ABSTRACT
Developing improved water management strategies and on-farm cultural practices are important in reducing pomegranate (Punica granatum L. cv Wonderful) production costs. A pomegranate orchard established in 2010 was irrigated at water replacement rates of 35, 50 and 100% of crop evapotranspiration from 2013 to 2016. A durable white plastic tarp was installed in one half of each water replacement plot to evaluate the added benefits to soil moisture retention, weed control, and plant growth and yield. Edaphic orchard floor conditions such as light reflectance into the lower tree canopy, mid-day soil surface temperature, soil water content, and average daily soil temperatures were moderated or improved by the plastic cover. Phenological changes such as leaf greenness, trunk basal suckering, and trunk diameter were generally enhanced by increased water application rates and plastic use. Marketable and total fruit yields increased by an average of 20 to 29% from 2013 to 2016 with the use of plastic cover compared to bare soil. Total fruit yield increased linearly with increasing water replacement rates. Neither the white plastic nor irrigation rates improved external fruit color. Fruit raw product attributes were little changed by treatments. The research clearly demonstrated the benefit of plastic cover on improving pomegranate production.
Introduction
Plastic films and mulches are effective barriers to weed growth and can provide additional benefits to cropping systems e.g. soil temperature moderation, soil water conservation, reduction of certain insect pests, reduced herbicide use, and a subsequent reduction of herbicide-resistant weeds, higher crop yields, and more efficient use of soil nutrients (Bonanno, Citation1996; Kasirajan and Ngouajio, Citation2012). A durable (7+ year) nylon reinforced opaque white plastic film was found to control weeds, moderate near surface soil temperatures, and to improve light reflectance into the canopy of blackberries (Makus, Citation2011). Berry yields and fruit size and quality were usually improved with the use of the plastic compared to other materials (Makus, Citation2010, Citation2011).
Since the late 1990’s, orchardists have used opaque reflecting films that are placed in the orchard row centers usually 3 to 6 weeks prior to fruit harvest to improve fruit color and in some cases effect crop maturity and soluble solids. Some of the environmental changes these films provide include improved lower to mid-canopy light penetration, higher orchard floor temperatures, reduced humidity, and increased soil moisture underneath the film (Glenn and Puterka, Citation2007; Iglesias and Alegre, Citation2009; Layne et al., Citation2001; Prive et al., Citation2008; Sun et al., Citation2012). One of the apple color pigments found to increase from improved light intensity is cyanidin glucoside (Jakopic et al., Citation2010), which is also one of the major anthocyanins found in pomegranate juice (Legua et al., Citation2012).
Yield response has been well documented for a wide variety of crops and is generally characterized as a linear function of applied water. which is true for most annual herbaceous crops (Smith and Steduto, Citation2012). This response is not well defined for perennial fruit and nut crops. Pomegranate is considered a drought tolerant crop and thus a linear relationship between applied water and yield may not be applicable (Yuan et al., Citation2015). Also, the water requirement for pomegranate has not been well defined using drip irrigation in arid areas (Ayars et al., Citation2017; Intrigliolo et al., Citation2011, Citation2020; Wang et al., Citation2015; Zhang et al., Citation2017). Much of the research on the water requirement of pomegranate has been done using surface irrigation and in more temperate regions (Mellisho et al., Citation2012).
This study was undertaken to evaluate the effects deficit irrigation and long-term placement of a reflective white plastic film on tree phenology, edaphic stress modification, fruit yield and quality, and weed control in a surface drip irrigated pomegranate orchard located in a semi-arid region.
Material and Methods
Field Experiments and Data Collection
Pomegranate trees (Punica granatum L. cv Wonderful) were planted in 2010 with 5.4 m spacing between rows and 2.7 m spacing within the row. The 1.3 ha orchard site is located at the USDA-ARS San Joaquin Valley Agricultural Sciences Center, Parlier, CA (Lat. 36º N, Long.119º W; elevation 102 m) on a non-saline Hanford sandy loam soil. Trees were trained to a ‘V’ shape with 4 main leaders forming a vase shape and topped at 3 m height similar to the practice used by our commercial cooperator. Uniform amounts of water were applied by surface drip irrigation for the first two years to establish the orchard.
