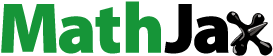
ABSTRACT
Drying is an essential unit operation in the food industry, with novel technologies introduced day after day. This study aimed to investigate different drying methods of apple using the continuous microwave, pulsed microwave (PM), and convective hot-air (CH) with and without ultrasound (U) pretreatment. Results showed that simultaneous U – PM – CH drying significantly reduced drying time. Shrinkage of the samples decreased when microwave power and pulse ratio were increased. Bulk density reduced by increasing the U duration and pulse ratio. The maximum raise in rehydration (39.35%) was seen in the dried samples pretreated at a higher U duration (30 min). By raising the U time and microwave power, specific energy consumption reduced by 30.05 and 41.36%, respectively. Overall, the U – PM – CH drying enhanced the quality of the dried apples and could be suggested for commercial drying industries.
Introduction
Apple, scientifically known as Malus Domestica, belongs to the family of Rosacea. This fruit is a rich source of phenolic compounds with a high amount of free polyphenols (Rodríguez et al., Citation2014). Apple is generally eaten fresh, juiced, and dried. The health benefits of apple encourage researchers to find a way to produce dehydrated products with high quality. Drying is a complex process of preserving food, comprising heat and mass transfer under an unsteady-state condition (Cruz et al., Citation2015). A suitable drying method should maintain maximum quality in shorter processing time, leading to consuming less energy (Chong et al., Citation2013).
Convective hot-air (CH) drying is a popular drying method (Brar et al., Citation2020; Ullah et al., Citation2018). However, it has unfavorable effects on the quality of dried foods. It also has a long drying time with low energy efficiency (Brar et al., Citation2020). The exposure to prolonged moisture removal adversely changes taste, odor, color, and nutrients of dried products (Horuz and Maskan, Citation2015). This drying method results in structural damage during drying, which, in turn, increases shrinkage and reduces rehydration rate (Horuz and Maskan, Citation2015; Tsuruta et al., Citation2015). By increasing drying temperature, the processing time is decreased and, therefore, the exposure to heat is reduced. On the other hand, high temperatures have some disadvantages due to the vulnerability of some foods to heat, browning reactions, and damage to organoleptic properties such as texture and color (Zlatanović et al., Citation2013). Drying at low temperatures minimizes severe reductions in the moisture content of the food surface, leading to prevent surface hardening. Low temperatures also prevent structural cracking by reducing the internal vapor pressure (Rodriguez-Ramirez et al., Citation2012).
New processes in the food industry are designed to overcome the drawbacks associated with CH drying, produce products with high quality, and enhance energy efficiency. One of these methods is ultrasound (U) processing, which is non-thermal and applied in different fields of the food industry (Tao and Sun, Citation2015). Ultrasound waves produce a cycle of the sound pressure, the frequency of which exceeds 20 kHz. These waves can be used as a pretreatment before CH drying to increase the drying rate and the quality of dried fruits (Azoubel et al., Citation2010; Fernandes et al., Citation2008). Ultrasound waves produce a series of continuous contractions and expansions inside a food structure called sponge effect (Mothibe et al., Citation2014). The forces involved in this mechanism break the cells, creating microscopic channels within the food, which, in turn, facilitates the removal of bound water, reduces drying time and saves energy (Fernandes and Rodrigues, Citation2007). Low frequency-high intensity U waves damage the cellular structure and its membrane, leading to increase the moisture transfer between the cell and its surrounding (Dehghannya et al., Citation2016). Besides, the U can improve the quality of the dried foods because heating is not involved during its application. Meanwhile, easier moisture removal makes drying at lower temperatures possible, which reduces shrinkage, improves rehydration properties, and increases drying efficiency (Nowacka et al., Citation2012).
In addition to the U processing, the application of microwave during drying of food products is another technique to resolve the problems related to CH drying. The microwave heat is generated by bipolar rotation and ionic polarization. Continuous changes in the electromagnetic field and, subsequently, molecular orientation creates friction between molecules, resulting in heat generation. Heat transfers from inside to outside of the food during microwave drying, unlike traditional methods of drying. This results in reaching the temperature of interest rapidly, leading to produce dried products with favorable quality. It also consumes less energy due to the reduction in drying time. Microwave drying is usually used with CH drying. In this hybrid method, the internal and surface moisture of the product is, respectively, removed by the microwave and hot air. As a result, efficiency is enhanced, and less energy is consumed (Andrés et al., Citation2004). Microwave and CH drying methods are combined using three strategies: 1) Using microwave at the start of drying. It brings the inner body of the product to the evaporation temperature right away; water vapor reaches the outer surface, resulting in moisture removal by the hot air. The porosity of food affects the drying rate by facilitating the transfer of water vapor. 2) Using microwave in the middle of drying, when the drying rate starts to decrease. Under this condition, concerning the fact that the surface is dried, but the inner parts still have moisture, microwave eases moisture transfer from the inside to the outside by enhancing vapor pressure. 3) Using microwave at the end of drying, to easily remove the bound water and reduce shrinkage (Andrés et al., Citation2004).
