ABSTRACT
Abstract: Postharvest diseases and loss of freshness are major problems that cause the deterioration of dragon fruit. The present study determined the effect of hot water treatment (HWT) at 50°C for 5 min followed by 1.0% chitosan and 0.2% κ-carrageenan-based composite coating to counter the issues. The results showed that HWT followed by the composite coating-enhanced antioxidant enzyme activities and reduced accumulation of H2O2 in the peel, which demonstrated the defensive response in the tissues leading to reduce disease development. The chitinase and β-1,3-glucanase did not show a significant effect in reducing the postharvest diseases of dragon fruit. While the HWT helped to increase phenolics content and antioxidant capacity in the pulp, the composite coating was crucial to maintain green color of the bract. Therefore, we concluded that HWT combined with composite coating controlled the diseases via antioxidant defensive response and maintained the overall quality of dragon fruit for 30 days of storage at 10°C.
Introduction
Dragon fruit (Hylocereus undatus) is a tropical fruit with high nutritional composition and attractive appearance with a colorful red peel and bright green bracts. It is commercially cultivated in at least 22 countries of the tropics (Gunasena, Citation2007). However, the fruit undergoes rapid deterioration in the postharvest period due to its susceptibility to a wide range of postharvest diseases. The major diseases in dragon fruit are caused by a large number of fungi such as Bipolaris cactivora, Colletotrichum sp., Alternaria sp., Fusarium sp., Rhizopus stolonifer (Castro et al., Citation2017; Nguyen Khanh et al., Citation2017; Ortiz-Hernández and Carrillo-Salazar, Citation2012). Besides, shriveling of the fruit and yellowing of the bract during senescence substantially limit its commercial value (Ali et al., Citation2013; Hoa et al., Citation2006).
Edible coating has been known as a safe technology to maintain freshness and prolong the shelf life of fruits and vegetables. An ideal coating could provide a barrier, which can retard loss of water vapor, exert the same effect as modified atmosphere packaging (MAP) in modifying the internal gas composition and reduce quality changes and quantity loss of fresh produces (Park, Citation1999). Recently, composite and bilayer coatings – combination of polymers – have intensely interested many due to combined beneficial properties of coating materials. Composite coating has the potential to alter permeability or mechanical properties of single-layer coating (Dhall, Citation2013). Regarding coating materials, many studies have concerned about the combination of chitosan and κ-carrageenan to develop composite coating due to opposite ionic charges in their structures. The interaction of κ-carrageenan and chitosan created an improved physico-mechanical properties film, resulting in decreased water vapor permeability compared to single-layer chitosan film, which is a poor water vapor barrier (Park et al., Citation2001; Pinheiro et al., Citation2012; Shahbazi et al., Citation2016). Also, Pinheiro et al. (Citation2012) reported that the κ-carrageenan/chitosan nanolayered coating exhibited a lower oxygen permeability compared to chitosan or ι-carrageenan film. In our recent previous study, we investigated the effect of the chitosan- and κ-carrageenan-based composite coating on dragon fruit. We found that 1.0% chitosan and 0.2% κ-carrageenan-based composite coating maintained freshness and reduced weight loss of the fruit, while single-layer chitosan coating could not provide that effect. However, as our finding, the mitigating effect on the disease in dragon fruit was not obtained upon applying chitosan coating alone or composite coating. On the other hand, Ali et al. (Citation2013) reported that chitosan coating reduced anthracnose symptoms in dragon fruit caused by C. gloeosporioides, in vivo test, while the effect of chitosan coating on the naturally infected diseases in dragon fruit has not been investigated. Therefore, other techniques should be investigated for combining with the chitosan- and κ-carrageenan-based composite coating to control the postharvest diseases in dragon fruit.
During the last decade, due to the concern about food safety, safe and eco-friendly postharvest technologies have increasingly interested many instead of synthetic fungicides to control postharvest diseases. Hot water treatment (HWT) is the simplest and oldest method among heat treatments to protect fruits and vegetables against pathogens. High temperature exhibits either lethal or sub-lethal effect to prevent fungi germination and growth (Klein and Lurie, Citation1992). Many studies have reported the effect of HWT on reducing the decay severity caused by Colletotrichum musae, Monilinia laxa, Monilinia fructicola, Fusarium oxysporum, Botrytis cinerea in various fruits (Fallik and Ilić, Citation2017). Additionally, HWT has been known as the elicitor for defense response activation in harvested fruits. HWT induced the accumulation of pathogenesis-related proteins including chitinase and β-1,3-glucanase in grapefruit and peach fruit (Liu et al., Citation2012; Pavoncello et al., Citation2001). HWT also significantly increased the activity of antioxidant enzymes or upregulated defense-related genes expression in kiwifruit and peach (Chen et al., Citation2015; Huan et al., Citation2017). However, beyond optimum exposure, HWT can cause fruit injury including shriveling or failure to soften (Alvindia, Citation2012), transpiration and mass loss (Caleb et al., Citation2016), the incidence of decay and high electrolyte leakage due to tissue damage (Yoon et al., Citation2018). The exceeded exposure time can also seriously damage the fruit color quality during ripening (Chavez-Sanchez et al., Citation2013). In dragon fruit, HWT has been used to control diseases in postharvest stage. According to Jitareerat et al. (Citation2018), HWT at 55°C for 5 min combined with 1% potassium sorbate reduced the severity of diseases in dragon fruit stored at 13°C. However, the mechanism for inhibitory effect of HWT on the disease involved in the defense system of dragon fruit was not elucidated. Meanwhile, Nguyen Khanh et al. (Citation2017) reported that severe injury occurred in dragon fruit dipped in hot water at about 51°C for 2 or 3 min before storage at 5°C. Meanwhile, alternative technologies such as edible coating have been investigated to combine with HWT to avoid unexpected damages as well as achieve a stronger effect in maintaining the quality of the fruit (Ban et al., Citation2015; Djioua et al., Citation2010).
So, both HWT and chitosan- and κ-carrageenan-based composite coating provided benefits to maintain the quality of dragon fruit during storage but did not attain the overall quality. Consequently, our study aimed to evaluate the effect of HWT, followed by chitosan and κ-carrageenan-based composite coating during postharvest handling processes, to not only maintain freshness, but also minimize postharvest diseases in dragon fruit. Furthermore, HWT-induced resistance in dragon fruit was examined to understand the defense mechanism of HWT against pathogens in dragon fruit.