The experimental design was a complete randomized split-plot with water replacement or irrigation level as the main plot and ground cover (white plastic vs. bare ground) as the sub-plot with four replications. Beginning in 2013, four water replacement regimes, 35, 50, 75, and 100% of crop evapotranspiration (ETc) were established based on evapotranspiration measured by two large weighing lysimeters (Schneider et al., Citation1996), located near the center of the orchard and each containing 2 trees. The deficit applications were accomplished by using 0.35, 0.50, and 0.75 of the run-time for 100% water replacement as measured on the control lysimeter. We used only the 35, 50 and 100% plots in this study, since these treatments represented the extremes of water application. Therefore, a total of 12 main irrigation plots and 24 irrigation x ground cover sub-plots were used. Irrigation was controlled by one lysimeter and all water applications were measured with electronic- and manually- read water meters. The trees were irrigated with a single drip line placed next to the tree row that used one 4 L/h drip emitters, placed at 45 cm on either side of the tree and an 8 L/h emitter placed at the mid-point between the trees.
Each irrigation main plot consisted of 3 rows with 15 trees per row. In 2013, a 2.4 m wide nylon spun-bonded white on black plastic with white side up (T-55, Reef Industries, Houston, TX, USA) was installed by May 24 in each irrigation replacement main plot. The plastic plots were located on the north row of the water experimental plots. A total of eight trees per plot had the plastic installed below the trees and 1.2 m into the space between rows. Five trees under the plastic were selected for experimental data collection. The remaining 7 trees on the same row, not covered with the white plastic, were the companion bare ground treatment and 5 trees, excluding the 2 edge trees, were used for data collection. The plastic was kept in place continuously after installation until the experiment ended in 2016.
Irrigation was initiated when 4 mm of water loss was determined by the weighing lysimeter. A total of 4 mm was applied to the 100% treatment with the remaining treatments getting proportionately less water during each event. Irrigation was from April 9 to November 26, 2013, April 11 to October 31, 2014, March 1 to November 1, 2015, and March 12 to November 1, 2016. The fertilization regime for each year is summarized in . All fertilizers were applied using the drip irrigation system. Trees in the lysimeters were fertilized at the same rate as the field. Weeds in the bare ground plots, including the lysimeters, were controlled as needed by herbicide application of paraquat + surfactants.
Table 1. Summary of applied nitrogen (N), phosphorus (P), potassium (K), and sulfur (S) fertilizers in a multi-year pomegranate experiment conducted at the USDA-ARS San Joaquin Valley Agricultural Sciences Center, Parlier, California, U.S.A
Basal suckers were defined as anything growing from the main trunk below 5 cm above ground. The suckers were removed in December 2013 and January 2016. The number of suckers was counted and weighed for both fresh and dry weight for all plots. The tree circumference on five trees was measured at 30 cm above the soil surface at the time of sucker removal. The circumference was used to estimate tree cross-sectional area assuming the circumference was representative of a circle.
Soil water content probes (EC-5, Decagon Devices, Pullman, WA, USA) were installed ca. 60 cm from an emitter under the drip line at a depth of 30 cm in each of the 24 plots and interrogated weekly by hand. Four CR10X data loggers (Campbell Scientific, Logan, UT, USA) were in a 35 and a 100% replacement plot to monitor temperature (with thermocouples) at 0, 5, 10 and 30 cm depth and soil moisture (with EC-5 probes) at 5, 10 and 30 cm on an hourly basis in the two plastic and bare ground sub-plots. The temperature sensors were also located ca. 60 cm from emitters and centered along the tree row.
Light irradiance and reflectance measurements were made with a line quantum sensor (LI-191SA, LI-COR, Lincoln, NE, USA) and ground surface temperatures measured with a thermal infrared (IR) unit (model 210.2 L, Everest Interscience, Tucson, AZ, USA) on June 21, August 6 and September 19, 2013. Leaf greenness was measured on September 18, 2013 and on June 19, August 27, and September 22, 2014 with a Minolta 502 SPAD unit (Konica-Minolta, Ramsey, NJ, USA). Leaf stomatal conductance was measured on August 2, 2103 with a leaf porometer (Decagon Devices, Pullman, WA, USA). The light and IR measurements were taken around solar noon on summer solstice (June 21), then 46 and 90 days later in 2013 and on August 7 and 8, 2014.
The fruits were harvested in a single harvest by tree and sorted, counted, and weighed into four categories: prime, prime, superficial, cracked, and immature green fruit. The prime fruits were those that did not have any cracks, had very few blemishes, were at least 10 cm in diameter, had bright or dark red coloring, and they were targeted for the fresh market. The superficial fruits were those that had superficial cracks but no arils exposed, or had some surface blemishes, were smaller than 10 cm in diameter, and they were targeted for the juice market. The cracked fruits were those that had deep cracks with arils exposed, and they were thrown out regardless the size or color. The immature green fruits were those that had more than 50% green, and they were also thrown out. Total marketable included prime and superficial fruits and total weight included all fruits except immature green fruits.
