ABSTRACT
After harvest, higher respiration rate in apricots limit their postharvest with extensive losses in fruit quality. Therefore, effect of different pre-storage treatment of modified atmosphere packaging (MAP) were studied on the shelf life and quality of apricot cv. ‘Shahroudi’ fruit. Experimental treatments were control (air), and active MAP includes: MAP1 (10% O2/20% CO2), MAP2 (20% O2/10% CO2), MAP3 (5% O2/80% CO2), MAP4 (80% O2/5% CO2) and MAP5 (40% O2/20% CO2), all treatments were balanced by N2. After 6 days of pre-storage treatments, fruits were unpacked, repacked and then were stored at 2°C and 85% relative humidity for 28 days. Results indicated that MAP5 and MAP3 treatments significantly reduced weight loss and total soluble solids, and maintained fruit hardness in comparison with other treatments. High CO2 treatment improved postharvest traits of apricot. Interestingly, high CO2 in combination with high O2 (active MAP5) improved the sensory evaluation as scored the best treatment by panelists, and also maintained better chemical properties such as total phenolic compounds, total carotenoids, and vitamin C. In addition, shelf life of fruit in active MAP5 (29.6 days) significantly extended followed by active MAP3 (27.6 days), compared to control (14.6 days) without signs of decay until the end of the storage period. Overall, it can be concluded that the pre-storage treatment of fresh apricot fruit in an atmosphere with high O2 (40%) and CO2 (20%) concentrations had a positive effect on the post-harvest quality attributes and shelf life of apricot cultivar ‘Shahroudi,’ and can be suggested as an effective and practical method to prolong its postharvest life.
Introduction
Apricot (Prunus armeniaca L.) is an important temperate delicious fruit with high nutritional value. The fruit is rich in a type of carotenoid that precedes vitamin A (beta-carotene) in our body, and it affects the health of the eyes and the human heart (Davarynejad et al., Citation2010). However, thin skin of fruit and its soft flesh makes it susceptible to physical damage, which leads to subsequent postharvest losses. Besides, apricot is a climacteric fruit, so the process of ripening is regulated by ethylene and after harvest, the ripening process is fast, which leads to limited shelf life. Therefore, the inhibition of ethylene biosynthesis by the modified atmosphere and the consequent delay of the ripening process could extend storage-life (Moradinezhad and Jahani, Citation2019).
Controlled and modified atmosphere storage is used to extend the fruit postharvest life and seasonal availability mainly by slowing down the respiration rate and inhibiting some pathogens development during cold storage. However, optimal atmospheric modifications may vary greatly among different fruit cultivars (Manganaris and Crisosto, Citation2020). Furthermore, its potential as an alternative to using postharvest chemicals is a subject of high interest (Mitcham and Elkins, Citation2007). But the economic implications of using this comparatively expensive technology (controlled atmosphere) have often limited its commercial application. Therefore, modified atmosphere packaging for shorter period attracted the attention of researchers during the last decade probably due to the fact that it may have the similar impact on fruit quality and shelf life and also with lower cost than controlled atmosphere (Yahia, Citation2009).
The impact of enriched atmospheres with high CO2 and high O2 was evaluated in different fruits (Moradinezhad et al., Citation2020; Moradinezhad and Dorostkar, Citation2020, Citation2021; Pérez and Sanz, Citation2001). Storage of minimally processed strawberries fruits in high O2 and high CO2 atmospheres significantly inhibited microbial growth compared to the control (Pérez and Sanz, Citation2001). Also, pre-storage high CO2 shock treatment for short period reduced the fruit decay in pomegranate fruits during cold storage (Moradinezhad et al., Citation2018).