Materials and Methods
Fruit Materials
Dragon fruit (Hylocereus undatus) in uniform size and maturity stage (over 80% of the surface of the fruit pericarp was bright red) was harvested from an orchard in Pathum Thani province (May 2019-September 2019) and delivered to the Laboratory of Postharvest Technology, School of Bioresources and Technology, King Mongkut’s University of Technology Thonburi within 2 h. The non-defected fruit was cleaned with tap water, then sanitized by dipping in 150 mg/L sodium hypochlorite for 5 min and subsequently air-dried for 20 min in a 25°C, 65–75% RH room before treatment.
Preparation of Composite Coating Solution
The coating solution was prepared according to our recent study. Firstly, 0.2% (w/v) κ-carrageenan (sulfated plant polysaccharide, Sigma Chemical Co., USA) was dissolved in distilled water and stirred for 1 h at 60°C. After being cooled down to room temperature, the solution was acidified by 1.0% (v/v) acetic acid. Subsequently, 1% chitosan {low molecular weight (50,000–190,000 Da), Sigma Chemical Co., USA} was added and stirred for 5 h to dissolve completely. Then, 0.75% (w/v) glycerol (Promega Co., USA) was added and the mixture was thoroughly stirred for 1 h. The coating solution was then homogenized at approximately 10,000 rpm by IKA T25 digital Ultra Turrax for 2 min. Finally, 1 M NaOH was used to adjust the pH to 5.4.
Experimental Designs
The composition of the coating solution was selected according to the results of our recent study. The HWT condition at 50°C for 5 min was defined based on the results of a preliminary experiment (Figure S1) on HWT for dragon fruit conducted at three different temperatures (47, 50, 53°C) and two dipping periods (3, 5 min). In this experiment, cleaned dragon fruit was randomly divided into four groups of 78 fruits each. No treatment was carried out for the first group before storage. The fruits of the second group were applied with the 1.0% chitosan and 0.2% κ-carrageenan-based composite coating. The remaining two groups of dragon fruit were treated in hot water at 50°C for 5 min and then air-dried. One of the hot water-treated groups was then coated with the 1.0% chitosan and 0.2% κ-carrageenan-based composite coating. After that, dragon fruits were stored at 10°C, 85–90% relative humidity, in a plastic basket with the surface covered with a polyethylene film. The evaluation of dragon fruit was performed before treatment, after 24 h, 48 h, 72 h and every 6 days during storage.
Disease Incidence and Severity
Disease incidence: The evaluation was done on 15 fruits of three replicates from each treatment. Disease incidence was expressed as the percentage of decay fruit per total number of fruits observed in each treatment.
Disease severity: Disease severity of dragon fruit was scored according to the rating scale (0: 0% of surface rotten; 1: 0 < rotten area ≤ 5% of surface; 2: 5% < rotten area < 10% of surface; 3: 10% < rotten area < 25% of surface; 4: 25% < rotten area < 50% of surface; 5: > 50% rotten area).
Bract Color
The bract color of the dragon fruit was measured based on the Commission International del’ Eclairage (CIE) color system (L*, a*, b*) using a colorimeter (model CR–400, Minolta, Japan). The measurement was carried out on the largest surface area of three randomly bracts at the upper part of each fruit (n = 5). Hue angle (ho) is used to express the color of bract.
Total Soluble Solids and Titratable Acidity
Total soluble solids (TSS): TSS of fruit juice was measured using a digital refractometer (PAL 1, Atago Co Ltd.). The results were expressed as degree Brix (oBrix).
Titratable acidity (TA): TA of fruit juice was determined by titration with 0.1 N NaOH solution in the presence of 1.0% phenolphthalein. TA was expressed as percentage (w/w) of citric acid.
Total Phenolic Content, Vitamin C Content and Antioxidant Activity (DPPH Scavenging Activity and FRAP Assay)
Total phenolic content: Total phenolic content of fruit pulp was determined by the Folin–Ciocalteu reagent (Singleton et al., Citation1999). In brief, supernatant extracted in methanol was reacted with Folin–Ciocalteu reagent for 2 h at 25°C with the presence of 1 N Na2CO3. After that, absorbance was recorded at 725 nm using an UV 1601 spectrophotometer (Shimadzu, Japan). Total phenolic content was expressed as g/kg of gallic acid equivalent (GAE) based on fresh weight.
Vitamin C content: Total vitamin C was determined by 2,4 dinitrophenyl hydrazine (DNP) method (Roe et al., Citation1948) with some modifications. 0.4 mL of the supernatant extracted in 5% metaphosphoric was reacted with 0.2 mL of 0.02% 2,6-Dichlorophenolindophenol. Then, 0.4 mL of 2% thiourea and 0.2 mL of 2% dinitrophenyl hydrazine were added. The reaction mixture was incubated for 1 h at 50°C. After that, 1 mL 85% sulfuric acid was added and incubated at 25°C for 30 min before measuring absorbance at 540 nm. Total vitamin C content was calculated based on a standard curve of L-ascorbic acid at 0, 10, 20, 30, 40, 50, 60 µg/mL and expressed as g/kg of fresh weight.
2,2-diphenyl-1-picrylhydrazyl (DPPH) scavenging activity: DPPH assay was performed according to (Li et al., Citation2017). The methanol extract supernatant was added to 110 µM DPPH dissolved in methanol (working DPPH). The reaction mixture was incubated at 25°C for 30 min. Absorbance was measured at 517 nm for sample mixture and working DPPH as control. DPPH scavenging activity was calculated by the formula: % Inhibition = [(A0-A1)/A0] × 100; Where A0 is the absorbance of the control; A1 is the absorbance of test samples.
Ferric reducing antioxidant power assay (FRAP): FRAP was determined following (Benzie and Strain, Citation1996) with slight modification. FRAP reagent included 10 mL of 300 mM acetate buffer, pH 3.6; 1 mL of 10 mM TPTZ (2,4,6-tripyridyl-s-triazine) in 40 mM HCl, and 1 mL of 20 mM FeCl3.6H2O. Supernatant extracted in methanol was added with freshly prepared FRAP reagent warmed to 37°C. The reaction mixture was then incubated at 25°C for 30 min. After that, absorbance was recorded at 593 nm. FRAP was expressed as g/kg of ascorbic acid equivalent (AsA) based on fresh weight.
Assays of Pathogen-related Proteins
Enzyme crude extraction: Two grams of frozen peel of dragon fruit were homogenized in 10 mL of 100 mM sodium acetate buffer pH 5.0. The homogenates were then centrifuged at 16,000 x g for 20 min at oC.
