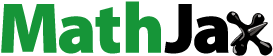
ABSTRACT
The vibration that occurs in vehicles during road transport has an important effect on the damage experienced by agricultural products, such as fruit. Young’s modulus and Poisson’s coefficient are critical mechanical properties of fruit, and alterations may indicate damage during transportation. This work studied the effect of vibration on mechanical damage in avocados (Persea americana Mill. cv. Hass) during a road trip of over 25 km in distance between the farm to avocado packing house. Chemical and mechanical properties were evaluated during twelve postharvest days at 23°C and 65% relative humidity. Once avocadoes reached full maturity, sensory tests were performed according to Colombian Technical Standards NTC 5328 and NTC 3932. Results obtained from field data are presented as power spectral density (PSD) functions to facilitate the interpretation of the exposure of produce to vibration energy during transportation. The highest intensity of these vibrations was recorded at a frequency of 2.5 Hz for frequency intervals of 0 Hz–5 Hz at the rear of the vehicle. The chemical analysis, includingmoisture content, protein, ash and ethereal extract, showed no statistically significant differences (p > .05) between the protected fruit (PF) and unprotected fruit (UF). The mechanical damage demonstrated a statistically significant difference (p < .05) in Young’s modulus between the PF and the UF. Sensory analysis also showed that the mechanical damage caused by the vibration levels affected the integrity of lenticels by means of the external appearance and pulp color descriptors of the fruit, which contributed to the subsequent darkening of the skin and the softening of the fruit.
Introduction
Postharvest of horticultural products consists of several stages including multiple instances of handling and transportation which are considered important in maintaining the physical integrity and quality of the food products (Fabela et al., Citation2002; Springael et al., Citation2018) during the distribution throughout the supply chain, from farms to consumers. Transportation is necessary, but the vibration generated by vehicles on different types of roads is one of the main causes of critical and superficial damage to the fruit (Eissa et al., Citation2012; Opara et al., Citation2016). As a result, interest has grown in the literature regarding the mechanical damage that occurs in different fruit species during transportation, such as apples (Springael et al., Citation2018), pears (Berardinelli et al., Citation2005), kiwi (Tabatabaekoloor et al., Citation2013), and tomatoes (Wu and Wang, Citation2014). According to Barchi et al. (Citation2002) mechanical damages are responsible for the quality spoilage of fresh fruit and Opara et al. (Citation2016) estimated 40% of fruits are rejected by buyers due to these damages.
Hinsch et al. (Citation1993) studied the vibration of cherries, nectarines, and pears in semi-trailers with steel spring suspension systems (Hinsch et al., Citation1993). The authors reported that highest levels of power spectral density (PSD) occurred in the rear of the trailer at frequencies between 3.5 Hz and 25 Hz. The vertical acceleration was considerably greater than the horizontal acceleration with similar observations reported by Singh and Marcondes (Citation1992). Similar results were reported when identifying more severe damage at frequencies of 3.5 and 18.5 Hz during transportation of Bartlett pears (Slaughter D. C. et al., Citation2013).
Considering these studies, the transportation of agricultural products is a well-established potential source of damage, which is directly related to the type of road, its roughness, the distance traveled, the speed of displacement, the suspension type of the truck, the load, and the number of axles (Berardinelli et al., Citation2005; Opara et al., Citation2016). In addition, the extent of fruit damage can be influenced by the products’ intrinsic characteristics and the energy exerted on it during transportation (Vursavus and Ozguven, Citation2004). The energy transmitted depends on the properties of the products, such as maturity or ripening stage of the fruit, as well as the packaging and storage conditions (Fernando et al., Citation2018; Zhang et al., Citation2017).
Intensity of the vibrations that appear during the transportation in vehicles is complex and plays a significant role in the level of damage experienced by fruits and vegetables in the distribution chain (Ranathunga et al., Citation2010). Therefore, the vibration measurement procedure depends on the nature and complexity of the process. Although the vertical vibrations in vehicles can be easily measured, the variability of the process and the lack of control over measured parameters makes it difficult, if not impossible, to obtain records that are typical of the vibrations produced by vehicles on all roads (García- Romeu, Citation2007).
The avocado trade has gained participation in both the Colombian internal market and the international market (Granados and Giraldo, Citation2011), causing an increase in the need to transport product over long distances, and at the same time, an elevation of consumer expectations regarding product quality. Consequently, there is a need to minimize the quality deterioration and waste caused during road transport (Whiley, et al., Citation2013; Woolf et al., Citation2009). Avocados are very popular in the worldwide market due to their taste, consistency, high nutritional value, and wide applications, not only in the food industry but also in the cosmetology and pharmaceutical industries (Bermeo et al., Citation2012, Granados Cortes and Giraldo, Citation2011; Bernal, Citation2016).
In recent years, avocado is one of the most dynamic fruits in the Colombian economy, being the third most important product in the national fruit sector (Granados and Giraldo, Bernal, Citation2016; Granados Cortes and Giraldo, Citation2011). As evidence of this, 148,000 tons of Hass avocado in 2018 were produced in the Country, of which 41,353 tons were exported, i.e. 48.5% more than in the same period of previous year (MADR, Citation2018). On the other hand, only about one-eighth of the road system is paved in Colombia, and 33% of the road system consists of unpaved tertiary roads (DANE, Citation2014). Therefore, moving this volume of fruit through the Colombian road system implies the mechanical damage to be a recurring problem present in the whole avocado supply chain. According to the Health and Social Protection Ministry and the Food and Agricultural Organization (FAO), postharvest losses of fruits in Colombia were estimated to be 33% of the total production, which amounts to 1,154,923 tons (DNP, Citation2016).