Fruit Quality Analysis
Five fruits, selected randomally from the prime category for each treatment replication, were used for external and internal quality measurements. Four quadrants of the external equatorial fruit surface and internal juice color were measured with a Minolta CR-400 meter (Konica-Minolta, Japan) and L, a, b, chroma, hue, and hue angle were determined. Approximately 600 ml of arils were collected for use in characterizing the internal quality. Fruit sub-samples were stored at 4°C and processed within 1 month of harvest by replication sequence.
One-hundred random arils were counted from the 600 ml sample, weighed, juice expressed, and the expressed juice (about 16 to 20 ml) was spun at 2000 g for 10 min. at 25°C. Five ml of centrifuged juice was pipetted into a 30-mm plastic Petri dish, that was placed on a white surface (‘L’ value of 97.4, ‘a’ = −0.4, ‘b’ = 1.2) and then measured with a color difference meter (CR 400) for juice color attributes. During each reading, measuring head of the meter was placed 2–3 mm from the surface of the 5 ml juice sample. Sample absorbance was determined spectrophometrically at 516 nm after a 1:5 dilution in de-ionized water. Absorbency was measured within 25 seconds of adding water to dilute the solution. Soluble solids (%) were determined next with an Atago PR 32-α digital refractometer (Atago USA Inc., Kirkland, WA, USA). Juice pH was determined on the remaining sample. Two banks of lights (or 50% in the laboratory) directly above the CR-400 meter were turned off during all of the internal juice color measurements. Four replications were measured for each experimental treatment. Soluble solids (%) were determined next with an Atago PR 32-α digital refractometer (Atago USA Inc., Kirkland, WA, USA). Juice pH was determined on the remaining sample.
Data Analysis
Measured tree phenology and edaphic parameters, soil and environmental variables, and fruit yield and quality were evaluated against the treatment effects of ground cover (white plastic vs bare ground), irrigation rate, and their interactions. Treatment responses were tested using analysis of variance and Least Squares Means test of SAS (Ver. 9.1, SAS Institute, Cary, NC, USA).
Results and Discussion
Edaphic Measurements
Monthly weather data for maximum, minimum, and average air temperature are reported in , and cumulative reference evapotranspiration (ETo) and rain are reported in for 2013 to 2016. The data show that 2014 had higher mean monthly temperatures throughout the year than the remaining years with an average mean of 18.2°C, which also resulted in the highest ETo for 2013 to 2016. Potential evapotranspiration ranged from 1387 mm in 2015 to 1471.6 in 2014. The rainfall ranged from 81.5 mm in 2013 during the drought to 278.4 mm in 2016 that marked the end of the drought; however, the climate in the San Joaquin Valley is characterized as being Mediterranean with the majority of rainfall occurring during the winter (November – April) outside of the growing season. The rainfall during the winters prior to most growing seasons would have replenished the soil water in the crop root zone prior to budbreak.
Table 2. Average monthly maximum, minimum, and mean air temperatures for 2013, 2014, 2015, and 2016 in a multi-year pomegranate experiment conducted at the USDA-ARS San Joaquin Valley Agricultural Sciences Center, Parlier, California, U.S.A. Weather station was located approximately 1,000 m from research site
Table 3. Monthly reference evapotranspiration (ETo) and rainfall for 2013, 2014, 2015, and 2016 in a multi-year pomegranate experiment conducted at the USDA-ARS San Joaquin Valley Agricultural Sciences Center, Parlier, California, U.S.A. Weather station was located approximately 1,000 m from research site
The effect of the plastic on maintaining soil water content (SWC) over the irrigation season was evaluated in 2013 and 2014. In 2013, it was demonstrated that between June 6 and the termination of irrigation on November 25, water content at 30 cm depth was improved by use of the plastic cover compared to bare ground (). Irrigation at the 50 and 100% levels exhibited higher SWC values compared to the 35% irrigation level. In 2014, the plastic cover improved SWC in only the 100% irrigation treatment. Plastic covers improved soil moisture retention over the entire season compared with no plastic in both 2013 and 2014. The average water content at 30 cm depth was also plotted in and it shows clearly that an average of 0.02 m3/m3 higher SWC was consistently observed in the plastic covered versus the bare ground plots from 2013 to 2015. An exception occurred approximately July 31, 2014 when an excessive amount of water was accidently released to the bare ground plots because a pipe broke and flooded some of the bare soil plots, thus the bare soil had greater water content on average than the plastic covered soils. The differential was reduced after approximately a month with the bare ground having slightly higher water content than the plastic covered areas for the remainder of the season. The difference was eliminated in 2015 and the bare ground once again had lower soil water content.