Chitinase (CHI) activity: CHI activity was measured according to Nguyen et al. (Citation2012) with some adjustment. The reaction mixture included 500 µL of crude enzyme and 500 µL of 3% colloidal chitin in 0.1 M sodium acetate buffer pH 5.2. After incubation for 2 h at 37°C, the reaction was stopped by centrifuge at 12,000 x g for 20 min. After that, 500 µL supernatant was added with 1 mL of 1% 3,5 dinitrosalicylic acid (DNS) and boiled for 5 min. For each enzyme preparation measured, an enzyme blank containing 0.1 M sodium acetate buffer pH 5.2 instead of colloidal chitin was carried through the procedure. The produced reducing sugar was determined by absorbance at 540 nm (absorbance of the blank was subtracted) using Ν-acetyl-D-glucosamine as the standard. One unit of CHI activity was defined as µmol Ν-acetyl-D-glucosamine produced per min under these assay conditions.
β-1,3-glucanase activity: GLU activity was determined following Zheng et al. (Citation2011). The crude enzyme (100 µL) extracted in sodium acetate buffer pH 5.0 was incubated with 50 µL of 0.4% laminarin at 37oC for 1 h. After that, 1 mL of 1% DNS was added and boiled for 5 min to stop the reaction. The mixture was then cooled on ice and absorbance was read at 540 nm. 1 unit of GLU activity was defined as mmol of glucose equivalents released per h from laminarin under the assay conditions (glucose content in the enzyme blank was subtracted).
H2O2 Content and Defense-related Enzymes Activities Analysis
H2O2 content: H2O2 content was measured using a modified method from Sagisaka (Citation1976). One g of frozen peel tissue was homogenized in 10 mL of 5% trichloroacetic acid (TCA) and then centrifuged at 12,000 x g for 10 min at 4°C. After that, 0.4 ml of 50% TCA and 0.4 ml of 10 mM ferrous ammonium sulfate were added to 2 mL supernatant. The color developed by adding 0.2 mL of 2.5 M potassium thiocyanate was measured at 480 nm. For each test tube prepared, a bank containing 0.2 mL of 50% TCA instead of potassium was carried through the procedure. H2O2 content was calculated with a plotted standard curve of H2O2 and expressed as µmol/g based on fresh weight.
Ascorbate peroxidase (APX) activity: Two grams of frozen peel of dragon fruit were homogenized in 10 ml 50 mM sodium phosphate buffer pH 7.0 containing 0.5 g polyvinylpolypyrrolidone (PVPP). The homogenates were then centrifuged at 16,000 x g for 20 min at 4oC. APX was determined following Andrade Cuvi et al. (Citation2011) with slight modification. The reaction mixture included 0.2 mL of crude enzyme, 1 mL of 100 mM sodium phosphate buffer pH 7.0, 1.0 mL of 0.1 mM EDTA, 1.5 ml of 0.5 mM ascorbic acid and 50 µL of 20 mM H2O2. APX activity was determined by decreasing absorbance at 290 nm due to oxidation of ascorbic acid. One unit of APX was defined as the change of absorbance per min under the specific condition.
Catalase (CAT) activity: CAT activity assay was according to (Beers and Sizer, Citation1952) with some adjustment. The reaction mixture included 0.2 mL of crude enzyme extracted in 50 mM sodium phosphate buffer pH 7.0, 1 mL of 100 mM sodium phosphate buffer pH 7.0 and 150 µL of 20 mM H2O2. CAT activity was determined by decreasing absorbance at 240 nm due to the action of CAT on hydrogen peroxide. One unit of CAT was defined as the change of absorbance per min under the specific condition.
Protein content in the enzyme crude was determined by the (Bradford, Citation1976) method, using bovine serum albumin as a standard. Specific activities of all enzymes were expressed as units per mg protein.
Statistical Analysis
Analysis of variance (ANOVA) was performed and Fisher’s least significant difference (LSD) test was used to compare the measured mean values at each sampling day at p ≤ 0.05 and p ≤ 0.01 using SPSS software version 19.0 for MS-Windows. Disease incidence data were transformed to sin(−1)(disease incidence) prior to ANOVA, according to (Boonkorn et al., Citation2012).
Results
Disease Incidence and Severity in Dragon Fruit
shows that HWT significantly reduced the disease incidence and severity in dragon fruit. Disease symptoms on the body of dragon fruit visually occurred after 18 days of storage in non-treated and coated dragon fruit, reaching 100% and 93.33%, respectively, on day 30. Meanwhile, disease symptoms were not found in HWT-treated fruits until day 24. A significantly (p < 0.05) lower percentage of disease incidence was seen after 30 days of storage in HWT-treated groups compared to remaining groups (). HWT also strongly reduced disease severity on the fruit body (). After 30 days of storage, disease severity in non-treated or coated fruits scored equal over 10% rotten area of the surface, while it was less than 5% in HWT-treated fruits, according to the rating scale.
Figure 1. Changes in body disease incidence and severity (A, B) (n = 15) in dragon fruit coated with 1.0% chitosan- and 0.2% κ-carrageenan-based composite, HWT, or HWT + composite coating, compared to non-treated fruit, during storage at 10°C. Different letters above bars indicate significant differences (p < 0.05) on the same storage day according to Fisher’s LSD test
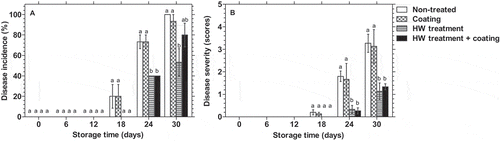
Activities of Pathogen-related Proteins
GLU and CHI, which are fungal resisting enzymes were typically low in the peel of dragon fruit throughout storage, as indicated by non-treated fruit. During the first three days of storage, the GLU and CHI activities increased to a higher level compared to day 0, except for that CHI activity in non-treated fruit was not detected. There was no significant difference observed among the treatments in GLU and CHI activities at this stage of storage ( and ). At the later stage of storage, the composite coating and HWT provided a stronger effect on GLU activity than CHI activity. and show that the individual treatment or combination of HWT and composite coating significantly (p < 0.05) increased GLU activity in the peel after 12 and 24 days of storage. At the end of storage, GLU activity was in the similarity between non-treated and coated fruits but was markedly higher in HWT alone or HWT + composite coating treated fruit. Meanwhile, HWT and composite coating could not markedly induce the activity of CHI during the storage, except on day 18 when CHI activity was significantly (p < 0.05) higher in the fruit coated with composite coating and on day 30 in the fruit treated with HWT + composite coating. Nevertheless, CHI activity was obviously not found in non-treated dragon fruit during storage, but detected in treated dragon fruits, almost every time of assessment ( and ).