In Colombia, existent research advances and technological developments at the national level, have not been sufficient to respond to the production needs or to further exploit the commercial potential of this fruit (Bernal, Citation2016). Therefore, it is necessary to increase the understanding of postharvest quality management in avocados, especially on the vibration effects during transportation by road in the Colombian structurally deficient road system.
Measurement and analysis of vibration levels during transport is essential to improving the fruits’ quality. Therefore, the objectives of the present study are to: (a) measure and analyze the distribution of vibration levels during avocado transportation and (b) analyze the effects generated by the vibration levels on mechanical, chemical, and sensory properties of avocados.
Materials and methods
Fruit material
The fruit material was collected from adult avocado (cv. Hass) trees, aged between 5 and 6 years, from an experimental field located in La Ceja – Antioquia, Colombia. Avocado fruits were harvested at grade 1 on the maturation scale (White et al., Citation2009) when the average temperature was 20 ± 2°C and the average a relative humidity was 70 ± 2 %. The samples were harvested on December 2018 and March 2019, corresponding with the main production peaks in Antioquia, when the good development of flowering and fruit formation is a consequence of the favorable phenological and environmental conditions. To avoid damages in the harvest and collection three random cutting operators were selected to take care of the fruit of mechanical damage in the area of the peduncle, cuts caused by harvesting hook or broken plastic boxes and injury caused by striking or compressing any part of the fruit.
Later, the fruits were inspected and classified as protected fruit (PF) and unprotected fruit (UP). Protected fruit was individually covered with a polyethylene foam mesh and stored in plastic baskets equally coated with the same material. In addition, plastic baskets with PF were transported separately. Unprotected fruit was exposed to real transportation conditions, i.e. in plastic baskets without any protection, and therefore experienced impact, compression, and shear stresses. shows the harvest and transport conditions mentioned.
Vehicle
The vehicle used to measure and analyze vibration levels during transportation was a Mitsubishi L200 4 × 4 equipped with a suspension of semi-elliptical laminated springs with shock absorbers as shown in . Each trip covered a route of approximately 25 km (15.5 miles) in distance with a 50 minutes average duration on tertiary roads from the experimental field to the avocado packing house, in the municipality of La Ceja, Antioquia. As mentioned, the roads were of tertiary type, i.e. roads predominately unpaved and single lane, usually used as access roads that connect the townships with their rural settlements.
Experimental design
The experiment was established according to a completely randomized design with multiple variables and outcomes. In each trip, two baskets of PF and two UF baskets were randomly selected in the avocado packing facility. In total, 10 trips during the peak of harvest during December 2018 and March 2019 were performed.
The Experimental Units (EU) were selected randomly from each avocado basket. For chemical and mechanical analysis 180 EU were used. For vibration damage evaluation and sensory analysis 180 EU and 60 EU were used, respectively. In addition, EU were stored at a constant average temperature of 23 ± 2°C and an average relative humidity of 65 ± 2% during the 0–12 days postharvest evaluation. shows the EU quantity according to test type. Results were statistically analyzed by Analysis of Variance (ANOVA) and Turkey´s Test method with Python Software version 3.6.
Data analysis and determination of vibration levels
For determination of vibration levels, Netux® technology was used. The Netux® set up included an accelerometer ADXL 345 (with feature sensitivity ± 16 G and output data rates of 3200 Hz), a conditioner (amplifier), and a MicroSD (8 G) data acquisition system. The accelerometer was set-up to continuously sample at 1.000 Hz and was coupled with an external battery pack to enable continuous sampling during each tri The Netux® device was randomly installed in one of the baskets to measure the vibration of vehicle. A computer controlled the signal and directed it to cloud storage.
Time series data from each replicate were obtained and the longitudinal (X-axis), vertical (Y-axis) and lateral (Z-axis) directions were averaged. In addition, the development of power spectral density (PSD) profiles based on the vibration levels was essential to understanding the exposure of sample to vibration energy in a given transit route (Fernando et al., Citation2019; Singh et al., Citation2006). Time series vibration data was converted to frequency domain by Fast-Fourier Transform (FFT) using the signal processing toolbox in MATLAB® version 2018a (Mathworks, Natick, MA, USA) (Fernando et al., Citation2019). Furthermore, a code generated in the software cleaned outliers and performed the overlap signal deconvolution, generating the best adjustment of the PSD function. The PSD peaks were present in the lower frequency range (0–5 Hz). Preview tests showed that after 5 Hz no significant PSD peaks were observed. Hereby, the frequencies between 0 and 5 Hz were selected. The Power spectral density of a given vibration signal within a band of frequencies was derived through the EquationEq. 1(1)
(1) and EquationEq. 2
(2)
(2) (García-Romeu, Citation2008).
where “n” is the number of samples used, Gf,i is the acceleration spectrum module in “g” associated with a given frequency of the spectrum “f” and a given event or record “i,” “n” is the number of events or records selected (the maximum power of two within the smaller of the vectors obtained), “FFT size” is the number of instants sampled for a given record or event, “Δf = (sampling frequency) • (FFTsize)−1 ” is the resolution of the frequency spectrum, and “ai,t” is the acceleration recorded in “g” for each event or record determined “i” in time “t” (García-Romeu, Citation2008).
Chemical characterization
Chemical characterization of avocados was carried out at 0, 6, and 12 postharvest days. For each of the chemical the analyses (humidity, protein, ash and ethereal extract) a total of 180 EU, 90 corresponding to PF and the same amount for UF, obtained from 10 trips were selected. The total EU were equally distributed for the analyses at each established postharvest day (0, 6, and 12), resulting in a 30 EU sample size for each treatment. The methodology for each of the tests is described in .
