bioluminescence toxicity test was purposed to test the toxicity of environmental pollutant in aquatic environment (Mariscal et al., Citation2003; Thomulka et al., Citation1993). However, this assay can also be applied to compare the toxicity level between extracts and can be used to determine the nontoxic concentration of extracts (Skotti et al., Citation2014).
EC50 values obtained from the same incubation time show significant differences to each other with Tukey’s Honest Significant Difference Test (P < .001)
The EC50 values of the extract toxicity ranged between 2471 and 7499 mg L−1 after 15 min incubation and 3026 to 7465 mg L−1 after 30 min incubation time (). All EC50 values of the extracts were far higher than the positive control. The EC50 values between extracts significantly differed from within the same incubation time. However, there were similar trends between the values of two incubation times. Consistently, the breadnut seed aqueous extract displayed the highest EC50 which indicated that the aqueous extract of breadnut seed was less toxic compared to the other aqueous extracts. Indeed, breadnut seed is often processed and consumed in form of local food and beverage (Luzuriaga-Quichimbo et al., Citation2019). Both breadnut and breadfruit fruit flesh aqueous extracts had the lowest EC50 compared to the other aqueous extracts from both incubation times. However, the EC50 values of the toxicity assay from all the aqueous extracts were higher than the IC50 values of DPPH radical scavenging activity and the EC50 values of reducing power.
Table 2. EC50 values of the toxicity assay using the reduction of V. harveyi bioluminescence from the aqueous extracts of various parts of breadnut and breadfruit
Acute toxicity test is commonly done by measuring the bioluminescence of the bacteria after exposure to a certain compound for 15 min and 30 min. Chronic effects can also be tested up to 20 h of incubation. One argued that if the EC50 value at longer incubation is lower than the value at shorter incubation time, the mode of toxicity can be concluded to be indirect and nonspecific (Nasuhoglu et al., Citation2017). Accordingly, the toxicity effects of the breadnut seed and fruit flesh aqueous extracts seem to confer specific mode of action to the V. harveyi biosynthetic pathway. We observed similar finding for ZnSO4 as the positive control used in this work. Bioluminescence inhibition assay EC50 values are also reported to be highly depending on the initial bioluminescence emission (Westlund et al., Citation2018).
Various toxicity assays such as the chronic toxicity test using the reduction of bioluminescence (Westlund et al., Citation2018) or Artemia salina brine shrimp lethality test (Ćujić et al., Citation2016) could act as alternative toxicity assay which are worth to notice. This assay indirectly correlate extracts toxicity toward microbe and toxicity to animals. The obtained EC50 can also be due to inhibition of bacterial growth or bactericidal effect (Hartung, Citation1987; Rosenkranz et al., Citation1993). Indeed, A. camansi organic extracts also displayed antimicrobial activity (Vianney et al., Citation2018). It should be noted too, that phenolic acids indicated toxicity toward various insects and mammalian herbivores. Relatively high toxicity in the fruitflesh may be also due to the plant defense to deter herbivory (Boeckler et al., Citation2011). Although the toxicity data reported here might not be fully in line with the values from assays using animal model, the acute toxicity assay using the reduction of V. harveyi bioluminescence could, nevertheless, serve as a preliminary study to compare the toxicity level of various compounds and extracts.
Conclusion
In this study, the aqueous extracts from the breadnut leaf, breadfruit leaf, and breadnut seed showed promising antioxidant activity by various in vitro assays. The EC50 toxicity values of all extracts (>2.4 mg mL−1) were higher than the effective concentration for the antioxidant activity from the various assays, indicating the extracts are safe to use and efficient in their health-related effects. All the aqueous extracts, especially the breadnut leaf, breadfruit leaf, and breadnut seed could be employed as an antioxidant and nutritional supplements. Thus, the ethnopharmacology and the usage of the leaf and fruit as a traditional food in various countries of South East Asia, the Pacific, and South American area are justified.
Acknowledgments
The authors thank Calvin Wijaya Johan for providing the V. harveyi culture. We are grateful to Prof. Gisela Buschle-Diller for proofreading, comments, and advice. We also thank the Faculty of Biotechnology for the facility support.