Table 4. Effect of irrigation level and presence or absence of plastic covering on soil water content at 30 cm depth in 2013 and 2014 in a multi-year pomegranate experiment conducted at the USDA-ARS San Joaquin Valley Agricultural Sciences Center, Parlier, California, U.S.A
Figure 1. Average soil water content at 30 cm depth in a pomegranate orchard under bare ground or white plastic cover treatments averaged over three irrigation levels from 2013 to 2015
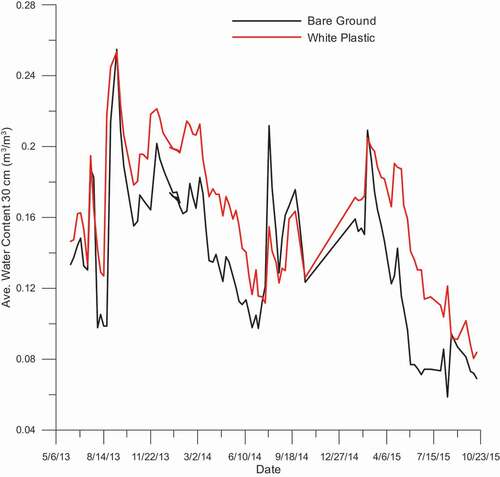
The effect of the white plastic film on soil temperatures was investigated in 2013 and 2014. The daily maximum and minimum soil temperatures recorded between May and December in both 2013 and 2014 showed a moderating effect of plastic covered vs. bare ground soil at depths between 0 and 30 cm (). Lower daily maximum and higher minimum temperatures were consistently recorded in the plastic than the bare ground soils. In addition, soil temperatures, averaged over all depths over a 193 day period in 2013, were 1.46°C higher under plastic compared to bare ground, and the average standard temperature deviation was reduced by ± 0.38°C under plastic. In 2014 the soil temperatures, averaged over a 289-day period from January 1 to October 16, were 1.27 °C higher under the plastic compared to bare ground, and the average standard deviation was reduced by ±1.01 °C under the plastic. When a similar white plastic material (T-65) was used for weed control at Lat. 26º N, similar soil temperature responses compared to bare ground were observed (Makus, Citation2010).
Figure 2. Daily maximum and minimum soil temperatures at 0, 5, 10 and 30 cm depths in a pomegranate orchard under bare ground (BG) or white plastic (WP) treatments with 35 and 100% irrigation levels in 2013 and 2014
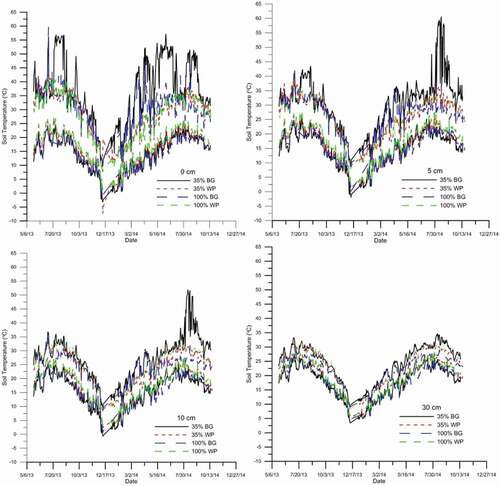
The effect of the white plastic film on reflected in-row orchard surface light and temperatures was investigated in 2013 and 2014. In 2013 as the sun’s azimuth changed over the summer in both unshaded and shaded areas beneath the canopy there was an impact on incident light, reflected light from the white plastic, and soil surface temperatures () with time. A gradual reduction in all these values was observed from the initial measurements on summer solstice (June 21). White plastic improved the per cent reflected light back into the canopy by 3.5-fold over the three measuring dates in 2013 but the situation was reversed in 2014. The percent reflected light from the white plastic declined from 20.3% to 18.8% between June and September 2013 but increased from 15.6% to 19.8% between June and July in 2014. White plastic reduced mid-day surface temperatures by over 10°C in full sun and 1.4°C in shade in 2013 and 5.5°C in shade and 1.3°C in the shade. Shaded surface temperatures were much lower than sunlit surface temperatures in both years. Increasing the irrigation replacement rate reduced the soil surface temperature. The date by cover interaction in 2013 resulted from a less dramatic response in temperature reduction due to the use of white plastic late in the season. All light measurements were on cloudless days. The measurement start time in June 2013 was about 1 hr. earlier than the other dates and probably negatively biased (lowered) the surface temperature readings for that date, although this starting time difference may have been compensated by the higher zenith sun angle in June. The date by cover interactions were not significant in 2014 because of the limited sampling period.