Table 1. Methodologies for chemical analysis
Evaluation of vibration damage to avocados and sensory analysis
Vibration damage during transportation may not become noticeable for several days. Flesh defects are often not visible externally, especially for avocado fruits. Mechanical damage during postharvest may result in skin spotting; this defect is also called lenticel damage, which has a significant impact on quality sensory descriptors, such as external appearance and pulp color (Whiley, et al., Citation2013; White, et al., Citation2009). Vibration damage was evaluated by quality descriptors and mechanical properties (Everett et al., Citation2007; Shahbazi et al., Citation2010; White, et al., Citation2009).
In this study, avocado vibration damage was identified by inspecting each of the 180 EU for the presence of a dark and elongated coloration in the epidermis (skin) and mesocarp (pulp). Given that avocado fruits do not show visible bruising until they start softening in the ripening process (Mazhar et al., Citation2015), the evaluation consisted of counting the external and pulp damages in each avocado once it had reached consumption maturity (i.e. 12 postharvest days) (Herrera et al., Citation2017). Besides, the Quality Index (QI) and Acceptance Index (AI) were calculated with EquationEq. 3(3)
(3) and EquationEq. 4
(4)
(4) , respectively, according to the Colombian Technical Standards NTC 3932. In EquationEq. 3
(3)
(3) and EquationEq. 4
(4)
(4) , WD is the quantity of fruit units without defects; RS is the quantity of fruit units rejected; and TS is the total quantity of EU evaluated.
Sensory analysis was conducted at consumption maturity (12 days postharvest) for 60 EU (30 for PF and 30 for UF). For the control group, 30 EU of PF that were not subjected to vibration during transportation were selected. The sensory analysis was carried out by eight trained judges, following the Colombian Technical Standards NTC 4129 and Colombian Technical Standards NTC 4130. A quantitative response scale of 7 points (0 = absent, 1 and 2 = low, 3 = medium-low, 4 = medium, 5 = medium-high, 6 and 7 = high intensity) was used according to Colombian Technical Standards NTC 3932 and Colombian Technical Standards NTC 5328. The sensory descriptors evaluated were external appearance, pulp color, characteristic smell, objectionable odor, characteristic flavor, bitter taste, objectionable taste, hardness, and fat feeling.
Determination of mechanical properties
Uniaxial compression tests were applied to determine the mechanical properties of Poisson’s ratio, strain (mm mm−1) and elastic modulus (MPa) (Bourne, Citation2002; Rosenthal, Citation1999). The mechanical properties tests of avocados were carried out for both PF and UF, at 0, 6, and 12 postharvest days, with a sample size of 30 EU for each treatment. Consequently, a total of 180 EU from 10 trips were selected; 90 EU corresponding to PF and the same amount for UF. The texture analyzer TA-XTPlus Stable Micro Systems® and Texture Exponent 32 Software, Version 6.1.4.0® was used, with an operating speed of 1 mm s−1 and compression plate of 75 mm (ASAE, Citation2005; Ochoa et al., Citation2009; Sharma et al., Citation2003). Uniaxial compression tests were carried out on cylindrical samples of avocado fruits, 16 mm in diameter by 12 mm in height. Three cylindrical samples of each EU were obtained using a borer and a sharp knife, from opposite sides of an equatorial area in each fruit (Ciro et al., Citation2005; Pérez-López et al., Citation2014). The indicated sample size was selected such that the height was equal to or less than its diameter in order to prevent the compression test results from being less reproducible and unreliable due to strain (Pérez-López et al., Citation2014; Shaw and Young, Citation1988).
Poisson’s ratio
Poisson’s ratio is the absolute value of the ratio of lateral strain to the corresponding axial strain resulting from uniformly distributed axial stress below the proportional limit of the material. This ratio will have more than one value if the material is anisotropic (Abbott and Lu, Citation1996; Mohsenin, Citation1986; Popov, Citation2000). The lateral strain and the axial strain were taken directly from the Texture Exponent 32 Software, Version 6.1.4.0® and recorded by means of a video capture and synchronization system from Stable Micro Systems®. The images from the videos were processed with the software Windows Movie Maker® with precision in the measurements of ± 0.1 mm/mm. Data obtained were reported as the arithmetic average for 0, 6, and 9 postharvest days; Poisson’s ratios were calculated from EquationEq. 5(5)
(5) (Popov, Citation2000):
where εl is the lateral strain, and εa is the axial strain.
Elastic modulus and strain
Through preliminary compression tests and the use of stress-strain graphs, the compression distance of the sample was determined (ASAE Standards, Citation2005). The modulus of elasticity was determined by EquationEq. 6(6)
(6) (Mohsenin, Citation1986; Popov, Citation2000):
where is the elastic modulus (MPa); F is the force (N); A is the original cross-sectional area (mm2); ΔL (mm) is the deformation corresponding to force F; L is the initial length;
is the normal stress (MPa); and
is the normal strain (mm mm−1).
The stress-strain graphs were recorded by the Texture Exponent 32 Software, Version 6.1.4.0®. The “real or true” condition relationships given by Mohsenin (Citation1986) and Popov (Citation2000) were determined by EquationEq. 7(7)
(7) and EquationEq. 8
(8)
(8) :
For true stress,
and for true strain,
where is the true stress (MPa); F is the applied force (N); A(t) is the original cross-sectional area (mm2); and
is the true strain (mm mm−1). The mechanical properties mentioned were obtained from the linear region of the stress–strain curve (Mohsenin, Citation1986).