Figure 2. Changes in β-1,3-glucanase (A, B) and chitinase activities (C, D) (n = 3) in the peel of dragon fruit coated with 1.0% chitosan- and 0.2% κ-carrageenan-based composite, HWT, or HWT + composite coating, compared to non-treated fruit, during storage at 10°C. Different letters above bars indicate significant differences (p < 0.05) on the same storage day according to Fisher’s LSD test
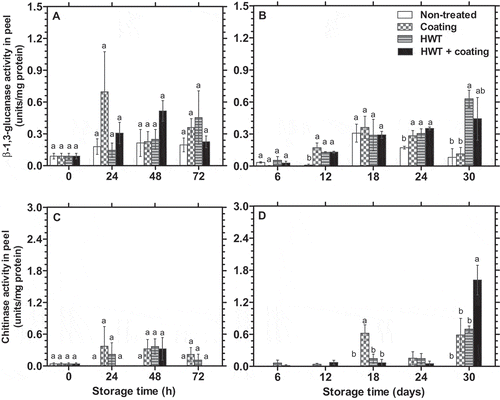
H2O2 Content and Defense-related Enzymes Activities
shows the effect of the 1% chitosan- and 0.2% κ-carrageenan- based composite coating, HWT, or HWT combined the coating on H2O2 content, CAT and APX activities in the peel of dragon fruit. and show that both the composite coating and HWT reduced the accumulation of H2O2 in the peel of dragon fruit. H2O2 content reduced noticeably after 24 h of storage in all groups of dragon fruit but was significantly (p < 0.05) higher in non-treated than treated fruits. After that, H2O2 content in non-treated dragon fruit increased and reached the highest value on day 6 (25.78 µmol/g), while the increment was observed in treated fruits only after 48 h before the gradual decrease. Both the individual composite coating and HWT or their combination showed a significant (p < 0.05) effect on inhibiting the accumulation of H2O2, at 24 h, day 6, and day 24 of storage.
Figure 3. Changes in H2O2 content (A, B), CAT (C, D), and APX activities (E, F) (n = 3) in the peel of dragon fruit coated with 1.0% chitosan- and 0.2% κ-carrageenan-based composite, HWT, or HWT + composite coating, compared to non-treated fruit, during storage at 10°C. Different letters above bars indicate significant differences (p < 0.05) on the same storage day according to Fisher’s LSD test
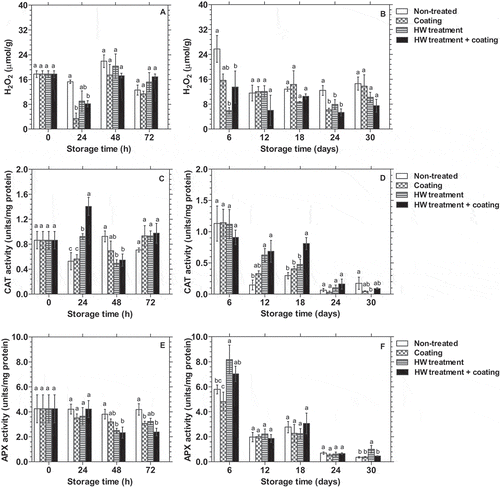
HWT alone and HWT combined with the composite coating affected CAT and APX activities depending on storage time. The CAT activity in the peel of the control and coated dragon fruit decreased considerably after 24 h of storage. Meanwhile, it was maintained in HWT-treated fruit and increased dramatically in HWT + composite coating treated fruits. Then, CAT activity in fruits treated with HWT or HWT followed by composite coating declined markedly, while it increased in non-treated and coated fruits after 48 h of storage (). Even though CAT activity decreased gradually during the rest of storage time across all treatments, it was significantly higher (p < 0.05) in fruits treated with HWT alone or HWT combined with composite coating compared to non-treated fruit on day 12 and day 18 ().
As shown in , APX activity in all treated dragon fruits decreased considerably after 48 h and 72 h of storage but remained quite stable in the control fruit. However, APX activity in dragon fruit then substantially increased in all treatments on day 6 (). Just then, the APX activity in HWT and HWT + composite coating treated fruits were significantly higher (p < 0.05) than that in the control and coated fruit. During the rest of storage time, APX activity of dragon fruit was similar in all treatments (p < 0.05), except on day 30 when higher activity was observed in HWT-treated fruit.
Changes in Total Phenolic, Vitamin C Content, and Antioxidant Activities in Fruit Pulp
shows that the HWT followed by chitosan- and κ-carrageenan-based composite coating improved the phenolic accumulation, maintained vitamin C content, and increased antioxidant activities in dragon fruit. Total phenolic content in dragon fruits across all treatments tended to increase slightly after being stored in the cold condition. During the storage, although a small fluctuation was observed, individual composite coating and HWT or their combination showed higher accumulation of phenolic in dragon fruits compared to the control fruit. However, a significant difference (p < 0.05) was recognized only after 18 and 24 days of the storage ().
Figure 4. Changes in phenolic content (A), vitamin C content (B), DPPH inhibition (C), FRAP antioxidant activity (D), TSS (E), and TA (F) (n = 3) in the pulp of dragon fruit coated with 1.0% chitosan- and 0.2% κ-carrageenan-based composite, HWT, or HWT + composite coating, compared to non-treated fruit, during storage at 10°C. Different letters above bars indicate significant differences (p < 0.05) on the same storage day according to Fisher’s LSD test
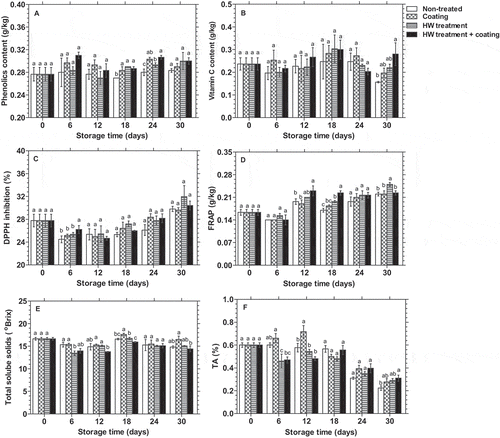
shows the change in Vitamin C content in the dragon fruit during 30 days of storage. Vitamin C gradually increased until day 18 of storage before slight reduction. After 30 days of storage, vitamin C content of 0.16 g/kg FW in non-treated dragon fruit was noticeably lower than the initial level (0.24 g/kg FW) and significantly (p < 0.05) lower than that of 0.28 g/kg FW in dragon fruit treated with HWT followed by composite coating.