Similarly, previous studies have indicated positive impact of modified atmosphere packaging in quality and shelf life of fresh apricot fruit. It has been shown that active MAP (5% O2 and 10% CO2) preserved the physical and sensorial qualities of apricots during 28 days of cold storage compared to passive MAP, while of control (air) was less than seven days with significant changes in physical and sensory properties (Muftuoğlu et al., Citation2012). Recently, a study showed that the use of passive MAP effectively reduced postharvest losses of apricot fruits, and improved the qualitative and sensory characteristics of the fruit stored at 0.5°C for 28 days (Moradinezhad and Jahani, Citation2019). However, research on pre-storage active packaging includes different concentrations of respiratory gases on quality attributes and extending the shelf life of apricot fruits particularly on ‘Shahroudi’ cultivar, has not been adequately assessed. Therefore, the aims of this work were to study the effect of different modified atmosphere compositions pre-storage treatment on the quality properties of apricot fruit ‘Shahroudi’ cultivar as well as to determine the optimum gases composition (O2/ CO2) to prolong the fruit shelf life during cold storage.
Materials and methods
Preparation of the Fruit
In this experiment, 10-year-old apricot trees (cv. Shahroudi) were used. Irrigation was once every 14 days, and fertilization was given to the trees as a complete fertilizer. Fruit were harvested from a commercial orchard at Birjand, South Khorasan province, Iran, on May 30, 2018. Fruit was picked at full mature stage with light green color background and yellow spots, and total soluble solids approximately 10% (). The fruits were then transferred to the Postharvest Laboratory, University of Birjand. For pre-cooling, the fruits were stored in the refrigerator for 24 h at 2°C with 85 ± 5% relative humidity. Thereafter, about 250 uniform and free of defects fruits with an average weight of 55 ± 5 g was selected.
Table 1. Physical and chemical properties of apricot fruit used at harvest.
Packaging
Fruit were transferred to 30 × 40 cm low-density polyethylene (LDPE) bags (0.06 mm thickness). Gas and moisture transmissions properties of the LDPE bag were: permeation to oxygen (O2) 15–30 (cm3/m2/day), permeation to carbon dioxide (CO2) 60–160 (cm3/m2/day), and WVTR 5–10 (g/m2/day). Three bags were used for each treatment and 20 fruits (approximately 1 kg) were placed in each bag. According to the number of replications (3) and treatment (6), 360 fruits were used for this experiment. Also, for more accuracy in evaluating the shelf-life, separate replications were considered. For each replication, 8 fruits were prepared (replication×treatment×8) and, 144 fruits were considered to evaluate the shelf-life. A total of 504 fruits were required for this experiment. The bags were vacuumed using a vacuum pump and were then flushed with different gas compounds (MAP1, MAP2, MAP3, MAP4, and MAP5) and then sealed using a Manual Heat Sealer, except for control fruit that were remained unpacked (slightly open) (). The bags were then placed in a cool room for six days at 2°C and 85 ± 5% relative humidity; so that the effects of the treatments were quite clear during this time (6 days). After that, the apricots were unpacked and placed in polypropylene (PP) containers with 700 ml volume and dimensions of (7 × 9 × 12 cm, and 0.2 mm thickness) (packed with its lid), and stored in a cold room at 2°C with 85 ± 5% relative humidity for 28 days.
Table 2. Pre-storage treatments applied on apricot fruit at 2°C for 6 days†.
Temperature and relative humidity were recorded during the storage period by a digital data logger (Extech Instrument, Model RHT 20, humidity and temperature data logger, USA). At the end of the storage period (28 days), physicochemical and sensory evaluation parameters of fruit were measured.
Gas Analysis inside Packages
The gas injection was performed using calibrated syringes and after each injection, the gas concentration inside the package was carefully measured to achieve the desired gas composition. Gas composition inside the packages was determined using a gas analyzer (OXYBABY 6.0+ CO2/O2/N2 Gas Analyzer, Germany). The gas analysis was performed by inserting a needle attached to the gas analyzer through an adhesive seal fixed on the lidding material. Changes in the composition of respiratory gases (O2, CO2 and N2) in the sealed polyethylene bags were measured using the gas analyzer in 2 days intervals.