Results and discussion
Analysis of the vibration levels
Acceleration in the lateral (Z-axis), longitudinal (X-axis), and vertical (Y-axis) axes are shown in , which shows that the magnitude of the vibration spectrum in the vertical direction is greater compared to the other directions (lateral and longitudinal). Longitudinal acceleration was present at a low intensity throughout the trip. Lateral acceleration had its highest intensity in the low frequency range of 1.0–1.5 Hz, and vertical acceleration had PSD peak at 2.5 Hz to low frequency range of 1.5–5.0 Hz. Similarly, Piercer et al. (Citation1992) found higher levels of vibration under mechanical conditions at 4.0 Hz in the vertical direction.
Figure 4. Power spectral density (PSD) for the longitudinal (X-axis), vertical (Y-axis), and lateral (Z-axis) directions
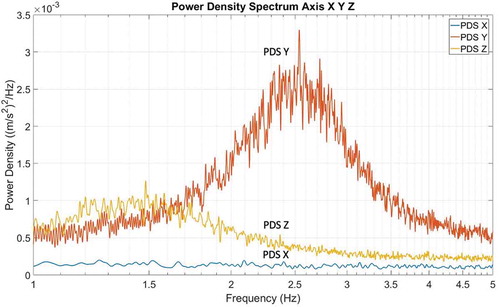
Similar studies were carried out during the transportation of pears, which reported peaks between 2.5 and 4.0 Hz on the platform of the truck (Jarimopas et al., Citation2005; Zhou et al., Citation2007). Similarly, Shahbazi (Citation2010) found peaks between 5–10 Hz and 10–15 Hz during the transportation of watermelons for two positions on the platform of the truck. During the transportation of apples, peaks between 1.0 and 5.0 Hz for the front of the transporter truck were recorded (Singh and Xu, Citation1993; Timm et al., Citation1996). Comparable results were also obtained by in Hinsch et al. (Citation1993) and Slaughter et al. (Citation2013), where the vibration on pear baskets did not exceed 3.5 Hz.
Chemical analysis
shows the components of the chemical analysis performed on the fresh avocado pulp for both the PF and UF at 0, 6, and 12 postharvest days. Significant differences were not found (p > .05) for PF and UF on postharvest days, indicating that, changes in chemical properties caused by the vibration in the low frequency range (0–5 Hz) were not observed between PF or UF in each of the analyzed postharvest days.
Table 2. Chemical composition of hass avocados
On the other hand, PF and UF results on day 0 were similar to those reported by Ortega (Citation2003), where humidity and protein were 75% and 1.7%, respectively. On day 12, the results were similar to those reported in Serpa et al. (Citation2014), where humidity reached 68.69%. On postharvest day 12, the oil content increased from 17.66% to 21.83% and 20.56% for the PF and UF, respectively. These results coincide with those reported by Serpa, (Citation2014) and Bernal (Citation2016) who determined oil contents to be 21.62% and 21.98%, respectively for the same postharvest day. These results can be attributed to a displacement of water molecules by oil inclusions outside the cellular vacuoles, thereby contributing to the reduction in moisture content (Davenport and Ellis, Citation2016). However, it is important to highlight that the climatic diversity of avocado (cv. Hass) producing regions in Antioquia affect fruit production and quality (Lobell et al., Citation2007); therefore, the chemical properties also vary according to the microregions (Izhaki et al., Citation2002).
In addition, the PF and UF had a maturation time of 12 days, with Dry Matter content (DM) increasing from 26.87% to 31.37% and 31.96%, respectively. Similar results were reported by Bernal (Citation2016), where dry matter content increased from 26.5% to 30.5% for a duration of 12.5 days. Additionally, oil content results were 10.64 units less than the DM. Similar results were reported by Whiley, et al. (Citation2013) where the oil content is approximately 11 units less than the DM. However, statistically significant differences (p > .05) were not observed between the oil content and dry matter results from PF and UF, for the peaks determined in the frequency range of 0.1–5 Hz during transportation on tertiary roads of Colombia.
Our results indicate that, as reported (Lee et al., Citation1983; Ranney, Citation1991), DM is strongly related to oil content. Given the DM test is considerably faster than the humidity test, and less expensive than oil content tests, DM could be a simplest, faster method to evaluate the maturity of the fruit, when compared to the more traditional methods such as humidity or oil content. In this sense, DM could become a low-technology alternative standard for determining avocado maturity within the industry in Colombia and other developing countries. For instance, countries such as Australia and Mexico have established a minimum DM value for harvest of 21% and 23%, respectively (Whiley, et al., Citation2013). In Colombia, this standard has yet to be created and currently, the harvest time is determined only with subjective criteria, based mainly on the experience of the harvesters and other aspects such as the time of year, color changes and fruit brightness (Bernal, Citation2016).
Evaluation of vibration damage to avocados and sensory analysis
Resulting vibration damage in the 12 postharvest day was revealed by dark brown-black damage areas in the epidermis (skin) and the mesocarp (pulp). This defect has a significant impact on the quality sensory descriptors (Whiley, et al., Citation2013; White, et al., Citation2009). presents the results of the vibration damage to avocados. The darkening of the skin and pulp were the main injuries to the fruit during transportation. The skin and pulp damage had statistically significant different results (p < .05) for the PF and UF. Mechanical damage in UF was more influenced than PF by the vibrations in low frequency range (0–5 Hz), causing a decrease in the internal and external quality. The results agree with those reported for Herrera et al. (Citation2017), where mechanical damages were estimated to damage 4.7% of the fruit in the trip from the harvest site to the packing facilities. Similar results were reported by other crops such as mango (Mazhar et al., Citation2011) and orange (Miranda et al., Citation2015), where mechanical damages are considered as a type of stress, consequence of an inappropriate harvest, handling, and transportation techniques of the fruit during the harvest and postharvest. In addition, these authors point out that the most common mechanical damages are cuts, tearing and bruising caused by impacts, compressions and vibrations.