References
- Adeleke, R.O., and O.A. Abiodun. 2010. Nutritional composition of breadnut seeds (Artocarpus camansi). Afr. J. Agric. Res. 5:1273–1276.
- Adjimani, J.P., and P. Asare. 2015. Antioxidant and free radical scavenging activity of iron chelators. Toxicol. Rep. 2:721–728. doi: 10.1016/j.toxrep.2015.04.005.
- Ahmed, D., M.M. Khan, and R. Saeed. 2015. Comparative analysis of phenolics, flavonoids, and antioxidant and antibacterial potential of methanolic, hexanic and aqueous extracts from Adiantum caudatum leaves. Antioxidants 4(2):394–409. doi: 10.3390/antiox4020394.
- Bakar, M.F.A., M. Mohamed, A. Rahmat, and J. Fry. 2009. Phytochemicals and antioxidant activity of different parts of bambangan (Mangifera pajang) and tarap (Artocarpus odoratissimus). Food Chemistry 113(2):479–483. doi: 10.1016/j.foodchem.2008.07.081.
- Boeckler, G.A., J. Gershenzon, and S.B. Unsicker. 2011. Phenolic glycosides of the Salicaceae and their role as anti-herbivore defenses. Phytochemistry 72:1497–1509.
- Ćujić, N., K. Šavikin, T. Janković, D. Pljevljakušić, G. Zdunić, and S. Ibrić. 2016. Optimization of polyphenols extraction from dried chokeberry using maceration as traditional technique. Food Chemistry. 194:135–142. doi: 10.1016/j.foodchem.2015.08.008.
- Davalli, P., T. Mitic, A. Caporali, A. Lauriola, and D. D’Arca. 2016. ROS, cell senescence, and novel molecular mechanisms in aging and age-related diseases. Oxid. Med. Cell. Longev. 2016:3565127. doi: 10.1155/2016/3565127.
- Fernández-Agulló, A., E. Pereira, M.S. Freire, P. Valentao, P.B. Andrade, J. González-Álvarez, and J.A. Pereira. 2013. Influence of solvent on the antioxidant and antimicrobial properties of walnut (Juglans regia L.) green husk extracts. Industrial Crops and Products. 113:126–132. doi: 10.1016/j.indcrop.2012.05.021.
- Fidrianny, I., V. Virna, and M. Insanu. 2018. ANTIOXIDANT POTENTIAL OF DIFFERENT PARTS OF BOGOR PINEAPPLE (Ananas comosus [L.] MERR. VAR. QUEEN) CULTIVATED IN WEST JAVA-INDONESIA. Asian Journal of Pharmaceutical and Clinical Research 11(1):129–133. doi: 10.22159/ajpcr.2018.v11i1.22022.
- Gastaldi, B., G. Marino, Y. Assef, F.M. Sofrás, C.A.N. Catalán, and S.B. González. 2018. Nutraceutical Properties of Herbal Infusions from Six Native Plants of Argentine Patagonia. Plant Foods Hum. Nutr. 73(3):180–188. doi: 10.1007/s11130-018-0680-3.
- Gülçin, İ., Z. Huyut, M. Elmastaş, and H.Y. Aboul-Enein. 2010. Radical scavenging and antioxidant activity of tannic acid. Arab. J. Chem. 3(1):43–53. doi: 10.1016/j.arabjc.2009.12.008.
- Hakim, E.H., S.A. Achmad, L.D. Juliawaty, L. Makmur, Y.M. Syah, N. Aimi, M. Kitajima, H. Takayama, and E.L. Ghisalberti. 2006. Prenylated flavonoids and related compounds of the Indonesian Artocarpus (Moraceae). J. Nat. Med. 60:161–184.
- Hannachi, H., W. Elfalleh, M. Laajel, I. Ennajeh, R.F. Mechlouch, and K. Nagaz. 2019. Chemical Profiles and Antioxidant Activities of Leaf, Pulp, and Stone of Cultivated and Wild Olive Trees (Olea Europaea L.). Int. J. Fruit Sci 1–21. doi: 10.1080/15538362.2019.1644574.
- Hartung, J. 1987. Testing the antimicrobial activity of compounds from the air of animal houses using the Microtox test. Environ. Toxicol. 2(1):1–15.