Determination of Weight Loss and Hardness
After 28 days of storage, fruit weight loss and hardness were measured using a digital balance and a digital penetrometer (FHT200, Extech Co., USA) fitted with a 2 mm probe, respectively as described in Moradinezhad and Jahani (Citation2016). Weight loss was expressed as a percentage and tissue hardness in Newtons.
Determination of Total Soluble Solids (TSS), Total Phenol Contents (TPC), Total Carotenoids (TC), and Vitamin C
To determine the chemical properties of apricot fruit, three samples were used to provide fruit juice in each replicate. TSS of juice was measured using a hand-held refractometer (RF 10, Brix, 0–32%, Extech Co., USA), and data were expressed as percent (%). TPC was determined using the Folin-Ciocalteau method (Chuah et al., Citation2008). The TPC was expressed as (GAE)/ 100 g of fresh apricot. The carotenoid values were determined using the Arnon method (Arnon, Citation1967). Vitamin C content was determined using 2, 6-dichlorophenol indophenols (Nielsen, Citation2010), and was expressed as mg/100 g of fresh weight.
Determination of Decay, Shelf Life, and Sensory Evaluation
The decay was visually evaluated during the storage time. Fruits were examined daily and considered infected when a visible lesion was observed (such as, surface mycelia, slimy patches, bruises, and blemishes). Results were expressed as the percentage of infected fruits in each replicate. To determine the shelf life (storage life) in cold storage conditions (at 2°C with 85 ± 5% relative humidity), separate replications were considered and all fruits were assessed daily. Eventually, the shelf life was based on the physical appearance of the fruit as judged by the retention of freshness, color, and glossy appearance of fruit without any desiccation, and was expressed as day (Moradinezhad and Jahani, Citation2016). To evaluate the taste and appearance of apricot fruits a five-point scoring test was performed by ten trained panelists at the end of the storage period. The panelists aged between 24 and 40 years (average age of 29.5, 4 males and 6 females). A score of 5 was rated as very good (high-quality fruit and without defects), and a score of 1 was calculated as very bad (unpleasant, and off-flavor). Score 3 was determined as an acceptable quality level.
Experimental Design and Statistical Analysis
In this experiment, separate replications were used to check the shelf life of the fruits, and the evaluation continued until the best fruits began to decline in quality. But other traits (other than shelf life) were assessed on day 28 of the experiment.
The experiment was conducted using a completely randomized design with six treatments. Three replicates were used for each treatment, and all results (except, sensory evaluation data) were expressed as mean ± standard error (SE). Data were subjected to analysis of variance (ANOVA). All analyses were performed using the GenStat program (version 9.2, 2009, VSN, International, UK). The LSD’s test at the level of 1% probability (P ≤ .01) was also used to identify the significant differences between the means.
Results and Discussion
Changes in O2 and CO2 Concentrations
The changes in O2 and CO2 levels of apricot packages were monitored every two days. shows that in the control samples, the space around the fruit was air (21% O2/0.03% CO2). In MAP1, O2 levels increased slightly, while CO2 levels decreased from 20% to 12%. In MAP2 treatment, both O2 and CO2 were reduced. Interestingly, the concentration of O2 and CO2 in the treatment of MAP1 and MAP2 were almost the same. In MAP3, which was rich in CO2, the concentration of CO2 dramatically decreased, so that the amount of CO2 decreased from 80% to 20%. In O2-rich MAP4 treatment the O2 concentration decreased from 80% to 25%. These changes could be explained by the fact that the permeability of CO2 is three to four times higher than O2 in polyethylene packages (Siracusa, Citation2012). On the other hand, the increase in nitrogen gas levels in the packages indicates the entry of this gas into the package. The concentration of gases in the MAP5 gradually decreased so that the O2 content decreased from 40% to 30% and the CO2 from 20% to 12%. It is recognized that steady-state CO2 and O2 levels inside a package mainly depend on product respiration rate and film permeability (González et al., Citation2008).