Table 3. Evaluating vibration damage of hass avocados
In this study, only 3% of the avocados were rejected, both for PF and UF. However, in terms quality index, PF had a significantly statistic (p < .05) higher assessment than UF, at 84% and 66% respectively. Likewise, PF had less bruising in the pulp (4.62 ± 0.36, compared to 5.88 ± 0.16 for UF) and skin (4.94 ± 0.36, compared to 5.96 ± 0.22 for UF) than UF.
shows the effects of mechanical damage to avocados, which manifested as dark brown spots on the epidermis (skin) and mesocarp (pulp).
Sensory analysis results did not show significant differences (p > .05) for the PF and UF in the descriptors of appearance, pulp color, characteristic smell, characteristic flavor, hardness and fat feeling. The descriptors of objectionable odor, bitter taste, and objectionable taste were absent during the sensory evaluation, because the immature fruits were rejected before sensory analysis. Similar results were reported in Bernal (Citation2016), where the judges issued favorable scores for the appearance of the pulp and the taste of the harvested fruit. presents the results of the sensory evaluation in fresh Hass avocado fruits at twelve days postharvest.
Mechanical damages generated for vibration levels were more prevalent in UF than PF, decreasing the internal and external fruit quality. In addition, avocados with vascular obscuration influenced sensory analysis descriptors, such as external appearance, pulp color and characteristic flavor. Furthermore, the sensory analysis results were similar to those obtained by Singh and Xu (Citation1993), Hinsch et al. (Citation1993), Slaughter et al. (Citation2013), Jarimopas et al. (Citation2005), Zhou et al., (Citation2007), and Shahbazi et al. (Citation2010).
Mechanical properties
Poisson’s ratio
An increase in Poisson’s ratio was observed as the postharvest days increased, due to the ripening of the avocados, from 0.30 on 0 days to 0.42 on 9 days for the PF, and 0.33 on 0 days to 0.38 on 9 days for the UF (see ). Results indicated that the number of days postharvest of the avocado significantly influenced the Poisson’s ratio of the avocado. However, statistically significant differences (p > .05) were not observed in Poisson’s ratio results between the PF and UF for postharvest days evaluated. Therefore, Poisson’s ratio properties were not affected by vibrations generated in the low frequency range (0–5 Hz) of the spectrum during transportation by tertiary roads of Colombia.
Table 4. Poisson’s ratio
A greater lateral strain compared to the same axial strain decrease was observed for both PF and UF during each postharvest day. In addition, PF showed greater resistance to change in the longitudinal direction by perpendicular compression. On the other hand, UF presented a low resistance to compression compared to PF. Consequently, UF were exposed to greater mechanical damages with respect to PF.
Advances in maturity and postharvest days resulted in higher Poisson’s ratios in avocados. On the other hand, the avocado also demonstrated its anisotropic behavior, which may be attributed to the shape and arrangement of parenchymal cells and other morphological components (Khan and Vincent, Citation1990; Kays, S.J., Paull, R.E., Citation2004. Postharvest Biology. Exxon Press, Athens, Georgia).
Analysis of elastic modulus and strain
Through preliminary compression tests and the use of stress-strain graphs, the compression distance of the sample and the point of failure was determined. The relationship between stress and strain in true criteria was approximated to the linear regression model represented by EquationEq. 4(4)
(4) . The modulus of elasticity indicates the stiffness of the product in a fixed load direction.
shows a decrease in the modulus of elasticity as the postharvest days increased. Elasticity results decreased from 3.845 MPa at 0 days to 0.352 MPa at 12 days for the PF and 3.677 MPa at 0 days to 0.298 MPa at 12 days for UF, because the material’s resistance decreased with maturity. As the rigidity of the material decreases, its ability to deform increases. Similar results were reported in Keitt and Tommy Atkins mechanical harvest studies, where the elastic modulus also decreased from 2.065 MPa to 1.381 MPa and 1.698 MPa to 1.518 MPa, respectively (Hernández et al., Citation2013). Pérez-López et al., (Citation2014) reported elastic modulus values in peach fruits that decreased from 0.789 MPa to 0.393 MPa.
Table 5. Elastic modulus and strain
An increase in the strain values as the postharvest days and maturity increased is shown in . The decrease in stiffness of the avocado during maturation depends largely on the extent to which the structural changes occur in the cell wall constituents. In a previous study with peach fruits, the strain values increased from 0.335 mm mm−1 to 0.370 mm mm−1 (Pérez-López et al., Citation2014).
Results of elastic modulus and strain presented statistically significant differences (p > .05) between the PF and UF for the evaluated postharvest days. Elastic modulus and strain properties for UF were affected by generated vibrations in the low frequency range (0–5 Hz) of the spectrum. These results suggest that UF it is more fragile during transportation due to vibrations.
This study confirmed that low frequency vibrations (0–5 Hz) caused irreversible damages on the elastic modulus and strain of avocados. In addition, vibration detectable as PSD peaks in the lower range of the spectrum, is essential for causing mechanical damages to fruit. In addition, provides a comprehensive approach to study the vibration levels generated during transportation of fruit by unpaved gravel roads in a rural setting, combined with an effort to correlate avocado damage with said vibration. However, a limitation of this study is that some other potential factors and variables were not tested: the motion of the vehicle is dependent on many features like the road profile (paved, unpaved, single-lane, etc), vehicle characteristics (speed, vehicle axle and wheel type, suspension, tires and viscous damper position on the vehicle) as well as the load (Van Zeebroeck et al., Citation2006). Future work could attempt to study the incidence of these factors in the damage by vibration on fruit.