- Hashim, N., M. Rahmani, M.A. Sukari, A.M. Ali, N.B. Alitheen, R. Go, and H.B.M. Ismail. 2010. Two new xanthones from Artocarpus obtusus. J. Asian Nat. Prod. Res. 12(2):106–112. doi: 10.1080/10286020903450411.
- Hendry, G.A.F., K. Thompson, C.J. Moss, E. Edwards, and P.C. Thorpe. 1994. Seed persistence: A correlation between seed longevity in the soil and ortho-dihydroxyphenol concentration. Functional Ecology 8(5):658–664. doi: 10.2307/2389929.
- Jagtap, U.B., and V.A. Bapat. 2010. Artocarpus: A review of its traditional uses, phytochemistry and pharmacology. J. Ethnopharmacol. 129(2):142–166. doi: 10.1016/j.jep.2010.03.031.
- Jagtap, U.B., S.N. Panaskar, and V.A. Bapat. 2010. Evaluation of antioxidant capacity and phenol content in jackfruit (Artocarpus heterophyllus Lam.) fruit pulp. Plant Foods for Human Nutrition 65(2):99–104. doi: 10.1007/s11130-010-0155-7.
- Jalal, T.K., I.A. Ahmed, M. Mikail, L. Momand, S. Draman, M.L.M. Isa, M.S.B. Rasad, M.N. Omar, M. Ibrahim, and R.A. Wahab. 2015. Evaluation of antioxidant, total phenol and flavonoid content and antimicrobial activities of Artocarpus altilis (Breadfruit) of underutilized tropical fruit extracts. Appl. Biochem. Biotechnol. 129(7):3231–3243. doi: 10.1007/s12010-015-1499-0.
- Jayasinghe, U.L.B., T.B. Samarakoon, B.M.M. Kumarihamy, N. Hara, and Y. Fujimoto. 2008. Four new prenylated flavonoids and xanthones from the root bark of Artocarpus nobilis. Fitoterapia 79(1):37–41. doi: 10.1016/j.fitote.2007.07.014.
- Jones, A.M.P., S.J. Murch, J. Wiseman, and D. Ragone. 2013. Morphological diversity in breadfruit (Artocarpus, Moraceae): Insights into domestication, conservation, and cultivar identification. Genetic Resources and Crop Evolution 60(1):175–192. doi: 10.1007/s10722-012-9824-8.
- Kasangana, P.B., P.S. Haddad, and T. Stevanovic. 2015. Study of polyphenol content and antioxidant capacity of Myrianthus arboreus (Cecropiaceae) root bark extracts. Antioxidants 4(2):410–426. doi: 10.3390/antiox4020410.
- Khoddami, A., M. Wilkes, and T. Roberts. 2013. Techniques for analysis of plant phenolic compounds. Molecules 18(2):2328–2375. doi: 10.3390/molecules18022328.
- Li, W.J., X.L. Cheng, J. Liu, R.C. Lin, G.L. Wang, S.S. Du, and Z.L. Liu. 2012. Phenolic compounds and antioxidant activities of Liriope muscari. Molecules 17(2):1797–1808. doi: 10.3390/molecules17021797.
- Liu, Y., D. Ragone, and S.J. Murch. 2015. Breadfruit (Artocarpus altilis): A source of high-quality protein for food security and novel food products. Amino Acids 47(4):847–856. doi: 10.1007/s00726-015-1914-4.
- Loganayaki, N., P. Siddhuraju, and S. Manian. 2013. Antioxidant activity and free radical scavenging capacity of phenolic extracts from Helicteres isora L. and Ceiba pentandra L.. J. Food. Sci. Tech. 50(4):687–695. doi: 10.1007/s13197-011-0389-x.
- Loizzo, M.R., R. Tundis, U.G. Chandrika, A.M. Abeysekera, F. Menichini, and N.G. Frega. 2010. Antioxidant and antibacterial activities on foodborne pathogens of Artocarpus heterophyllus Lam. (Moraceae) Leaves Extracts. J. Food. Sci. 75:M291–M295.