Antioxidant activity in terms of DPPH scavenging activity in the dragon fruit reduced in the initial stages of storage and then increased gradually. There was almost no significant difference in DPPH inhibition activity observed, except on day 6 when the percentage of DPPH inhibition in HWT + coating treated dragon fruit was significantly higher than that in other treatments (). On the other hand, antioxidant activity in the dragon fruit in terms of FRAP increased noticeably during storage across all treatments. There was a significant difference (p < 0.05) in FRAP between treatments on days 12, 18 and 30 of storage. While FRAP antioxidant activity in the dragon fruit treated with HWT + coating was at the highest level on days 12 and 18, it was significantly higher in HWT alone treated group after 30 days of storage compared to others ().
and f show that the treatments also provided an effect on TSS and TA in the pulp of dragon fruit. TSS in non-treated and composite coated fruit was significantly (p < 0.05) higher than that in HWT alone-treated fruit on day 6 of storage. Meanwhile, TSS in dragon fruit coated with composite coating showed a significantly (p < 0.05) higher level than others on day 18. At the end of the storage, TSS content in dragon fruit in all treatments was slightly lower than the initial content, but no significant (p < 0.05) difference was found between the treated fruits compared to the control (). TA in the pulp of the dragon fruit showed a decline during storage in all treatments, except TA in coated fruit exposed a slight increase during 12 days of storage. On day 6, TA content in coated dragon fruit was significantly higher (p < 0.05) than that of the fruit sample exposed to HWT, but it was not significantly (p < 0.05) different to the control fruit. However, coated dragon fruit showed a significantly higher level (p < 0.05) than all other groups at TA level on day 12. After that, TA content in dragon fruit in all treatments reduced markedly, and no noticeable difference was observed between treatments ().
Changes in Bract Color
Bract color of dragon fruit represented by Hue value (ho) was affected by the treatments. The ho in the bract of non-treated and HWT-treated dragon fruits declined sharply since day 18, while the changes were markedly slower in coating or HWT + coating treated fruits (). After 30 days of storage, ho values in the bract of coated and HWT + coating treated fruits were 107.95 and 108.32, respectively, which were significantly higher than those in the control (98.95) and HWT treated fruit (96.97). Besides, further shows that composite coating and HWT + coating treatments maintained freshness and green color of the fruit bracts after 30 days of storage compared to control and HWT alone treated fruits.
Figure 5. Changes in bract color (ho value) (n = 5) in dragon fruit coated with 1.0% chitosan- and 0.2% κ-carrageenan-based composite, HWT, or HWT + composite coating, compared to non-treated fruit, during storage at 10°C. Asterisk (*) indicates significant differences (p < 0.05) on the same storage day according to Fisher’s LSD test
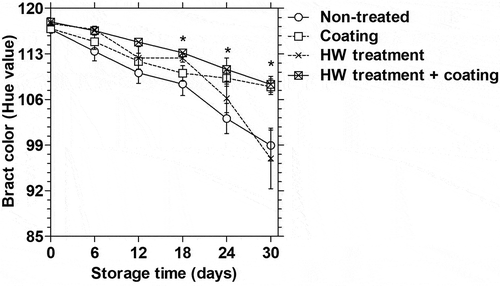
Figure 6. Visual appearance of dragon fruit coated with 1.0% chitosan- and 0.2% κ-carrageenan-based composite, HWT, or HWT + composite coating, compared to non-treated fruit, after 30 days of storage at 10°C. The green arrow indicates fresh and green bracts; the yellow arrow indicates the wilted and degreened bracts; the blue arrow indicates disease symptom
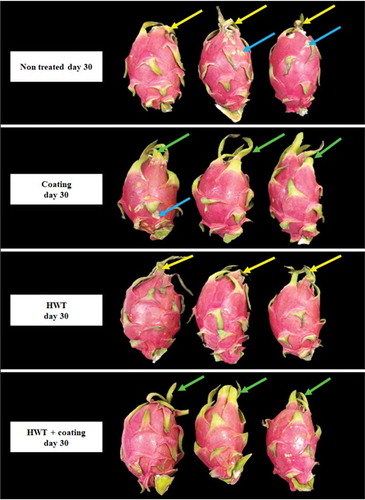
Discussion
HWT Followed by Chitosan- and κ-Carrageenan-Based Composite Coating Reduced Disease Development and Induced Resistance in Dragon Fruit
One of the major restrictions in dragon fruit production and commerce are postharvest diseases caused by various fungal pathogens. Consistent with our study, Ali et al. (Citation2013) found that disease incidence in dragon fruit reached 99.9% with over 75% of the rotten surface after 28 days of storage at 10°C. Jitareerat et al. (Citation2018) reported that HWT at 55°C for 5 min combined with 1% potassium sorbate reduced disease severity on the surface of the dragon fruit stored at 13oC for 15 days. Besides, according to Nguyen Khanh et al. (Citation2017), HWT at 49°C and 51°C for two or three min inhibited the rot on the body of the dragon fruit stored at 5°C for 28 days. Based on the results of our preliminary experiment, HWT at 50°C for 5 min was selected for this study and demonstrated being the best condition to control the disease on the body of the dragon fruit stored at 10°C (). Meanwhile, the chitosan- and κ-carrageenan-based composite coating did not provide a controlling effect on the disease development in the fruit, which was similar to the finding of Shi et al. (Citation2018) and Arnon et al. (Citation2014) on grapefruit and citrus fruits.
CHI and GLU activities were apparently traced in the peel of non-treated dragon fruit during low-temperature storage. CHI and GLU are two pathogenesis-related (PR) proteins involved in inducible or active defense mechanisms. CHI and GLU attack chitin and β-1,3-glucan, respectively, which are components of cell walls in many fungi. Besides, fragmentation of the fungal cell wall by these enzymes also generates oligosaccharides that induce plant defense responses (Ferreira et al., Citation2007). The results in the current study showed that even though both HWT and composite coating induced noticeable GLU activity in the peel of the dragon fruit on 12, 24 and 30 days of storage but not at initial stages after treatments (within 72 h after treatment), composite coating alone could not control diseases on the body of the dragon fruit. Interestingly, there is no obvious activity of CHI in untreated fruit throughout storage while CHI showed increasing trend in the peel of treated fruits. However, there was no significant difference to the control observed except on day 18 and day 30. Consequently, these results indicated that induced CHI and GLU activities might not be the crucial inhibitory mechanism of HWT on disease symptoms in the dragon fruit. According to Vidhyasekaran (Citation2008), although CHI and GLU, in general, show antifungal activity, some forms of these PR proteins apparently lack this activity. However, the transcription of CHI and GLU genes should be analyzed further to provide evidence on it. Similarly, Wei et al. (Citation2018) reported that heat treatment alone induced CHI activity in strawberry only after 12 h of storage and insignificantly increased GLU activity, although it reduced the gray mold in the fruit inoculated with B. cinerea. The induced CHI and GLU activities by HWT and chitosan in this study in some assessment time was in agreement with findings in previous studies. HWT increased CHI and GLU activities in sweet cherry (Wang et al., Citation2015) and mango (Sripong et al., Citation2015). Chitosan has been reported to improve CHI activity in grapefruit (Shi et al., Citation2018) and avocado (Obianom et al., Citation2019). The accumulation of some PR proteins such as CHI and GLU can be involved in cell wall thickening or release elicitor molecules from the plant tissues. These elicitors may stimulate the biosynthesis of phenolic compounds or other PR proteins (Vidhyasekaran, Citation2008).