Weight Loss
Fruit weight loss was significantly (P ≤ .01) affected by different active packaging treatments during storage. After 28 days of cold storage, the lowest weight loss was obtained in MAP3, followed by MAP5 samples compared to control samples. However, there was no significant difference between MAP3 and MAP5 treatments ().
Figure 2. Differences in weight loss (a), hardness (b), decay (c) and shelf life (d) of fresh apricot in different MAP treatments after 28 days of storage at 2 °C. Error bars represent the standard error deviation.
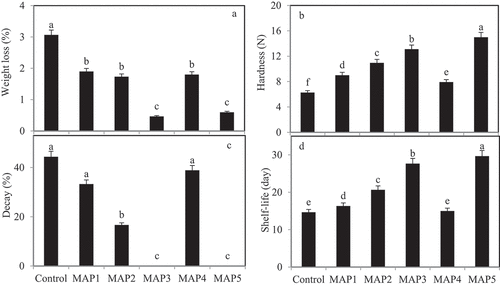
Water loss is one of the most important causes of weight loss, which reduces fruit quality and marketability (Manganaris et al., Citation2007). It is proven that in the process of respiration, glucose is consumed and carbon dioxide, water and energy are produced. If environmental conditions (temperature, CO2, O2, etc.) and internal conditions (ethylene, organic acid, etc.) increase respiration, the production of the respiratory cycle will also increase. Therefore, the product loses a lot of water, which leads to weight loss. On the other hand, the rate of respiration in climacteric fruits such as apricots is increased by ripening, which increases weight loss (Moradinezhad and Jahani, Citation2016). The results showed that apricot weight remained stable during storage under MAP3 and MAP5 treatments, whereas control samples showed an average weight loss of about 6–fold higher than MAP3 and MAP5 samples on days 28. It could be related to the effect of high CO2 shock in MAP3 or high CO2 with high O2 in MAP5. Álvarez-Hernández et al. (Citation2020) reported that apricot fruit weight loss was significantly reduced under passive MAP compared with air during cold storage. Also, the results showed that other MAP treatments (MAP1, MAP2 and MAP4) had less weight loss than the control. The lower weight loss of MAP fruit is related to a less water loss, as there is lower water vapor–pressure deficit in MAP compared to air (Thompson et al., Citation2018). The reduction in weight loss might be due to the barrier of moisture such as type of film and gas concentration, which can retard ripening, ethylene production, and respiration rate during storage. Our results were consistent with the results of Moradinezhad and Jahani (Citation2019) on apricot fruit.
Hardness
shows the effect of the MAP pre-treatments on hardness after 28 days of storage. According to , the hardness on the day of harvest was 18.24 N. Overall, decrease in fruit hardness was observed in all treatments compared at harvest. Nevertheless, the fruit treated with MAP5 (15 N) had the highest amount of tissue hardness, followed by MAP3 (13.11 N) and MAP2 (10.95 N). Also, the lowest amount of hardness was obtained from control (6.27 N) and MAP4 treatment (7.92 N).
Various studies have found that high carbon dioxide in cold storage has a significant effect on maintaining the hardness of fruits such as strawberries (Bang et al., Citation2019) and peaches (Belge et al., Citation2019). High carbon dioxide inhibits ethylene production and delays ripening, but importantly, the tissue sensitivity of different fruits to carbon dioxide varies to some extent (Kader and Watkins, Citation2000). A recent study showed that atmosphere containing a combination of high carbon dioxide and high oxygen reduces tissue susceptibility to high levels of carbon dioxide and also has little adverse effects of high carbon dioxide alone application (Van de Velde et al., Citation2019). The results showed that in the MAP5 treatment, CO2 (20%) with O2 (40%) delayed the softening process. Similarly, in a study showed that high CO2 with high O2 (10% CO2/90% O2 or 20% CO2/80% O2) maintained tissue hardness of strawberries stored at 8°C for 9 days compared to the control (Pérez and Sanz, Citation2001). They have speculated that the mechanism for CO2-induced hardness enhancement in strawberry is due to changes in the pH of the apoplast that could promote precipitation of soluble pectins. The maintenance of constant activity of cell wall hydrolyzing enzymes has been proposed as the key factor explaining hardness retention in high-O2-stored berries (Pérez and Sanz, Citation2001).