The high rate of heat transfer in the continuous microwave during drying may result in structural damages, leading to increase shrinkage. High temperatures generated by microwaves might also cause structural damages to heat-sensitive foods (Kumar et al., Citation2015). Besides, hot spot developments in continuous microwave drying degrade the product quality due to non-uniform moisture and temperature distributions. Therefore, microwaves can be used intermittently to reduce this negative impact (Zhao et al., Citation2014). PM drying is a method in which the heat-generating source is turned on and off at specific time intervals. The pulsed process is an alternative method to avoid non-uniform heating and improve the quality by redistributing the temperature and moisture inside the product while the microwave is off. PM significantly enhances moisture removal by generating volumetric heat inside the product (Kumar et al., Citation2014). Today, combined PM – CH drying is used in the food industry due to consuming less energy, reducing the processing time, and producing high-quality products. According to the literature, there are limited comparative investigations regarding continuous microwave, PM, and low-temperature CH drying methods with and without applying the U. This study aimed to assess the impact of the drying procedures on some qualitative and quantitative characteristics of apple.
Materials and Methods
Raw Material
Apple samples (Golden Delicious) were obtained from a local market and kept in a refrigerator at 4°C until carrying out the experiments.
Equipment
An ultrasonic bath (As One, US-4 R, Japan) with the frequency of 28 and 40 kHz was used to conduct the U pretreatment. A microwave – CH dryer (LG SolarDOM, SD-3855SCR, South Korea) was used with adjustable microwave output power between 90 to 900 W at 2450 MHz and hot-air temperature ranging from 40 to 230°C. An oven (Fan Azma Gostar, BM120, Iran) was used to measure the samples’ moisture content. To blanch the apple samples, a bain-marie (Fan Azma Gostar, WM22, Iran) equipped with a microcontroller with a precision of ±1°C was used. A digital balance (Adam Equipment, UK) with an accuracy of ±0.01 g was used to weigh the samples.
Sample Preparation
Before starting the experiments, the apple samples were kept at room temperature to reach equilibrium. The fruits were first washed by tap water and, after peeling, were cut into 1.2 cm cubes. The blanching of the samples was done at 100°C for 3 min inside beakers filled with water in the bain-marie. The samples were then immediately cooled down with water for 5 min at 15°C to eliminate excess heat. The excess moisture was removed by a moisture absorbent paper (Mothibe et al., Citation2014).
Ultrasound Pretreatment
Apple samples (150 g) were placed in beakers filled with distilled water (sample to water ratio of 1:4 w/w), and then, the beakers were placed in the U bath. The U pretreatment was conducted at 40 kHz frequency at four-time levels of 0 (control), 10, 20, and 30 min. According to the output power of the ultrasonic bath (160 W), the samples pretreated with ultrasound for 0, 10, 20 and 30 min, used 0, 0.096, 0.192, and 0.288 MJ energy, respectively. At the end of the U pretreatment, the excess moisture was taken by an absorbent paper. The temperature of the water in the U bath was held at 25°C. The temperature increase after 30 min of U pretreatment was negligible (2°C) (Dehghannya et al., Citation2015).
Drying Experiments
Microwave – CH drying was performed at 360, 600, and 900 W powers at pulse ratios of 1, 2, 3, and 4 (). The pulse ratio (PR) was determined by (Zhao et al., Citation2014):
Table 1. On/off times at different microwave powers and pulse ratios
where ton is the microwave on-time (s), and toff is the microwave off-time (s). After completing the microwave drying, the process was carried on by hot air at 40°C and 1 m/s until reaching the moisture content of 0.2 g water/g dry solids or lower.
Measurement of Moisture Content
The moisture content of the samples was measured by the oven drying for 24 h at 105°C to reach a constant weight. The moisture content was obtained using (Deng and Zhao, Citation2008):
where Mw is the mass of water (g), and Ms is the mass of dry solids (g).