Table 5. Effect of measurement date, ground cover, and irrigation treatments on pomegranate in-row solar radiation exchange and ground surface temperature in 2013 and 2014 in a multi-year pomegranate experiment conducted at the USDA-ARS San Joaquin Valley Agricultural Sciences Center, Parlier, California, U.S.A. †
Phenological Measurements
Leaf greenness, as measured on September 18, 2013 was higher in leaves grown on trees with white plastic compared to leaves of trees grown without the plastic. No water replacement affects were observed, but leaf greenness was further reduced in leaves of trees grown on bare ground and supplied with irrigation at 100% water replacement, thus giving rise to the cover x irrigation level interaction (). The greenness was affected by the water replacement levels in 2014 with 35% replacement being paler than the other 2 replacement values. The greenness was not significantly different between cover treatments. Anecdotally, it was visually observed in mid-November 2013 that as leaves were senescing, those on trees grown over white plastic retained more of their greenness compared to those of the bare ground-grown leaves.
Table 6. Effect of irrigation level and presence or absence of plastic covering on pomegranate leaf greenness in 2013 and 2014 in a multi-year pomegranate experiment conducted at the USDA-ARS San Joaquin Valley Agricultural Sciences Center, Parlier, California, U.S.A. †
Tree circumference was measured on 6/25/13, 1/2/14, 10/6/14, 2/11/15, and 2/8/16; and is summarized in and . Response to water replacement level increased trunk cross-section area linearly with added water, both early in the growing season and at the end of the season in all years. The rate of increase in diameter differs across irrigation treatments. The average slope of the 35% is less than the 50% and the 100% treatment as well. A simple linear regression characterized rate of growth of the trunk for the 50% treatment as increasing 20% faster than the 35% treatment and the 100% as 58% faster than the 35% treatment. The increase in the diameter was 31% greater in the 100% treatment compared to the 50% treatment. This portion of the study was initiated after 3 years of growth and the differences in the cross-section area are apparent because of the early treatment. The rate of increase in trunk size is a function of the applied water with the rate increasing as the total applied water increases. For the same irrigation treatment, it is also quite evident that the placement of white plastic increased tree trunk growth, as compared to the bare ground control treatment.
Table 7. Average pomegranate tree trunk cross-sectional area in plots with different irrigation and ground cover treatments measured in 2013, 2014, 2015, and 2016 in a multi-year pomegranate experiment conducted at the USDA-ARS San Joaquin Valley Agricultural Sciences Center, Parlier, California, U.S.A. †
Figure 3. Pomegranate tree trunk cross-sectional area in plots under 35%, 50% or 100% irrigation treatments with or without plastic cover
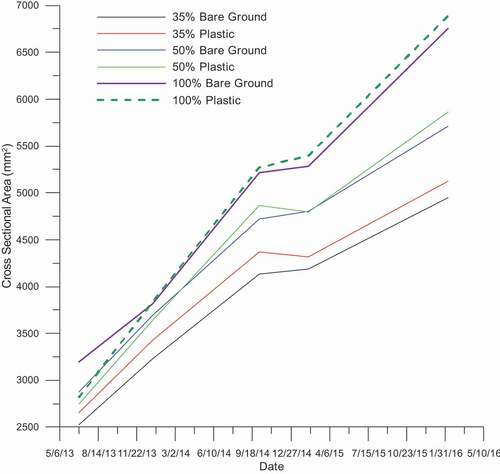
At the end of the season 2013, the percentage trees grown under white plastic were observed to have an increase in basal suckers, more suckers per tree, and larger sucker fresh weight (). The same effect of more suckers per tree and larger suckers with the plastic was also observed in the data from January 2015.
Table 8. Effect of ground cover and irrigation treatments on pomegranate tree basal suckering. Trees were pruned in December 2013 and January 2015 in a multi-year pomegranate experiment conducted at the USDA-ARS San Joaquin Valley Agricultural Sciences Center, Parlier, California, U.S.A. †
Fruit Yield
Yield data are reported in , for 2013, 2014, 2015, and 2016 for prime, superficial, cracked, immature green, total marketable, and total weight for plastic cover, bare ground, and the 35%, 50%, and 100% water replacement treatments. For prime fruit, there was no statistical difference in weight per tree from the plastic or irrigation treatments for the four years of study. The average prime fruit weight was not affected by the irrigation treatments until 2015 and 2016 when the 100% treatment was larger than the remaining treatments. The placement of plastic cover increased the size of the prime fruit in 2016 but not in 2013–2015. Total marketable fruit weight per tree was also higher for the plastic treatment in 2014 and 2016 as compared to the bare ground or under 100% irrigation as compared to deficit irrigation treatments, There was a difference in the total weight between the cover treatments in all years with the plastic cover having the larger total yields. Except for 2013 there were differences in the total yields between the replacement treatments with the 35% treatment differing from the remaining treatments. The total yield in the 35% and 50% treatments were statistically the same in 2015 and 2016 and the 50% and 100% treatments were statistically the same in 2013 and 2014. However, in each year the actual yield increased with increasing rates of water replenishment. The differences in total and marketable are a result of increased cracking and immature green fruit as the water replacement increases. In the cultivar Mollar de Elche, increasing water deficits reduced main harvest yields and fruit size (Mellisho et al., Citation2012). The flowering of ‘Wonderful’ tends to be precocious when temperatures are moderate and trees will flower and new fruit develop late in the season and not come to maturity.