The oxidative burst in plant tissue is one of the earliest responses to pathogen attack as a hypersensitive response. However, overproduction and accumulation of reactive oxygen species (ROS) such as superoxide anion (O2•−) and hydrogen peroxide (H2O2) cause oxidative stress, resulting in cell damage (Demidchik, Citation2015). H2O2 content accumulated in treated dragon fruits was significantly lower than that in non-treated fruit in general, but the high and equal levels were observed at 48 h of storage ( and ). CAT and APX are two enzymatic antioxidants that play an important role in the plant to withstand oxidative stress (Demidchik, Citation2015). In the present study, HWT alone or combined with composite coating immediately enhanced CAT activity in the peel of the dragon fruit at 24 h and mitigated the declines in the later stages of the storage ( and ). Besides, APX activity was significantly higher in HWT and HWT + composite coating treated dragon fruits on day 6 of storage ( and ). This was in correlation with a low level of H2O2 observed in HWT-treated groups. Meanwhile, CAT and APX activities were reduced in HWT fruits at 48 h, consistent with the increased H2O2 content. Therefore, the results in the present study demonstrated the effect of heat treatment on the maintenance of cell redox homeostasis in the dragon fruit via antioxidant enzymes. This could play an important role in reducing disease symptom on the body of the fruit. In agreement with our study, Huan et al. (Citation2017) found that expression of PpaAPX2 gene was suppressed by heat treatment in peach fruit on day 1, but increased and maintained high level after that. Expression level of PpaCATs was also higher in heat-treated peach fruit in the later stage of storage. Similarly, heat treatment prevented reduction of CAT and APX during the storage of strawberry (Jin et al., Citation2016). Subsequently, the results in our study suggested that HWT alone or combined with chitosan and κ-carrageenan-based composite coating controlled the disease in the dragon fruit by inducing resistance in it.
HWT Followed by Chitosan- and κ-Carrageenan-Based Composite Coating Maintained Quality Attributes of Dragon Fruit
The bract is an important part of which green color contributes to the attractive appearance of the dragon fruit, but the yellowing undergoes rapidly. Our recent study proved that chitosan- and κ-carrageenan-based composite coating delayed yellowing of the bracts of dragon fruit by suppressing activities of chlorophyll-degrading enzymes. In the present study, composite coating alone or combined with HWT maintained the bract color of the dragon fruit until after 30 days of storage, but HWT could not prevent the yellowing in the later stages (). This suggests that composite coating is crucial for maintaining the bract quality. In agreement with this, Nguyen Khanh et al. (Citation2017) reported that HWT at 51°C for 3 min damaged the bract of dragon fruit stored at 5°C. In contrast, Jitareerat et al. (Citation2018) found that HWT at 55°C for 5 min combined with sodium carbonate maintained the green color of the bract of the dragon fruit stored at 13°C for 15 days. It is supposed that different effects of HWT on bract color changed because of different storage temperature as well as storage duration. For further understanding, the effect of HWT on activities of chlorophyll-degrading enzymes in the bract of the dragon fruit should be investigated.
On the other hand, HWT alone or followed by composite coating promoted the accumulation of phenolic compounds, maintained vitamin C content and improved FRAP antioxidant activity in the pulp of the dragon fruit (–). In agreement with this, Jin et al. (Citation2016) reported that heat treatment enhanced the activity of phenylalanine ammonia-lyase (PAL), leading to an increase of phenolic content in strawberry. Ban et al. (Citation2015) also found that combination of heat treatment and chitosan coating maintained higher levels of ascorbic acid, total phenolic contents and antioxidant capacity in wolfberry.
HWT and composite coating of chitosan- and κ-carrageenan-based also affect the TSS and TA content in the pulp of the dragon fruit. Composite coating maintained TSS content and even increased TA level in the fruit, while HWT alone reduced both of them in the initial stages of storage. However, no significant difference was observed between the treatments in TSS and TA in the later stages of storage. In agreement with our results, previous studies also proved that HWT insignificantly affects TSS and TA content in dragon fruit (Jitareerat et al., Citation2018), wolfberry (Ban et al., Citation2015) and kiwifruit (Cheng et al., Citation2015) in the storage period.
Conclusions
The present study demonstrated that HWT at 50°C for 5 min followed by 1% chitosan- and 0.2% κ-carrageenan-based composite coating is a potential strategy to maintain the overall quality of dragon fruit during the storage at 10°C. HWT regulated H2O2 accumulation and CAT, APX enzymes activities in the peel, thus enhanced the resistance against postharvest disease. Meanwhile, the coating following HWT helped maintaining bract quality of dragon fruit. Furthermore, HWT alone or HWT combining with the composite coating improved the edibility of the fruit in terms of phenolic content and antioxidant activity.
Supplemental Material
Download MS Word (57.7 KB)Acknowledgments
This study was funded by Petchra Pra Jom Klao Ph.D. Research Scholarship (No. of agreement: 25/2560), King Mongkut’s University of Technology Thonburi. We also acknowledge the Postharvest Technology Innovation Center, Bangkok, Thailand, and the United Graduate School of Agricultural Science (UGSAS), Gifu University, Japan for providing us some apparatus facilities.
Supplementary Material
Supplemental data for this article can be accessed on the publisher’s website.
Additional information
Funding
References
- Ali, A., N. Zahid, S. Manickam, Y. Siddiqui, P.G. Alderson, and M. Maqbool. 2013. Effectiveness of submicron chitosan dispersions in controlling anthracnose and maintaining quality of dragon fruit. Postharvest Biol. Technol. 86:147–153. doi: 10.1016/j.postharvbio.2013.06.027.
- Alvindia, D.G. 2012. Revisiting hot water treatments in controlling crown rot of banana cv. Buñgulan. Crop. Prot. 33:59–64. doi: 10.1016/j.cropro.2011.09.023.
- Andrade Cuvi, M.J., A.R. Vicente, A. Concellón, and A.R. Chaves. 2011. Changes in red pepper antioxidants as affected by UV-C treatments and storage at chilling temperatures. LWT- Food. Sci. Technol. 44(7):1666–1671. doi: 10.1016/j.lwt.2011.01.027.