Measurement of Shrinkage
Shrinkage was obtained by the method explained by Nowacka et al. (Citation2012):
where S is the shrinkage, Vt is the volume of the sample at time t (cm3), and V0 is the initial volume (cm3).
The toluene displacement technique was used to obtain the volume (Yan et al., Citation2008):
where Vf is the flask volume (cm3), Msf is the toluene mass added to fill the flask (g), Mt+s is the flask mass filled with the sample and toluene (g), Mf is the flask mass (g), M is the sample mass (g), and ρs is the density of toluene (0.87 g/cm3 at 20°C).
Measurement of Bulk Density
The bulk density (ρb) was measured as the ratio of the sample’s mass at time t (mt) to its volume (Vt) (Koc et al., Citation2008):
Measurement of Rehydration Ratio
The dried samples were immersed in distilled water at 100°C for 2 min (Aghilinategh et al., Citation2015). The rehydration ratio was calculated as:
where Wr is the mass of the rehydrated sample (g), and Wd is the mass of the dried sample (g).
Measurement of Specific Energy Consumption
The specific energy consumption (E) was calculated as the sum of the energy consumption during microwave drying (E1; MJ/kg water), and the energy consumption during CH drying (E2; MJ/kg water) (Aghilinategh et al., Citation2015; Chayjan et al., Citation2015; Singh and Heldman, Citation2014):
where P is the power of microwave (W), tm is the drying time of microwave (s), PR is the pulse ratio of microwave, mvm is the mass of water removed during microwave drying (kg), A is the area of the sample container (m2), Va is the velocity of air (m/s), ρa is the density of air (kg/m3), and ΔH is the enthalpy of air (kJ/kg dry air). Besides, tc is the drying time of CH (s), mvc is the mass of water removed during CH drying (kg), Cp,a is the specific heat of the air (kJ/kg °C), W is the absolute humidity of the air (kg water vapor/kg dry air), Cp,v is the specific heat of the water vapor (kJ/kg °C), Tin is the air temperature inside the dryer (°C), Tamb is the ambient temperature (°C), and λ is the latent heat of vaporization (kJ/kg water vapor).
Statistical Analysis
A factorial experiment was executed in SAS (version 9.4) using a completely randomized design (CRD) framework. The examined factors included the duration of exposure to the U (0, 10, 20, and 30 min), the microwave output power (360, 600, and 900 W), and the pulse ratio (1, 2, 3, and 4). The experiments were conducted in three replications (Total treatments: 4 × 3 × 4 × 3 = 144). A comparison of means was made using Duncan’s multiple range test at the probability level of 5%.
Results and Discussion
Drying Kinetics
The moisture content in all the treatments continuously reduced by increasing drying time (). The moisture removal rate decreased over time, as indicated by the slopes. At the beginning of the process (when using the microwave drying), the steep slope of the curves showed the high drying rate ( and ) due to the volumetric heating of microwaves. Indeed, thermal conductivity, a function of moisture content, decreased as the moisture content reduced (Kumar et al., Citation2015). Additionally, the moisture content of the samples increased after the U pretreatment (the starting point of the diagrams) ( and ). The increase in moisture content during the U pretreatment was due to the presence of a concentration gradient that transfers solids from the product to the fluid medium and transfers moisture from the liquid medium to the product (Fernandes and Rodrigues, Citation2007).