Table 9. Effect of ground cover and irrigation treatment on pomegranate yield attributes in 2013 in a multi-year pomegranate experiment conducted at the USDA-ARS San Joaquin Valley Agricultural Sciences Center, Parlier, California, U.S.A. †
Table 10. Effect of ground cover and irrigation treatment on pomegranate yield attributes in 2014 in a multi-year pomegranate experiment conducted at the USDA-ARS San Joaquin Valley Agricultural Sciences Center, Parlier, California, U.S.A. †
Table 11. Effect of ground cover and irrigation treatment on pomegranate yield attributes in 2015 in a multi-year pomegranate experiment conducted at the USDA-ARS San Joaquin Valley Agricultural Sciences Center, Parlier, California, U.S.A. †
Table 12. Effect of ground cover and irrigation treatment on pomegranate yield attributes in 2016 in a multi-year pomegranate experiment conducted at the USDA-ARS San Joaquin Valley Agricultural Sciences Center, Parlier, California, U.S.A. †
Fruit Quality
Fruit external color was not affected by either water application rate or the use of white plastic (data not shown). Deficit irrigations with ‘Mollar de Elche’ also did not influence peel lightness values (Mellisho et al., Citation2012). Plastic cover or increasing water application rates had no effect on aril weight, and juice pH but increased soluble solids at 50% and 100% irrigation rate in 2014 (). Except for the “L” value in 2013, plastic use did not change juice internal objective color attributes as compared to the bare ground treatment. However, there was a response of irrigation levels on juice internal objective color attributes. The L, b, hue angle, and absorbance values in 2104 were affected by irrigation replacement with the 35% being significantly different that the remaining treatments. These differences suggest that the 35% treatment results in darker fruit. External color measurements were not good predictors of juice raw product attributes such as pH, soluble solids, and spectrometric absorbance at 516 nm, compared to internal measurements of the expressed juice (). Drogoudi et al. (Citation2005) found no significant correlations among pomegranate fruit of 2 l accessions and their seed color parameters, suggesting that external color does not reflect internal color attributes. Borochov-Neori et al. (Citation2009) found juice anti-oxidative capacity to be correlated with soluble phenolics (R2 = 0.98), but not with red color intensity (R2 = 0.38) in 11 cultivars. Since fruit appearance was not closely correlated to juice antioxidants, they suggested that changes in agricultural production practices, to include climate management, would bring fruit quality and appearance attributes closer together. Wetztein et al. (Citation2011) reported that fruit size and arils per fruit, but not average aril weight, were highly correlated in ‘Wonderful’. Mena et al. (Citation2012) reported that deficit irrigations of 43 and 12% of ETo resulted in a dramatic decrease in bioactive phenolic compounds resulting in weaker red juice and fruit color. Raw product attributes (SS%, titratable acidity, pH) were relatively unchanged in ‘Mollar de Elche’ due to water replacement rates. Mellisho et al. (Citation2012) found similar raw product results, with aril total anthocyanin contents lowest in the most extreme deficit treatment. Mid-summer and late season deficit irrigations were found to modify fruit composition with respect to SS (%) and bioactive compounds and generally improved weight loss and peel pitting after 8 and 19 weeks in storage in ‘Mollar de Elche’ (Laribi et al., Citation2013).