- Arnon, H., Y. Zaitsev, R. Porat, and E. Poverenov. 2014. Effects of carboxymethyl cellulose and chitosan bilayer edible coating on postharvest quality of citrus fruit. Postharvest Biol. Technol. 87:21–26. doi: 10.1016/j.postharvbio.2013.08.007.
- Ban, Z., W. Wei, X. Yang, J. Feng, J. Guan, and L. Li. 2015. Combination of heat treatment and chitosan coating to improve postharvest quality of wolfberry (Lycium barbarum). Int. J. Food. Sci. 50(4):1019–1025. doi: 10.1111/ijfs.12734.
- Beers, R.F., and I.W. Sizer. 1952. A spectrophotometric method for measuring the breakdown of hydrogen peroxide by catalase. J. Biol. Chem. 195(1):133–140.
- Benzie, I.F., and J.J. Strain. 1996. The ferric reducing ability of plasma (FRAP) as a measure of “antioxidant power”: The FRAP assay. Anal. Chem. 239(1):70–76. doi: 10.1006/abio.1996.0292.
- Boonkorn, P., H. Gemma, S. Sugaya, S. Setha, J. Uthaibutra, and K. Whangchai. 2012. Impact of high-dose, short periods of ozone exposure on green mold and antioxidant enzyme activity of tangerine fruit. Postharvest Biol. Technol. 67:25–28. doi: 10.1016/j.postharvbio.2011.12.012.
- Bradford, M.M. 1976. A rapid and sensitive method for the quantitation of microgram quantities of protein utilizing the principle of protein-dye binding. Anal. Chem. 72:248–254. doi: 10.1016/0003-2697(76)90527-3.
- Caleb, O.J., G. Wegner, C. Rolleczek, W.B. Herppich, M. Geyer, and P.V. Mahajan. 2016. Hot water dipping: Impact on postharvest quality, individual sugars, and bioactive compounds during storage of ‘Sonata’ strawberry. Sci. Hortic. 210:150–157. doi: 10.1016/j.scienta.2016.07.021.
- Castro, J.C., E.H. Endo, M.R. de Souza, E.B. Zanqueta, J.C. Polonio, J.A. Pamphile, T. Ueda-Nakamura, C.V. Nakamura, B.P. Dias Filho, and B.A. de Abreu Filho. 2017. Bioactivity of essential oils in the control of Alternaria alternata in dragon fruit (Hylocereus undatus Haw.). Ind. Crops. Prod. 97:101–109. doi: 10.1016/j.indcrop.2016.12.007.
- Chavez-Sanchez, I., A. Carrillo-Lopez, M. Vega-Garcia, and E.M. Yahia. 2013. The effect of antifungal hot-water treatments on papaya postharvest quality and activity of pectinmethylesterase and polygalacturonase. J. Food. Sci. Technol. 50(1):101–107. doi: 10.1007/s13197-011-0228-0.
- Chen, H., Z. Cheng, M. Wisniewski, Y. Liu, and J. Liu. 2015. Ecofriendly hot water treatment reduces postharvest decay and elicits defense response in kiwifruit. Environ. Sci. Pollut. Res. 22(19):15037–15045. doi: 10.1007/s11356-015-4714-1.
- Cheng, Y., L. Liu, G. Zhao, C. Shen, H. Yan, J. Guan, and K. Yang. 2015. The effects of modified atmosphere packaging on core browning and the expression patterns of PPO and PAL genes in ‘Yali’ pears during cold storage. LWT- Food. Sci. Technol. 60(2):1243–1248. doi: 10.1016/j.lwt.2014.09.005.
- Demidchik, V. 2015. Mechanisms of oxidative stress in plants: From classical chemistry to cell biology. Environ. Exp. Bot. 109:212–228. doi: 10.1016/j.envexpbot.2014.06.021.
- Dhall, R. 2013. Advances in edible coatings for fresh fruits and vegetables: A review. Crit. Rev. Food Sci. Nutr. 53(5):435–450. doi: 10.1080/10408398.2010.541568.
- Djioua, T., F. Charles, M. Freire, H. Filgueiras, M.-N. Ducamp-Collin, and H. Sallanon. 2010. Combined effects of postharvest heat treatment and chitosan coating on quality of fresh-cut mangoes (Mangifera indica L.). Int. J. Food. Sci. 45(4):849–855. doi: 10.1111/j.1365-2621.2010.02209.x.
- Fallik, E., and Z. Ilić. 2017. Hot water treatments, p. 241–258. In: S. Pareek (ed.). Novel postharvest treatments of fresh produce. CRC Press, Boca Raton.
- Ferreira, R.B., S. Monteiro, R. Freitas, C.N. Santos, Z. Chen, L.M. Batista, J. Duarte, A. Borges, and A.R. Teixeira. 2007. The role of plant defence proteins in fungal pathogenesis. Mol. Plant. Pathol. 8(5):677–700. doi: 10.1111/j.1364-3703.2007.00419.x.
- Gunasena, H. 2007. Dragon fruit Hylocerus undatus Haw Brittin and Rose, p. 110–141. In: D.K.N.G. Pushpakumara, H.P.M. Gunasena, and V.P. Singh (eds.). Underutilized fruit trees in Sri Lanka. World Agroforestry Centre, South Asia office, New Delhi, India.
- Hoa, T., C. Clark, B. Waddell, and A. Woolf. 2006. Postharvest quality of Dragon fruit (Hylocereus undatus) following disinfesting hot air treatments. Postharvest Biol. Technol. 41(1):62–69. doi: 10.1016/j.postharvbio.2006.02.010.
- Huan, C., S. Han, L. Jiang, X. An, M. Yu, Y. Xu, R. Ma, and Z. Yu. 2017. Postharvest hot air and hot water treatments affect the antioxidant system in peach fruit during refrigerated storage. Postharvest Biol. Technol. 126:1–14. doi: 10.1016/j.postharvbio.2016.11.018.
- Jin, P., C. Zheng, Y.-P. Huang, X.-L. Wang, Z.-S. Luo, and Y.-H. Zheng. 2016. Hot air treatment activates defense responses and induces resistance against Botrytis cinerea in strawberry fruit. J. Integr. Agric. 15(11):2658–2665. doi: 10.1016/s2095-3119(16)61387-4.
- Jitareerat, P., K. Sripong, K. Masaya, S. Aiamla-or, and A. Uthairatanakij. 2018. Combined effects of food additives and heat treatment on fruit rot disease and quality of harvested dragon fruit. Agric. Nat. Resour. 52(6):543–549. doi: 10.1016/j.anres.2018.11.019.
- Klein, J.D., and S. Lurie. 1992. Heat treatments for improved postharvest quality of horticultural crops. Hort. Technol. 2(3):316–320. doi: 10.21273/HORTTECH.2.3.316.