Figure 1. Drying kinetics of the samples pretreated with ultrasound for of 0 (control), 10, 20 and 30 min during pulsed microwave – convective hot-air drying at 360 W with different pulse ratios (PR) of 1 (a), 2 (b), 3 (c), and 4 (d)
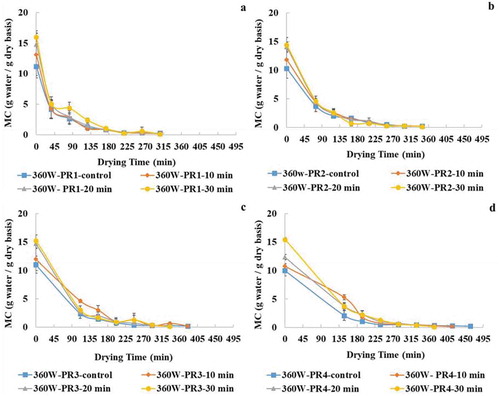
Figure 2. Magnitudes of moisture content after ultrasound pretreatment (MC-U), moisture content after microwave drying (MC-MD), and final moisture content (MC-F) of the samples as influenced by ultrasonication time [0 (control), 10, 20, and 30 min] and pulse ratio [PR = 1, 2, 3, and 4] at microwave powers of 360 W (a), 600 W (b), and 900 W (c)
![Figure 2. Magnitudes of moisture content after ultrasound pretreatment (MC-U), moisture content after microwave drying (MC-MD), and final moisture content (MC-F) of the samples as influenced by ultrasonication time [0 (control), 10, 20, and 30 min] and pulse ratio [PR = 1, 2, 3, and 4] at microwave powers of 360 W (a), 600 W (b), and 900 W (c)](/cms/asset/50ff0155-31da-4fcb-aef1-ce4eb8803a62/wsfr_a_1830919_f0002_oc.jpg)
Generally, as the duration of the U pretreatment increased, the final moisture content significantly decreased ( and ) (Roueita et al., Citation2020). Ultrasound waves ease moisture removal due to the creation of microscopic channels in the food structure, leading to enhance the drying rate (Fernandes and Rodrigues, Citation2007). The drying time significantly decreased by increasing the U pretreatment time (). The minimum time required to dry the samples was obtained after 30 min of the U pretreatment (). This result was ascribed to the structural changes of the samples pretreated with the 30-min U and improvement in the moisture removal paths (Dehghannya et al., Citation2015).
Table 2. Mean comparison of various indexes including moisture content (MC), drying time (DT), bulk density (BD), shrinkage (Sh), rehydration ratio (RR) and specific energy consumption (SEC) of samples as influenced by different process variables
Besides, the moisture content significantly decreased as the pulse ratio increased ( and ). By increasing the pulse ratio, temperature and moisture are redistributed within the product when the microwave power is off, leading to facilitate the removal of moisture during the microwave on-time (Aghilinategh et al., Citation2015). Easier removal of moisture due to the application of the PM at higher pulse ratios reduced the drying time ().
Furthermore, by increasing the microwave power from 360 to 600 and 900 W, the final moisture content of the samples significantly decreased ( and ). Horuz and Maskan (Citation2015) obtained similar results. The application of high microwave powers results in increased internal vapor pressure and accelerates moisture removal. The drying time decreased as the microwave power was increased (). Further heating at higher powers accelerated mass transfer, leading to reduce the drying time.
Shrinkage
Shrinkage adversely affects the quality of dried products. During the drying process, the loss of moisture imposes stress on the food structure, leading to change the shape of the product. When shrinkage does not equally occur in the whole part of a product, it results in cracking on the product’s surface. Shrinkage also reduces rehydration capacity. During the drying process, shrinkage takes place by merging the viscoelastic matrix into space where previously filled by water (Akbarian et al., Citation2015). Results showed that the shrinkage of the samples increased as the process proceeded (). Shrinkage raised further during the CH drying after the microwave drying completed ( and ). This could happen due to a drop in heat transfer and removal of cellular water during the prolonged CH drying (Akbarian et al., Citation2015).
Figure 3. Shrinkage of the samples pretreated with ultrasound for of 0 (control), 10, 20 and 30 min during pulsed microwave – convective hot-air drying at 360 W with different pulse ratios (PR) of 1 (a), 2 (b), 3 (c), and 4 (d)
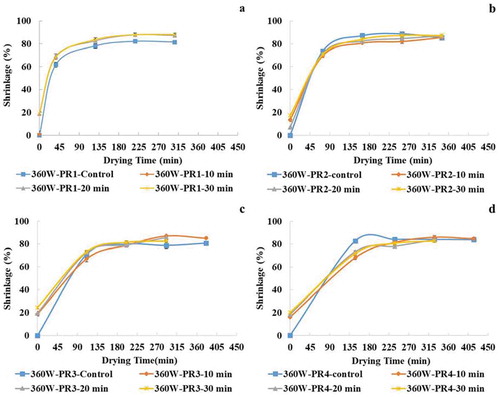
Generally, the shrinkage significantly increased by increasing the U duration () (Roueita et al., Citation2020). Consistent with this result, Nowacka et al. (Citation2012), who exposed the samples of apple to the U pretreatment for 10, 20, and 30 min, reported that control samples without ultrasound pretreatment had the lowest shrinkage. The higher shrinkage with the increased U duration could be attributed to the development of pores and microscopic channels during the pretreatment, and, as a result, higher stress on the products’ cellular structure during drying (Nowacka et al., Citation2012).