Conclusions
The vibration levels that occurred in the vehicle and road used in this investigation varied in its lateral and longitudinal coordinates and, at the same time, were minor in comparison with the vertical vibrations, where the greatest intensity was presented. During road trips of 25 km and 50 min, Hass avocados were exposed to vibrations generated in the low frequency range (0–5 Hz). In this frequency range, a PSD peak in 2.5 Hz was observed, altering the quality of the fruits in consumption maturity by causing dark brown-black bruises in skin and pul Chemical properties in Hass avocado, forboth protected fruit (PF) and the unprotected fruit (UF), did not change by exposed to vibrations during the trips. Mechanical damages generated by vibration levels were more representative in UF than PF, decreasing the internal and external fruit quality. In addition, avocados with vascular obscuration influenced sensory analysis descriptors, such as external appearance, pulp color and characteristic flavor. Mechanical properties on UF were affected by vibrations i.e. UF structure was more fragile. However, Poisson’s ratio was not affected by vibrations in the low frequency range, confirming the known avocado’s isotropic behavior. Future research needs to focus on minimizing mechanical injuries and improving the fruit quality in postharvest supply chain, by the evaluation of longer trips and the assesstment of the effectiveness of vibration isolation mechanisms, such as improved packaging alternatives for the transport of avocado, such as foam mesh polymer-based, to minimize the vibration energy transmission from the vehicle floor to the plastic baskets.
Highlights
The energy exerted by vibration during transportation is one of the main causes internal and superficial damage in the avocado.
The magnitude of vibration in the vertical direction are greater compared to the lateral and longitudinal vibration spectrum.
The vertical acceleration had their highest PSD peak in 2.5 Hz to low-frequency range of 1.5–5.0 Hz.
The protected fruit presented higher Quality Index then unprotected fruit, at 84% and 66% respectively.
Acknowledgments
The authors acknowledge the Ministry of Science, Technology and Innovation of the Colombian Government (Minciencias) for the national doctorates 727 of 2015 and the Research Center for Investigation and Development (CIDI) from the Universidad Pontificia Bolivariana for their financial support. The authors are also grateful to the Faculty of Agricultural Sciences of the Universidad Nacional de Colombia - Medellín for their invaluable assistance.
Additional information
Funding
References
- Abbott, J.A., and R. Lu. 1996. Anisotropic mechanical properties of apples. En: Trans. ASAE. 39:1451–1459.
- AOAC. 1990. Official methods of analysis. 17th ed. Association of Official Analytical Chemists, Washington, DC.
- ASAE Standards. 2005. Compression test of food material of convex shape. american society for agricultural engineering. St.Joseph, Michigan. S368.4.
- Barchi, G.L., A. Berardinelli, A. Guarnieri, L. Ragni, and C.T. Fila. 2002. Damage to loquats by vibration-simulating intra-state transport. Biosyst. Eng. 82(3):305–312. doi: 10.1006/bioe.2002.0067.
- Berardinelli, A., V. Donati, A. Giunchi, A. Guarnieri, and L. Ragni. 2005. Damage to pears caused by simulated transport. J. Food Eng. 66(2):219–226. doi: 10.1016/j.jfoodeng.2004.03.009.
- Bermeo, H. Moreno Carolina and Sandoval Angélica. 2012. Prospectiva para la innovación en la agrocadena del aguacate en el Tolima.Universidad de Ibagué, Ibagué, Colombia. 107 p.
- Bernal, J. 2016. Estudios ecofisiológicos en aguacate cv. Hass en diferentes ambientes como alternativa productiva en Colombia. Universidad Nacional de Colombia. 296 p.
- Bourne, M.C. 2002. Food texture and viscosity: concept and measurement. food science and technology. 2nd ed. Academic Press, New York, NY.
- Ciro, J., D. Vahos, and C. Márquez. 2005. Estudio experimental de la fuerza de fractura en frutos tropicales: El tomate de árbol (Cyphomandra betacea Sendt). En: DYNA. 72(146):55–64.
- Colombian Technical Standards NTC 3932. 1996. Sensory analysis. Identification and selection of descriptors for establishing a sensory profile by a multidimensional approach. ICONTEC. Bogotá, D.C.
- Colombian Technical Standards NTC 4130. 1997. Sensory analysis. General guide for the selection, training and monitoring of judges. Part 1. ICONTEC. Bogotá, D.C.
- Colombian Technical Standards NTC 5328. 2004. Sensory analysis. Guidelines for the use of quantitative response scales. ICONTEC. Bogotá, D.C.
- DANE-Departamento Administrativo Nacional de Estadística. 2014. Infraestructura Vial. Documento técnico. Bogota, D.C.
- Davenport, J.B., and S.C. Ellis. 2016. Chemical changes during growth and storage of the avocado fruit. Austral. J. Biol. Sci. 12:445–454. doi: 10.1071/BI9590445.
- DNP - Departamento Nacional de Planeación. 2016. Pérdida y Desperdicio de Alimentos en Colombia. Estudio de la Dirección de Seguimiento y Evaluación de Políticas Públicas. Bogotá; D. C.
- Eissa, A.A.H., G.R. Gamaa, F.R. Gomaa, and M.M. Azam. 2012. Comparison of package cushioning materials to protect vibration damage to golden delicious apples. Int. J. Latest Trends Agric. Food Sci. 2(1):36–57.
- Everett, K.R., I.C. Hallett, R.W. Chynoweth, and H.A. Pak. 2007. Avocado lenticel damage: The cause and the effect on fruit quality. Posharvest Biol. Technol. 48:383–390. doi: 10.1016/j.postharvbio.2007.09.008.
- Fabela, M. de Jesús, Hernández, J., Vega, D. and Lozano, A. 2002. Vibration during transport and its effect on perishables. Introductory approach. Technical publication No.188.