Table 13. Effect of ground cover and irrigation treatment on pomegranate fruit quality attributes in 2013 in a multi-year pomegranate experiment conducted at the USDA-ARS San Joaquin Valley Agricultural Sciences Center, Parlier, California, U.S.A. †
Table 14. Effect of ground cover and irrigation treatment on pomegranate fruit quality attributes in 2014 in a multi-year pomegranate experiment conducted at the USDA-ARS San Joaquin Valley Agricultural Sciences Center, Parlier, California, U.S.A. †
Table 15. Correlations between pomegranate internal juice objective color, external fruit color, and juice raw product attributes in a multi-year pomegranate experiment conducted at the USDA-ARS San Joaquin Valley Agricultural Sciences Center, Parlier, California, U.S.A. †
Weed Control
There were four paraquat + surfactant applications for weed control in 2013 and the season costs were estimated at US 324 USD/ha for chemicals and 8 hrs/ha required for tractor spraying labor. Estimated manual with-in-row weed control would require 58 man-hrs/ha per weeding event. The cost of the plastic was 1.72 USD/m2; 4,388 m2 would be required per hectare for a 2.1 m in-row width, secured at both ends to a depth of 15 cm. Plastic life is estimated to be at least 5 years. A similar plastic (Reef Industries T-65) was used in a weed control experiment with peaches at Lat. 26º N and was found to be in excellent condition at the termination of the test after 4 years in the field (Makus and Jifon, Citation2007). The estimated average yearly relative weed control costs prorated over seven years were: hand ≫ plastic > chemical. Major weeds observed in the bare ground plots were Conyza spp., Erodium spp., Malva spp., and Poa annua.
Conclusions
Application of a white plastic to a young pomegranate orchard in the fourth leaf negated the use of herbicides for in-row weed control, improved initial orchard floor reflectance 3.5 fold, which resulted in cooler mid-day soil surface temperatures and enhanced leaf greenness. Soil moisture retention and uniformity was improved, resulting in greater trunk circumference compared with trees grown without a plastic cover. Total yield of all fruit categories was improved by 20 to 29%, suggesting that the reflecting plastic improved plant productivity through increased light reflectance into the lower canopy, soil temperature and moisture modulation, and accelerated fruit maturation. Fruit juice color was generally not affected by plastic cover or water replacement rates. In summary, tree performance was improved by both a reflective plastic cover and irrigating at 100% of the water removed by the crop.
References
- Ayars, J.E., C.J. Phene, R.C. Phene, S. Gao, D. Wang, K.R. Day, and D.J. Makus. 2017. Determining pomegranate water and nitrogen requirements with drip irrigation. Agric. Water Manage. 187:11–23. doi: https://doi.org/10.1016/j.agwat.2017.03.007.
- Bonanno, A.R. 1996. Weed management in plasticulture. HortTechnol 6(3):186–189. doi: https://doi.org/10.21273/HORTTECH.6.3.186.
- Borochov-Neori, H., S. Judeinstein, E. Tripler, M. Harari, A. Greenberg, I. Shomer, and D. Holland. 2009. Seasonal and cultivar variations in antioxidant and sensory quality of pomegranate (Puncia granatum L.) fruit. J. Food Comp. Anal. 22:189–195. doi: https://doi.org/10.1016/j.jfca.2008.10.011.
- Drogoudi, P.D., C. Tsipouridis, and Z. Michailidis. 2005. Physical and chemical characteristics of pomegranates. HortScience 40(5):1200–1203. doi: https://doi.org/10.21273/HORTSCI.40.5.1200.
- Glenn, D.M., and G.J. Puterka. 2007. The use of plastic films and sprayable particle films to increase light penetration in apple canopies and improve apple color and weight. HortScience 42(1):92–96. doi: https://doi.org/10.21273/HORTSCI.42.1.91.
- Iglesias, I., and S. Alegre. 2009. The effects of reflective film on fruit color, quality, canopy light distribution, and profitability of ‘Mondial Gala’apples. HortTechnology 19(3):488–498. doi: https://doi.org/10.21273/HORTSCI.19.3.488.
- Intrigliolo, D.S., E. Nicolas, L. Bonet, P. Ferrer, J.J. Alarcon, and J. Bartual. 2011. Water relations of field grown pomegranate trees (Puncia granatum) under different drip irrigation regimes. Agric. Water Manage. 98:691–696. doi: https://doi.org/10.1016/j.agwat.2010.11.006.
- Intrigliolo, D.S., D. Wang, M.B. Pérez-Gago, L. Palou, J. Ayars, H. Puerto, and J. Bartual. 2020. Chapter 10. Pomegranate water requirements and responses to irrigation restrictions, p. 320–343. In: A. Sarkhosh, A.M. Yavari, and Z. Zamani (eds.). The pomegranate: Botany, production and uses. Wallingford, Oxfordshire, UK: CABI.
- Jakopic, J., F. Stamper, and R. Veberic. 2010. Influence of hail net and reflective foil on cyaniding glycosides and quercetin glycosides in ‘Fuji’ apple skin. HortScience 45(10):1447–1452. doi: https://doi.org/10.21273/HORTSCI.45.10.1447.