Besides, the shrinkage decreased by increasing the pulse ratio (). This result could be ascribed to the uniform moisture and temperature distributions inside the food product during the off-times of the PM drying, leading to an increase in the moisture removal rate, and a decrease in the drying time (Dehghannya et al., Citation2018a). Moisture removal accelerated at higher pulse ratios (), resulting in reducing the shrinkage.
Furthermore, the shrinkage of the samples reduced as the microwave power was raised (). This result could be attributed to a decrease in the length of time that the microwave energy was applied as its power was increased (). Additionally, the reduction in the time required for drying by increasing microwave power () might prevent increased structural damages and eventually could reduce the shrinkage (Dehghannya et al., Citation2018b).
Bulk Density
Changes in the bulk density are dependent on drying conditions and have a significant effect on drying rate and structural variations of food products. Bulk density influences most thermophysical properties of foods. Some physical properties, such as porosity and density, are also dependent on the changes in shrinkage during the drying process (Dehghannya et al., Citation2015).
Generally, bulk density reduced by increasing the U pretreatment time ( and ) (Nowacka et al., Citation2012). This result could be attributed to the acceleration of moisture removal in the U-pretreated samples () and the creation of more microchannels, leading to increase porosity (Aghilinategh et al., Citation2015). Additionally, bulk density during the PM drying (pulse ratios of 2, 3, and 4) decreased compared to the continuous microwave (pulse ratio of 1) (). This result was ascribed to the moisture and temperature redistributions during the microwave off-times, and, structural improvements, leading to decrease shrinkage () (Aghilinategh et al., Citation2015). Moreover, the bulk density generally increased by raising the microwave power (). Fang et al. (Citation2010) obtained similar results during microwave drying of jujube. The increase could be attributed to the internal structural damages and the dried surface of the samples, leading to a decrease in porosity (Fang et al., Citation2010).
Rehydration
Rehydration, while reconstituting the properties of dried foods, serves as a criterion indicating the damage incurred during the drying process (Dehghannya et al., Citation2018c). Blanching, drying method, physical and chemical structure, volume, and density affect rehydration. Rehydration occurs over time, with an increase in the volume and moisture content of dried products (Tao and Sun, Citation2015).
Results showed that as the drying process proceeded, the rehydration increased (). Besides, rehydration significantly enhanced by increasing the U pretreatment time (). The higher the U pretreatment time, the more the creation of the structural pores (Tao and Sun, Citation2015), and, subsequently, the easier the moisture transfer (). This result could also be attributed to the reduction in bulk density by raising the U time (), leading to increase porosity and rehydration (Nowacka et al., Citation2012). Mothibe et al. (Citation2014) also showed that the rehydration increased by increasing the U pretreatment time. They attributed this result to the cellular breakdown during the U pretreatment, leading to decrease resistance against moisture flow.
Figure 5. Rehydration ratio of the samples pretreated with ultrasound for of 0 (control), 10, 20 and 30 min during pulsed microwave – convective hot-air drying at 360 W with different pulse ratios (PR) of 1 (a), 2 (b), 3 (c), and 4 (d)
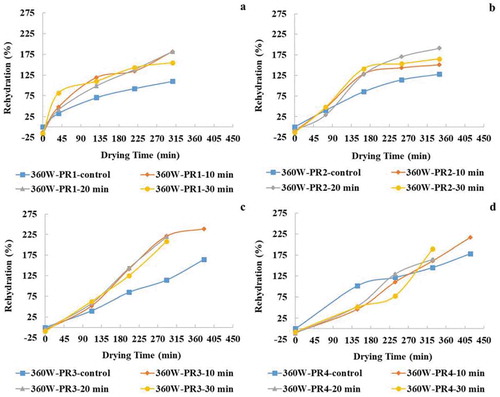
Moreover, the PM drying (pulse ratios of 2, 3, and 4) significantly increased the rehydration compared to the continuous microwave (pulse ratio of 1) (). This result was attributed to the temperature and moisture redistributions when microwave power was off, leading to improve the food structure (Aghilinategh et al., Citation2015). The rehydration increase during the PM drying could also be ascribed to the shrinkage decrease during the PM drying ().