Total Soluble Solids
The TSS of active MAP fruits was significantly (P ≤ .01) lower than control (), so that the highest soluble solids content was showed in control (17.67%) and the lowest in MAP5 treatment (11.33%).
Table 3. Effect of different pre-storage MAP treatments on total soluble solids (TSS), total phenolic compound (TPC), total carotenoids (TC), vitamin C, and sensory evaluation of apricot fruit after 28 days of storage at 2 °C.
One of the important changes that occur during fruit ripening is the hydrolysis of starch to sugar and conversion of insoluble propectins into soluble pectins, which changes the taste and texture of the fruit. Likely any treatment that delays ripening will prevent the conversion of carbohydrates like starch to sugars (Fan et al., Citation2019). Studies have shown that high carbon dioxide inhibits the activity of ethylene by binding to ethylene receptors (Kader and Saltveit, Citation2003). We have found that the combined application of high CO2 with high O2 (MAP5) is more effective than the individual use of these gases to maintain the total soluble solids. It seems that MAP5 treatment reduced the respiration rate of fruit and resulting in lower TSS. However, in control samples, TSS was higher due to increased respiration, and fruit ripening. Also, our results confirm those of other researchers that the TSS of strawberries in 70% O2 + 20% CO2 and 90% O2 + 10% CO2 atmospheres are significantly reduced after 20 days of storage (Van de Velde et al., Citation2019).
Total Phenolic Compound (TPC)
TPC in all active MAP treatments significantly increased compared to the control. As shown in the , the highest amount of TPC was obtained from MAP3 treatment (63.67 GAE/ 100 g), whereas the lowest amount of TPC observed in the control (62.29 GAE/ 100 g). Also, TPC values maintained in MAP5 (63.13 GAE/ 100 g) and MAP2 (63.04 GAE/ 100 g) treatments.
Phenolic compounds are very valuable for human health, and affect the taste, aroma and appearance of products (Ballard and Junior, Citation2019). These compounds are known as antioxidants and inhibitors of free radicals. Increased free radicals during the ripening process of fruits cause damage and increase senescence (Ballard and Junior, Citation2019). Tissue oxygen restriction and increased carbon dioxide both reduce respiration rate (disrupting ethylene synthesis). It has been suggested that by reducing the rate of respiration, the amount of carbohydrate consumed in respiration is also reduced and carbohydrates accumulate in the tissue, the accumulated carbohydrate is used to produce phenolic compounds (Wang et al., Citation2017). Authors reported that high CO2 (20%) in cherimoya fruit increases synthesis of phenylalanine ammonia-lyase enzyme (PAL), which is the first phase in the making of phenolic compounds (Assis et al., Citation2001). The results of current study showed that MAP3 (CO2, 80%), MAP5 (CO2, 20%) and MAP2 (CO2, 10%) treatments had the highest TPC levels, respectively. This increase in the amount of phenol content may be due to the fact that high CO2 concentration could induce abiotic stress, which increases phenolic compounds in plants (as a resistance mechanism). Researchers in a study also showed that TPC in mango fruits was higher in high carbon dioxide (10%) and low O2 (5%), which is in agreement with our results (Kim et al., Citation2007). But it must also be taken into account that plant phenolic compounds are depended on genetic potential and environmental factors during growing and postharvest.