- Luzuriaga-Quichimbo, C.X., J. Blanco-Salas, C.E. Cerón-Martínez, and T. Ruiz-Téllez. 2019. Providing added value to local uses of paparahua (Artocarpus altilis) in Amazonian Ecuador by phytochemical data review. Revista Brasileira De Farmacognosia 29(1):62–68. doi: 10.1016/j.bjp.2018.09.008.
- Makmur, L., S. Syamsurizal, T. Achmad, S.A. Achmad, N. Aimi, E.H. Hakim, M. Kitajima, and H. Takayama. 2000. Artoindonesianin C, a New Xanthone Derivative from Artocarpus teysmanii. J. Nat. Prod. 63(2):243–244. doi: 10.1021/np990220u.
- Mariscal, A., M.T. Peinado, M. Carnero-Varo, and J. Fernández-Crehuet. 2003. Influence of organic solvents on the sensitivity of a bioluminescence toxicity test with Vibrio harveyi. Chemosphere 50(3):349–354. doi: 10.1016/S0045-6535(02)00312-0.
- Méabed, E.M.H., N.M. El-Sayed, A.I.B. Abou-Sreea, and M.H.H. Roby. 2018. Chemical analysis of aqueous extracts of Origanum majorana and Foeniculum vulgare and their efficacy on Blastocystis spp . cysts. Cysts. Phytomedicine. 43:158–163. doi: 10.1016/j.phymed.2018.04.017.
- Mierziak, J., K. Kostyn, and A. Kulma. 2014. Flavonoids as important molecules of plant interactions with the environment. Molecules 19(10):16240–16265. doi: 10.3390/molecules191016240.
- Mittler, R. 2017. ROS are good. Trends in Plant Science 22(1):11–19. doi: 10.1016/j.tplants.2016.08.002.
- Mushtaq, M., B. Sultana, F. Anwar, A. Adnan, and S.S.H. Rizvi. 2015. Enzyme-assisted supercritical fluid extraction of phenolic antioxidants from pomegranate peel. The Journal of Supercritical Fluids. 104:122–131. doi: 10.1016/j.supflu.2015.05.020.
- Nasuhoglu, D., P. Westlund, S. Isazadeh, S. Neamatallah, and V. Yargeau. 2017. Development of a facile and high-throughput bioluminescence assay using Vibrio fischeri to determine the chronic toxicity of contaminated samples. . Bulletin of Environmental Contamination and Toxicology 98(2):196–203. doi: 10.1007/s00128-016-2008-z.
- Nnam, N.M., and M.O. Nwokocha. 2003. Chemical and organoleptic evaluation of biscuits made from mixtures of hungry rice, acha (Digitaria exilis) sesame; (Sesamum indicum); and breadfruit (Artocarpus atilis) flours. Plant Foods Hum. Nutr. 58:1–11.
- Ragone, D., and C.G. Cavaletto. 2006. Sensory evaluation of fruit quality and nutritional composition of 20 breadfruit (Artocarpus, Moraceae) cultivars. Econ. Bot. 60(4):335–346. doi: 10.1663/0013-0001(2006)60[335:SEOFQA]2.0.CO;2.
- Roby, M.H.H., M.A. Sarhan, K.A.-H. Selim, and K.I. Khalel. 2013. Antioxidant and antimicrobial activities of essential oil and extracts of fennel (Foeniculum vulgare L.) and chamomile (Matricaria chamomilla L.). . Industrial Crops and Products. 44:437–445. doi: 10.1016/j.indcrop.2012.10.012.
- Rosenkranz, H.S., J. Pangrekar, and G. Klopman. 1993. Similarities in the mechanisms of antibacterial activity (Microtox™ assay) and toxicity to vertebrates. Alternatives to Laboratory Animals 21(4):489–500. doi: 10.1177/026119299302100412.
- Sahreen, S., M.R. Khan, and R.A. Khan. 2010. Evaluation of antioxidant activities of various solvent extracts of Carissa opaca fruits. Food Chemistry 122(4):1205–1211. doi: 10.1016/j.foodchem.2010.03.120.
- Salleh, R.M., and N.S.M. Jinis. 2016. Proximate composition, mineral and total phenolic contents, and scavenging activity of breadnut fruit (Artocarpus camansi). J. Trop. Agric. Food Sci. 44:1–7.