Besides, rehydration significantly increased by raising the microwave power () (Aghilinategh et al., Citation2015). Microwaves at higher powers create a higher capacity for rehydration and restoration of the dried structure through increasing the internal pressure of the sample, and creating a porous structure in the product (Aghilinategh et al., Citation2015). Kesbi et al. (Citation2016) studied the impact of combined microwave – CH drying on lemon slices. The method enhanced the quality of the dried products due to decreasing the processing time. The improvement in quality was more significant compared to the individual use of the CH or microwave. The highest rehydration (94.16%) was observed in the combined method due to structural damages that occurred in the CH-only-dried samples.
Specific Energy Consumption
In addition to the production of high-quality products, all industries primarily intend to increase economic efficiency and reduce costs. The application of ultrasound and microwaves during drying could be considered an economical way to dry foods due to decreasing drying time (Tao and Sun, Citation2015).
Results showed that, generally, the energy consumption significantly reduced by increasing the U pretreatment time ( and ) (Fernandes and Rodrigues, Citation2007). The increase in the U duration influences the sample structure. It creates more microscopic channels, which, in turn, helps to raise the drying rate and decrease energy consumption by reducing the time required for drying (Fernandes and Rodrigues, Citation2007).
Figure 6. Specific energy consumption of microwave drying (SE-MD), specific energy consumption of hot-air drying (SE-HA), and total specific energy consumption (SE-Total) as influenced by ultrasonication time [0 (control), 10, 20, and 30 min] and pulse ratio [PR = 1, 2, 3, and 4] at microwave powers of 360 W (a), 600 W (b), and 900 W (c)
![Figure 6. Specific energy consumption of microwave drying (SE-MD), specific energy consumption of hot-air drying (SE-HA), and total specific energy consumption (SE-Total) as influenced by ultrasonication time [0 (control), 10, 20, and 30 min] and pulse ratio [PR = 1, 2, 3, and 4] at microwave powers of 360 W (a), 600 W (b), and 900 W (c)](/cms/asset/5f5c9741-fa04-42e7-81a8-222763cb3158/wsfr_a_1830919_f0006_oc.jpg)
Moreover, energy consumption decreased when the pulse ratio was increased ( and ) due to the shortened drying time (). The energy consumption also reduced significantly by increasing the microwave power ( and ) (Aghilinategh et al., Citation2015). This was due to lowering the microwave application time (). Andrés et al. (Citation2004) showed that the use of microwave before CH drying significantly shortened the processing time and decreased energy consumption. Application of the simultaneous microwave and CH drying accelerated the drying process. Kumar et al. (Citation2015) assessed the influence of PM and CH during the drying of apple. The combined PM – CH drying accelerated the drying rate by four times compared to the CH drying, resulting in a considerable reduction in energy consumption.
Conclusions
Both continuous and pulsed microwave (PM) coupled with convective hot-air (CH) with and without ultrasound (U) intensification during drying of apple was investigated. The U application facilitated moisture removal, leading to accelerate drying rate and decrease the final moisture content of the product. By enhancing the microwave power from 360 to 900 W and pulse ratio from 1 to 4, moisture removal increased, and shrinkage decreased. Besides, bulk density reduced as the U application time increased from 0 to 30 min, leading to an increase in the volume of the dried products. By increasing the U time, the microwave power, and the pulse ratio, rehydration increased, and specific energy consumption decreased. In conclusion, the combined U – PM – CH drying improved the quality of dehydrated apples and might be suggested as an alternative drying method for the food industry.
References
- Aghilinategh, N., S. Rafiee, A. Gholikhani, S. Hosseinpur, M. Omid, S.S. Mohtasebi, and N. Maleki. 2015. A comparative study of dried apple using hot air, intermittent and continuous microwave: Evaluation of kinetic parameters and physicochemical quality attributes. Food Science and Nutrition 3(6):519–526. doi: 10.1002/fsn3.241.
- Akbarian, M., B. Ghanbarzadeh, M. Sowti, and J. Dehghannya. 2015. Effects of pectin-CMC-based coating and osmotic dehydration pretreatments on microstructure and texture of the hot-air dried quince slices. Journal of Food Processing and Preservation 39(3):260–269. doi: 10.1111/jfpp.12229.
- Andrés, A., C. Bilbao, and P. Fito. 2004. Drying kinetics of apple cylinders under combined hot air–microwave dehydration. Journal of Food Engineering 63(1):71–78. doi: 10.1016/S0260-8774(03)00284-X.