- Kasirajan, S., and M. Ngouajio. 2012. Polyethylene and biodegradable mulches for agricultural applications: A review. Agron. Sustainable Dev. 32(2):501–529.
- Laribi, A.I., L. Palou, D.S. Intrigliolo, P.A. Nortes, C. Roja-Argudo, V. Taberner, J. Bartual, and M.B. Perez-Gago. 2013. Effect of sustained and regulated deficit irrigation on fruit quality of pomegranate cv. ‘Mollar de Elche’ at harvest and during cold storage. Agric. Water Manage. 125:61–70. doi: https://doi.org/10.1016/j.agwat.2013.04.009.
- Layne, D.R., Z. Jiang, and J.W. Rushing. 2001. Tree fruit reflective film improves red skin coloration and advances maturity in peach. HortTechnology 11(2):234–242. doi: https://doi.org/10.21273/HORTTECH.11.2.234.
- Legua, P., P. Melgarejo, J.J. Martinez, R. Martinez, and F. Hernandez. 2012. Evaluation of Spanish pomegranates juices: Organic acids, sugars, and anthocyanins. Int. J. Food Prop. 15(3):481–494. doi: https://doi.org/10.1080/10942912.2010.491931.
- Makus, D.J. 2010. Use of synthetic ground covers to control weeds in blackberries. Int. J. Fruit Sci. 11(3):1–13.
- Makus, D.J. 2011. Weed control and canopy light management in blackberries. Int. J. Fruit Sci. 10(2):177–186. doi: https://doi.org/10.1080/15538362.2010.492335.
- Makus, D.J., and J.L. Jifon. 2007. Impact of weed barriers on newly planted peach trees. HortScience 42(3):456–457. (Abstract).
- Mellisho, C.D., I. Egea, A. Galindo, P. Rodriguez, J. Rodriguez, W. Conejero, F. Romojaro, and A. Torrecillas. 2012. Pomegranate (Punica granatum L.) fruit response to different deficit irrigation conditions. Agric. Water Manage. 114:30–36. doi: https://doi.org/10.1016/j.agwat.2012.06.010.
- Mena, P., A. Galindo, J. Collado-Gonzalez, S. Ondono, C. Garcia-Viguera, F. Ferreres, A. Torrecillas, and A. Gil-Izquierdo. 2012. Sustained deficit irrigation affects the colour and phytochemical characteristics of pomegranate juice. J. Sci. Food Agric. 93:1922–1927. doi: https://doi.org/10.1002/jsfa.5991.
- Prive, J.P., L. Russel, and A. Leblanc. 2008. Use of extenday reflective groundcover in production of ‘Gala’ apples (Malus domestica) in New Brunswick, Canada: 1. Impact on canopy microclimate and leaf gas exchange. N. Z. J. Crop. Hort Sci. 36(4):221–231. doi: https://doi.org/10.1080/01140670809510238.
- Schneider, A.D., J.E. Ayars, and C.J. Phene. 1996. Combining monolithic and repacked soil tanks for lysimeter high water table sites. Appl. Eng. Agric. 12(6):649–654. doi: https://doi.org/10.13031/2013.25694.
- Smith, M., and P. Steduto. 2012. Yield response to water: The original FAO water production function. FAO Irrigation and Drainage Paper 2012 No.66, p.6–13 ref.13.
- Sun, H., L. Shao, X. Liu, W. Miao, S. Chen, and X. Zhang. 2012. Determination of water consumption and the water-saving potential of three mulching methods in a jujube orchard. Eur. J. Agron. 43:87–95. doi: https://doi.org/10.1016/j.eja.2012.05.007.
- Wang, D., J.E. Ayars, R. Tirado-Corbala, D. Makus, C.J. Phene, and R. Phene. 2015. Water and nitrogen management of young and maturing pomegranate trees. Acta Hortic. 1089:395–401. doi: https://doi.org/10.17660/ActaHortic.2015.1089.53.
- Wetztein, H.Y., Z. Zhang, N. Ravid, and M.E. Wetztein. 2011. Characterization of attributes related to fruit size in pomegranate. HortScience 46(6):908–912. doi: https://doi.org/10.21273/HORTSCI.46.6.908.
- Yuan, Z.H., E. Wilkins, and D. Wang. 2015. Proceedings of the Third International Symposium on Pomegranate and Minor Mediterranean Fruits. Leuven, Belgium: International Society of Horticultural Science.
- Zhang, H.H., D. Wang, J.E. Ayars, and C.J. Phene. 2017. Biophysical response of young pomegranate trees to surface and sub-surface drip irrigation and deficit irrigation. Irrig. Sci. 35:425–435. doi: https://doi.org/10.1007/s00271-017-0551-y.