- Santos, P.A., L.C. de Rezende, J.C.S.D. Oliveira, J.M. David, and J.P. David. 2019. Chemical Study, Antioxidant and Cytotoxic Activities of Oil Seeds of Spondias tuberosa (Anacardiaceae). . International Journal of Fruit Science 22(1):246–257. doi: 10.1080/15538362.2018.1502721.
- Shahidi, F., and P. Ambigaipalan. 2015. Phenolics and polyphenolics in foods, beverages and spices: Antioxidant activity and health effects – A review. . Journal of Functional Foods. 18:820–897. doi: 10.1016/j.jff.2015.06.018.
- Sidahmed, H.M.A., N.M. Hashim, J. Amir, M.A. Abdulla, A.H.A. Hadi, S.I. Abdelwahab, M.M.E. Taha, P. Hassandarvish, X. Teh, M.F. Loke, et al. 2013. Pyranocycloartobiloxanthone A, a novel gastroprotective compound from Artocarpus obtusus Jarret, against ethanol-induced acute gastric ulcer in vivo. Phytomedicine 20(10):834–843. doi: 10.1016/j.phymed.2013.03.002.
- Skotti, E., E. Anastasaki, G. Kanellou, M. Polissiou, and P.A. Tarantilis. 2014. Total phenolic content, antioxidant activity and toxicity of aqueous extracts from selected Greek medicinal and aromatic plants. Ind. Crops Prod. 53:46–54. doi: 10.1016/j.indcrop.2013.12.013.
- Tamuly, C., R. Buragohain, M. Hazarika, J. Bora, and P.R. Gajurel. 2015. Assessment of Antioxidant Activity of Six Ficus Species—Underutilized Fruits from Arunachal Pradesh in North East India. International Journal of Fruit Science 15(1):85–99. doi: 10.1080/15538362.2014.931174.
- Thomulka, K.W., D.J. McGee, and J.H. Lange. 1993. Detection of biohazardous materials in water by measuring bioluminescence reduction with the marine organism Vibrio harveyi. J. Environ. Sci. Health A 28(9):2153–2166.
- Tuominen, A. 2013. Defensive strategies in Geranium sylvaticum, Part 2: Roles of water-soluble tannins, flavonoids and phenolic acids against natural enemies. Phytochemistry 95:408-420.
- Tuominen, A., E. Toivonen, P. Mutikainen, and J.-P. Salminen. 2013. Defensive strategies in Geranium sylvaticum. Part 1: Organ-specific distribution of water-soluble tannins, flavonoids and phenolic acids. Phytochemistry. 95:394–407. doi: 10.1016/j.phytochem.2013.05.013.
- Vianney, Y.M., N. Amanda, K. Pieknell, C.W. Johan, and P.H. Hardjo. 2018. Evaluation of the antioxidant and antibacterial activity of breadnut (Artocarpus camansi Blanco) leaf extracts. Indian J. Nat. Prod. Resour. 9:151–159.
- Westlund, P., D. Nasuhoglu, S. Isazadeh, and V. Yargeau. 2018. Investigation of acute and chronic toxicity trends of pesticides using high-throughput bioluminescence assay based on the test organism Vibrio fischeri. Archives of Environmental Contamination and Toxicology 74(4):557–567. doi: 10.1007/s00244-017-0483-9.
- Zerega, N., T. Wiesner-Hanks, D. Ragone, B. Irish, B. Scheffler, S. Simpson, and F. Zee. 2015. Diversity in the breadfruit complex (Artocarpus, Moraceae): Genetic characterization of critical germplasm. Tree Genetics & Genomes 11(1):4. doi: 10.1007/s11295-014-0824-z.
- Zerega, N.J.C., D. Ragone, and T.J. Motley. 2005. Systematics and Species Limits of Breadfruit (Artocarpus, Moraceae). Syst. Bot. 30(3):603–615. doi: 10.1600/0363644054782134.
- Zhang, Y.J., R.Y. Gan, S. Li, Y. Zhou, A.N. Li, D.P. Xu, and H.B. Li. 2015. Antioxidant phytochemicals for the prevention and treatment of chronic diseases. Molecules 20(12):21138–21156. doi: 10.3390/molecules201219753.