- Azoubel, P.M., M.D.A.M. Baima, M.D.R. Amorim, and S.S.B. Oliveira. 2010. Effect of ultrasound on banana cv pacovan drying kinetics. Journal of Food Engineering 97(2):194–198. doi: 10.1016/j.jfoodeng.2009.10.009.
- Brar, H.S., P. Kaur, J. Subramanian, G.R. Nair, and A. Singh. 2020. Effect of chemical pretreatment on drying kinetics and physiochemical characteristics of yellow European plums. International Journal of Fruit Science. 1–28. doi: 10.1080/15538362.2020.1717403.
- Chayjan, R.A., M. Kaveh, and S. Khayati. 2015. Modeling drying characteristics of hawthorn fruit under microwave-convective conditions. Journal of Food Processing and Preservation 39(3):239–253. doi: 10.1111/jfpp.12226.
- Chong, C.H., A. Figiel, C.L. Law, and A. Wojdyło. 2013. Combined drying of apple cubes by using of heat pump, vacuum-microwave, and intermittent techniques. Food and Bioprocess Technology 7(4):975–989. doi: 10.1007/s11947-013-1123-7.
- Cruz, A.C., R.P.F. Guiné, and J.C. Gonçalves. 2015. Drying kinetics and product quality for convective drying of apples (cvs Golden Delicious and Granny Smith). International Journal of Fruit Science 15(1):54–78. doi: 10.1080/15538362.2014.931166.
- Dehghannya, J., S. Bozorghi, and M.K. Heshmati. 2018a. Low temperature hot air drying of potato cubes subjected to osmotic dehydration and intermittent microwave: Drying kinetics, energy consumption and product quality indexes. Heat and Mass Transfer 54(4):929–954. doi: 10.1007/s00231-017-2202-5.
- Dehghannya, J., P. Farshad, and M.K. Heshmati. 2018b. Three-stage hybrid osmotic–intermittent microwave–convective drying of apple at low temperature and short time. Drying Technology 36:1982–2005.
- Dehghannya, J., R. Gorbani, and B. Ghanbarzadeh. 2015. Effect of ultrasound-assisted osmotic dehydration pretreatment on drying kinetics and effective moisture diffusivity of Mirabelle plum. Journal of Food Processing and Preservation 39(6):2710–2717. doi: 10.1111/jfpp.12521.
- Dehghannya, J., S.-H. Hosseinlar, and M.K. Heshmati. 2018c. Multi-stage continuous and intermittent microwave drying of quince fruit coupled with osmotic dehydration and low temperature hot air drying. Innovative Food Science & Emerging Technologies. 45:132–151. doi: 10.1016/j.ifset.2017.10.007.
- Dehghannya, J., E.-A. Naghavi, and B. Ghanbarzadeh. 2016. Frying of potato strips pretreated by ultrasound-assisted air-drying. Journal of Food Processing and Preservation 40(4):583–592. doi: 10.1111/jfpp.12636.
- Deng, Y., and Y. Zhao. 2008. Effect of pulsed vacuum and ultrasound osmopretreatments on glass transition temperature, texture, microstructure and calcium penetration of dried apples (Fuji). LWT - Food Science and Technology 41(9):1575–1585. doi: 10.1016/j.lwt.2007.10.018.
- Fang, S., Z. Wang, X. Hu, H. Li, W. Long, and R. Wang. 2010. Shrinkage and quality characteristics of whole fruit of Chinese jujube (Zizyphus jujuba Miller) in microwave drying. International Journal of Food Science & Technology 45(12):2463–2469. doi: 10.1111/j.1365-2621.2010.02367.x.
- Fernandes, F.A., F.E. Linhares Jr., and S. Rodrigues. 2008. Ultrasound as pre-treatment for drying of pineapple. Ultrasonics Sonochemistry 15(6):1049–1054. doi: 10.1016/j.ultsonch.2008.03.009.
- Fernandes, F.A.N., and S. Rodrigues. 2007. Ultrasound as pre-treatment for drying of fruits: Dehydration of banana. Journal of Food Engineering 82(2):261–267. doi: 10.1016/j.jfoodeng.2007.02.032.
- Horuz, E., and M. Maskan. 2015. Hot air and microwave drying of pomegranate (Punica granatum L.) arils. Journal of Food Science and Technology 52(1):285–293. doi: 10.1007/s13197-013-1032-9.