- Li, X., Q. Long, F. Gao, C. Han, P. Jin, and Y. Zheng. 2017. Effect of cutting styles on quality and antioxidant activity in fresh-cut pitaya fruit. Postharvest Biol. Technol. 124:1–7. doi: 10.1016/j.postharvbio.2016.09.009.
- Liu, J., Y. Sui, M. Wisniewski, S. Droby, S. Tian, J. Norelli, and V. Hershkovitz. 2012. Effect of heat treatment on inhibition of Monilinia fructicola and induction of disease resistance in peach fruit. Postharvest Biol. Technol. 65:61–68. doi: 10.1016/j.postharvbio.2011.11.002.
- Nguyen, H.A., T.H. Nguyen, T.T. Nguyen, C.K. Peterbauer, G. Mathiesen, and D. Haltrich. 2012. Chitinase from Bacillus licheniformis DSM13: Expression in Lactobacillus plantarum WCFS1 and biochemical characterisation. Protein. Expr. Purif. 81(2):166–174. doi: 10.1016/j.pep.2011.10.005.
- Nguyen Khanh, N., V.P. Nguyen, T. Nguyen Thanh, and V.H. Nguyen. 2017. Postharvest diseases and effect of hot water treatments on white fleshed dragon fruit [Hylocereus undatus (Haw.) Britton & Rose]. Int. J. Curr. Res. Biosci. Plant. Biol. 4(5):30–37. doi: 10.20546/ijcrbp.2017.405.003.
- Obianom, C., G. Romanazzi, and D. Sivakumar. 2019. Effects of chitosan treatment on avocado postharvest diseases and expression of phenylalanine ammonia-lyase, chitinase and lipoxygenase genes. Postharvest Biol. Technol. 147:214–221. doi: 10.1016/j.postharvbio.2018.10.004.
- Ortiz-Hernández, Y.D., and J.A. Carrillo-Salazar. 2012. Pitahaya (Hylocereus spp.): A short review. Comunicata. Scientiae. 3(4):220–237.
- Park, H.J. 1999. Development of advanced edible coatings for fruits. Trends. Food. Sci. Technol. 10(8):254–260. doi: 10.1016/S0924-2244(00)00003-0.
- Park, S.Y., B.I. Lee, S.T. Jung, and H.J. Park. 2001. Biopolymer composite films based on κ-carrageenan and chitosan. Mater. Res. Bull. 36(3):511–519. doi: 10.1016/S0025-5408(01)00545-1.
- Pavoncello, D., S. Lurie, S. Droby, and R. Porat. 2001. A hot water treatment induces resistance to Penicillium digitatum and promotes the accumulation of heat shock and pathogenesis related proteins in grapefruit flavedo. Physiol. Plant. 111(1):17–22. doi: 10.1034/j.1399-3054.2001.1110103.x.
- Pinheiro, A.C., A.I. Bourbon, B.G.D.S. Medeiros, L.H. da Silva, M.C. da Silva, M.G. Carneiro-da-Cunha, M.A. Coimbra, and A.A. Vicente. 2012. Interactions between κ-carrageenan and chitosan in nanolayered coatings—Structural and transport properties. Carbohydr. Polym. 87(2):1081–1090. doi: 10.1016/j.ifset.2012.06.004.
- Roe, J.H., M.B. Mills, M.J. Oesterling, and C.M. Damron. 1948. The determination of diketo l-gluonic acid, dehydro-l-ascorbic acid, and l-ascorbic acid in the same tissue extract by the 2, 4-dinitrophenylhydrazine method. J. Biol. Chem. 174:201–208.
- Sagisaka, S. 1976. The occurrence of peroxide in a perennial plant, Populus gelrica. Plant. Physiol. 57(2):308–309. doi: 10.1104/pp.57.2.308.
- Shahbazi, M., G. Rajabzadeh, R. Ettelaie, and A. Rafe. 2016. Kinetic study of κ-carrageenan degradation and its impact on mechanical and structural properties of chitosan/κ-carrageenan film. Carbohydr. Polym. 142:167–176. doi: 10.1016/j.carbpol.2016.01.037.
- Shi, Z., F. Wang, Y. Lu, and J. Deng. 2018. Combination of chitosan and salicylic acid to control postharvest green mold caused by Penicillium digitatum in grapefruit fruit. Sci. Hortic. 233:54–60. doi: 10.1016/j.scienta.2018.01.039.
- Singleton, V.L., R. Orthofer, and R.M. Lamuela-Raventós.1999. Analysis of total phenols and other oxidation substrates and antioxidants by means of folin-ciocalteu reagent. Methods Enzymol. 299:152–178. doi:10.1016/s0076-6879(99)99017-1
- Sripong, K., P. Jitareerat, S. Tsuyumu, A. Uthairatanakij, V. Srilaong, C. Wongs-Aree, G. Ma, L. Zhang, and M. Kato. 2015. Combined treatment with hot water and UV-C elicits disease resistance against anthracnose and improves the quality of harvested mangoes. Crop. Prot. 77:1–8. doi: 10.1016/j.cropro.2015.07.004.
- Vidhyasekaran, P. 2008. Induction and evasion of pathogenesis-related proteins, p. 345–409. In: VidhyasekaranP. (ed.). Fungal pathogenesis in plants and crops: Molecular biology and host defense mechanisms. CRC Press, Boca Raton
- Wang, L., P. Jin, J. Wang, H. Gong, S. Zhang, and Y. Zheng. 2015. Hot air treatment induces resistance against blue mold decay caused by Penicillium expansum in sweet cherry (Prunus cerasus L.). Fruit. Sci. Hortic. 189:74–80. doi: 10.1016/j.scienta.2015.03.039.
- Wei, Y., Y. Wei, F. Xu, and X. Shao. 2018. The combined effects of tea tree oil and hot air treatment on the quality and sensory characteristics and decay of strawberry. Postharvest Biol. Technol. 136:139–144. doi: 10.1016/j.postharvbio.2017.11.008.
- Yoon, H.S., I.-L. Choi, J.-Y. Heo, J.Y. Kim, S.J. Han, and H.-M. Kang. 2018. Influence of hot water immersion and MAP pre-treatments on sterilization and asparagus spear qualities during cold storage. Horticultural. Sci. Technol. 36(5):756–765. doi: 10.12972/kjhst.20180074.
- Zheng, Y., L. Shen, M. Yu, B. Fan, D. Zhao, L. Liu, and J. Sheng. 2011. Nitric oxide synthase as a postharvest response in pathogen resistance of tomato fruit. Postharvest. Biol. Technol. 60(1):38–46. doi: 10.1016/j.postharvbio.2010.12.003.