- Fernando, I., J. Fei, and R. Stanley. 2019. Measurement and analysis of vibration and mechanical damage to bananas during long-distance interstate transport by multi-trailer road trains. Postharvest Biol. Technol. 158:1–10. doi: 10.1016/j.postharvbio.2019.110977.
- Fernando, I., J. Fei, R. Stanley, and H. Enshaei. 2018. Measurement and evaluation of the effect of vibration on fruits in transit. Packag. Technol. Sci. 31:723–738. doi: 10.1002/pts.2409.
- García-Romeu, M. Manuel. (2008) Contribución a la determinación y simulación de las vibraciones e impactos en operaciones de transporte y distribución de productos de consumo embalados. Universidad Politécnica de Valencia. Departamento de Proyectos de Ingeniería. https://doi.org/10.4995/Thesis/10251/2927
- Granados Cortes, H., and O. Giraldo. 2011. Prospectiva de la actividad productiva del aguacate para Colombia. Mercatec 49:p 15.
- Hernández, L.I. 2013. Mechanical behavior of two varieties of mango (Mangifera Indica) under axial compression. Rev. Cienc. Téc. Agropecuarias. 22(2):11–15.
- Herrera, J., E. González, and L. Madrigal. 2017. Proportions of mechanical damages and their effect on post-harvest quality of avocado ‘Hass’. Rev. Mex. Cienc. Agrícolas. 19:3897–3909.
- Hinsch, R.T., D.C. Slaughter, W.L. Craig, and J.F. Thompson. 1993. Vibration of fresh fruits and vegetables during refrigerated truck transport. Trans. ASAE. 36:1039–1042. doi: 10.13031/2013.28431.
- Izhaki, I., E. Tsahar, O. Paluy, and J. Friedman. 2002. Within population variation and interrelationships between morphology, nutritional content, and secondary compounds of Rhamnus alaternus fruits. New Phythol. 156(2):217–223. doi: 10.1046/j.1469-8137.2002.00515.x.
- Jarimopas, B., S. Singh, and W. Saengnil. 2005. Measurement and analysis of truck transport vibration levels and damage to packaged tangerines during transit. Packag. Technol. Sci. 18:179–188. doi: 10.1002/pts.687.
- Kays, S.J., Paull, R.E., 2004. Postharvest Biology. Exxon Press, Athens, Georgia.
- Khan, A.A., and J.F.V. Vincent. 1990. Anisotropy of apple parenchyma. J. Sci. Food Agric. 52(4):455–466. doi: 10.1002/jsfa.2740520404.
- Lee, S.K., R.E. Young, M. Schiffman, and C.W. Coggins. 1983. Maturity studies of avocado fruitt base don picking dates and dry weigth. J. Am. Soc. Hortic. Sci 108:390–394.
- Lobell, D.B., K.N. Cahill, and C.B. Field. 2007. Historical effects of temperature and precipitation on California crop yields. Clim Change. 81:187–203. doi: 10.1007/s10584-006-9141-3.
- MADR-Ministerio de Agricultura y Desarrollo Rural – MADR. 2018. Cadena de aguacate. Indicadores e Instrumentos. Bogota, D.C.
- Mazhar, M.S. 2015. Bruising in avocado (persea americana m.) cv. Hass supply chains: From the ripener to the consumer. School of Agriculture and Food Sciences. The University of Queensland, Queensland, Australia. doi:10.14264/uql.2015.782.
- Mazhar, M.S., M. Amin, A.U. Malik, J. Campbell, and Johnson. 2011. Improved harvest and desapping practices affect mango fruit quality along the supply chains. Inter. J. Agric. Biol. 13(5):776–780.
- Miranda, M., C. Spricigo, and M.D. Ferreira. 2015. Management zones using fuzzy clustering based on spatial-temporal variability of soil and corn yield. J. Brazilian Assoc. Agric. Eng. 35(7):154–162.
- Mohsenin, N. 1986. Physical properties of plant and animal materials: Structure, physical, characteristics and mechanical properties. Gordon and Breach Science Publishers, New York. 664 p.
- Colombian Technical Standards NTC 3932 (1996) Sensory analysis. Identification and selection of descriptors for establishing a sensory profile by a multidimensional approach. ICONTEC. Bogota, D.C.
- Colombian Technical Standards NTC 4129 (1997) Sensory analysis. General guide for the selection, training and monitoring of judges. Part 1. ICONTEC. Bogota, D.C
- Colombian Technical Standards NTC 4130 (1997) Sensory analysis. General guide for the selection, training and monitoring of judges. Part 2. ICONTEC. Bogota, D.C.
- Colombian Technical Standards NTC 5328 (2004) Sensory analysis. Guidelines for the use of quantitative response scales. ICONTEC. Bogota, D.C.
- Ochoa, S., Hertog, M.L., and Nicolai, B.M. 2009. Modelling the transient effect of 1-MCP on ‘Hass’ avocado softening: A Mexican comparative study. Postharvest Biol. Technol. 51:62–72. doi: 10.1016/j.postharvbio.2008.06.002.
- Opara, U.L., T. Fadiji, O. Chukwu, L. Chen, and C. Coetzee. 2016. Susceptibility of apples to bruising inside ventilated corrugated paperboard packages during simulated transport damage. Postharvest Biol. Technol. 118:111–119. doi: 10.1016/j.postharvbio.2016.04.001.
- Ortega, T.M. 2003. Valor nutrimental de la pulpa fresca de aguacate Hass. En: Consejería de Agricultura y Pesca. V Congreso Mundial del Aguacate, 19/24 de octubre de 2003, Málaga, España: Servicio de Publicaciones y Divulgación, pp. 741–748.