- Kesbi, O.M., M. Sadeghi, and S.A. Mireei. 2016. Quality assessment and modeling of microwave-convective drying of lemon slices. Engineering in Agriculture, Environment and Food 9(3):216–223. doi: 10.1016/j.eaef.2015.12.003.
- Koc, B., I. Eren, and F.K. Ertekin. 2008. Modelling bulk density, porosity and shrinkage of quince during drying: The effect of drying method. Journal of Food Engineering 85(3):340–349. doi: 10.1016/j.jfoodeng.2007.07.030.
- Kumar, C., M.U.H. Joardder, T.W. Farrell, G.J. Millar, and M.A. Karim. 2015. Mathematical model for intermittent microwave convective drying of food materials. Drying Technology 34(8):962–973. doi: 10.1080/07373937.2015.1087408.
- Kumar, C., M.A. Karim, and M.U.H. Joardder. 2014. Intermittent drying of food products: A critical review. Journal of Food Engineering. 121:48–57. doi: 10.1016/j.jfoodeng.2013.08.014.
- Mothibe, K.J., M. Zhang, A.S. Mujumdar, Y.C. Wang, and X. Cheng. 2014. Effects of ultrasound and microwave pretreatments of apple before spouted bed drying on rate of dehydration and physical properties. Drying Technology 32:1848–1856.
- Nowacka, M., A. Wiktor, M. Śledź, N. Jurek, and D. Witrowa-Rajchert. 2012. Drying of ultrasound pretreated apple and its selected physical properties. Journal of Food Engineering 113(3):427–433. doi: 10.1016/j.jfoodeng.2012.06.013.
- Rodríguez, Ó., J.V. Santacatalina, S. Simal, J.V. Garcia-Perez, A. Femenia, and C. Rosselló. 2014. Influence of power ultrasound application on drying kinetics of apple and its antioxidant and microstructural properties. Journal of Food Engineering. 129:21–29. doi: 10.1016/j.jfoodeng.2014.01.001.
- Rodriguez-Ramirez, J., L. Mendez-Lagunas, A. Lopez-Ortiz, and S.S. Torres. 2012. True density and apparent density during the drying process for vegetables and fruits: A review. Journal of Food Science 77(12):R146–154. doi: 10.1111/j.1750-3841.2012.02990.x.
- Roueita, G., M. Hojjati, and M. Noshad. 2020. Study of physicochemical properties of dried kiwifruits using the natural hypertonic solution in ultrasound-assisted osmotic dehydration as pretreatment. International Journal of Fruit Science. 1–17. doi: 10.1080/15538362.2020.1741057.
- Singh, R.P., and D.R. Heldman. 2014. Introduction to Food Engineering. 5th ed. Academic Press (Elsevier Inc.), San Diego.
- Tao, Y., and D.W. Sun. 2015. Enhancement of food processes by ultrasound: A review. Critical Reviews in Food Science and Nutrition 55(4):570–594. doi: 10.1080/10408398.2012.667849.
- Tsuruta, T., H. Tanigawa, and H. Sashi. 2015. Study on shrinkage deformation of food in microwave–vacuum drying. Drying Technology 33(15–16):1830–1836. doi: 10.1080/07373937.2015.1036286.
- Ullah, F., K. Min, M.K. Khattak, S. Wahab, N. Wahab, M. Ameen, M.S. Memon, X. Wang, S.A. Soomro, K. Yousaf, et al. 2018. Effects of different drying methods on some physical and chemical properties of loquat (Eriobotrya japonica) fruits. International Journal of Fruit Science 18(4):345–354. doi: 10.1080/15538362.2018.1435330.
- Yan, Z., M.J. Sousa-Gallagher, and F.A.R. Oliveira. 2008. Shrinkage and porosity of banana, pineapple, and mango slices during air-drying. Journal of Food Engineering 84(3):430–440. doi: 10.1016/j.jfoodeng.2007.06.004.
- Zhao, D., K. An, S. Ding, L. Liu, Z. Xu, and Z. Wang. 2014. Two-stage intermittent microwave coupled with hot-air drying of carrot slices: Drying kinetics and physical quality. Food and Bioprocess Technology 7(8):2308–2318. doi: 10.1007/s11947-014-1274-1.
- Zlatanović, I., M. Komatina, and D. Antonijević. 2013. Low-temperature convective drying of apple cubes. Applied Thermal Engineering 53(1):114–123. doi: 10.1016/j.applthermaleng.2013.01.012.