- Pérez-López, A., S.H. Chávez-Franco, C.A. Villaseñor-Perea, T. Espinosa-Solares, L.H. Hernández-Gómez, and C. Lobato-Calleros. 2014. Respiration rate and mechanical properties of peach fruit during storage at three maturity stages. J. Food Eng. 142:111–117. doi: 10.1016/j.jfoodeng.2014.06.007.
- Popov, E. 2000. Mecánica de materiales. Segunda edición. Pearson Educación, México. 888 p.
- Ram, Z., S. Shuqiang, L. Yan, and L. Yunfei. 2007. Effect of transport vibration levels on mechanical damage and physiological responses of Huanghua pears (Pyrus pyrifolia Nakai, cv. Huanghua). Postharvest Biology and Technology. 46, 20–28 p.
- Ranathunga, C.L., H.H.E. Jayaweera, S.K.K. Suraweera, S.C. Wattage, K.K.D.L. Ruvinda, and T.R. A. 2010. Vibration effects in vehicular road transportation. Proc. Tech. Sess. Sri Lanka 26:9–16.
- Ranney, M.S. 1991. Relationship between physiological maturity and porcent dry matter of avocados. California avocado society yearbook 75, 71–85
- Rosenthal, J.A. 1999. Food texture: measurement and perception. Aspen Publisher Inc, Gaithersburg, Maryland. ISBN: 0834212382.
- Serpa, A.M., A. Echeverri, M. Lezcano, L.M. Vélez, A.F. Ríos, and G.A. Hincapié. 2014. Extracción de aceite de aguacate variedad “Hass” (Persea americana Mill) liofilizado por prensado en frío. Rev. Invest. Apl 8:113–123.
- Shahbazi, F., A. Rajabipour, S. Mohtasebi, and S. Rafie. 2010. Simulated in-transit vibration damage to watermelons. J. Agric. Sci. Techno 12:23–34.
- Sharma, S., S. Mulvaney, and S. Risvi. 2003. Ingeniería de alimentos. Limusa Wiley, México. 348 p.
- Shaw, M.C., and E. Young. 1988. Rubber elasticity and fracture. J. Eng. Mater. Technol. 110:258. doi: 10.1115/1.3226046.
- Singh, S., and J. Marcondes (1992). Vibration levels in commercial truck shipments as a function of suspension and payload BT - vibration levels in commercial truck shipments as a function of suspension and payload.
- Singh, S., and M. Xu. 1993. Bruising in apples as a function of truck vibration and packaging. Appl. Eng. Agric. 9(5):455–460. doi: 10.13031/2013.26009.
- Singh S.P, Pierce C, Burgess G. 1992. A comparison of leaf spring to air cushion trailer suspensions in the transportation environment. International Journal of Packaging Technology and Science. 5, p. 11–15.
- Singh, S.P., G. Burgess, J. Singh, and M. Kremmer. 2006. Measurement and analysis of the net-day air shipping environment for mid-sized and lightweight packages for DHL Fedex and United Parcel Service. Packag. Technol. Sci. 19:227–235. doi: 10.1002/pts.726.
- Slaughter, D.C., R.T. Hinsch, and J.F. Thompson. 2013. Assessment of vibration injury to bartlett pears. Trans. ASAE 36(4):1043–1047. doi: 10.13031/2013.28432.
- Springael, J., A. Paternoster, and J. Braet. 2018. Reducing postharvest losses of apples: Optimal transport routing (while minimizing total costs). Comput. Electron. Agric. 146:136–144. doi: 10.1016/j.compag.2018.02.007.
- Tabatabaekoloor, R., S.J. Hashemi, and G. Taghizade. 2013. Vibration Damage to Kiwifruits during. Int. J. Agric. Food Sci. Technol 4(5):467–474.
- Timm, E.J., G.K. Brown, and R. Armstrong. 1996. Apple damage in bulk bins during semi-trailer transport. Appl. Eng. Agric. 12:369–377. doi: 10.13031/2013.25661.
- Van Zeebroeck, M., E. Tijskens, E. Dintwa, J. Kafashan, J. Loodts, J. De Baerdemaeker, and H. Ramon. 2006. The discrete element method (DEM) to simulate fruit impact damage during transport and handling: Case study of vibration damage during apple bulk transport. Postharvest Biol. Technol. 41:92–100. doi: 10.1016/j.postharvbio.2006.02.006.
- Vursavus, K., and F. Ozguven. 2004. Determining the effects of vibration parameters and packaging method on mechanical damage in golden delicious apples. Turk. J. Agric. For. 28:311–320.
- Whiley,, A.W., B. Schaffer, and B.N. Wolstenholme. 2013. The avocado botany. Production and Uses, New York. 416 p.
- White,, A., Woolf, A., Hofman, P., and Arpia, M., 2009. Manual internacional de la calidad del aguacate. Plant & food research, Nueva Zelanda.
- Woolf, A., M. Wong, L. Eyres, T. mcghie, C. Lund, S. Olsson, Y. Wang, C. Bulley,, M. Wang, E. Friel, et al. 2009. Avocado Oil. Gourmet Health Promoting Specialty Oils. doi: 10.1016/B978-1-893997-97-4.50008-5.
- Wu, G., and C. Wang. 2014. Investigating the effects of simulated transport vibration on tomato tissue damage based on vis/NIR spectroscopy. Postharvest Biol. Technol. 98:41–47. doi: 10.1016/j.postharvbio.2014.06.016.
- Zhang, Z., Q. Zhu, M. Hu, Z. Gao, F. An, M. Li. 2017. Low-temperature conditioning induces chilling tolerance in stored mango fruit. Food Chem. 219:76–84. doi: 10.1016/j.foodchem.2016.09.123.