Total Carotenoid (TC)
shows the effect of MAP on total carotenoid content of cold stored apricot fruit. The amount of TC at harvest time was reported to be 0.87 mg/g (). However, the results show that after 28 days of storage, the TC value has increased in all treatments compared to harvest. Carotenoid biosynthesis continues after harvest in fruits. The highest amount of TC was obtained from MAP5 treatment (7.5 mg/g) followed by MAP3 (4.67 mg/g) and MAP1 (3.87 mg/g) treatments. The lowest amount of TC was recorded in MAP4 treatment (0.9 mg/g). Also, the results showed that there was no significant difference between MAP4 treatment and control.
The carotenoids are pigments responsible for the creation of red, yellow and orange colors in fruits and leaves. In addition to the importance of carotenoids, which are related to vitamin A and its effect on animal vision. Also, its importance in plants has been determined (Mandić et al., Citation2021). Researchers believe that carotenoids absorb light wavelengths that cause chlorophyll optical oxidation, preserving chlorophyll and reducing damage (Sun et al., Citation2020). Environment factors such as oxygen level, temperature, and light affect carotenoid oxidative degradation (Sun et al., Citation2020). Non-enzymatic oxidation of carotenoids by reactive oxygen species oxidizes carotenoids (Mapelli-Brahm et al., Citation2020). An important factor in the synthesis of carotenoids is oxygen (Mapelli-Brahm et al., Citation2020). The results showed that apricots treated with 40% oxygen (MAP5) had the highest TC. However, if the oxygen in the plant tissue is high to cause free radicals, the carotenoids in the tissue are used to remove the oxygen-free radical. Therefore, the amount of TC in tissue is reduced. Similar results have been reported for TC in apricot fruit using modified atmosphere (5% O2 and 10% CO2) (Ayhan et al., Citation2009). They stated that increased carotenoid content was probably due to the prevention of oxidative stress in the presence of high CO2.
Vitamin C Content
MAP5 treatment had a significant (P ≤ .01) effect on vitamin C of apricot fruit (). The results showed that vitamin C content significantly increased about fivefold in MAP5 compared to the control. However, there was no significant (P ≥ .05) difference between control, MAP3 and MAP4. Research has shown that high amounts of carbon dioxide (10%) or high O2 (80%) increase the vitamin C content of strawberries (ascorbic acid) in the first 5 days of storage (Allende et al., Citation2007). Authors, also reported that high CO2 (10%) increased ascorbic acid in strawberries, but higher levels of this gas (more than 30%) synthesize the ascorbate peroxidase enzyme, which results in ascorbic acid oxidation, they also observed that on the seventh day of the storage, high concentrations of O2 (30%) increased the ascorbic acid value in the strawberries, but decreased gradually until the last day of storage (Agar et al., Citation1997). Respiration rate increases with increasing O2 content and then organic acids (like ascorbic acid) are used as respiratory substrates, and consequently, their contents in fruit tissue decreases. Researchers stated that the decline of vitamin C was due to the consumption of ascorbic acid as an antioxidant to reduce free radicals and oxidative stress (caused by high O2) (Pérez and Sanz, Citation2001).
Decay
Decay is a major cause of postharvest fruit losses mainly due to fungal infections. All MAP treatments had a significant (P ≤ .01) effect on the decay percentage of apricot fruit stored at 2°C for 28 days. As shown in all treatments except MAP4 significantly reduced decay percentage compared to control. Interestingly, no decay was observed in MAP3 and MAP5 treatments. It has been reported that high concentrations of CO2 activate the cytoplasmic pH, which inhibits microorganisms and prevents the decay (Van de Velde et al., Citation2020). In our recent study, we reported that high concentration of carbon dioxide (20%) controlled decay in pomegranates (Moradinezhad et al., Citation2018). In another study, researchers also stated that elevated O2 above ambient 21% O2 would reduce decay (Yang et al., Citation2020). Van de Velde et al. (Citation2019) indicated that the high O2 and high CO2 combined atmosphere inhibited effectively microbial growth in blackberries. Also, atmospheres combining high CO2 and high O2 were the most effective in preventing fungal growth in strawberry fruit stored at 8°C (Pérez and Sanz, Citation2001). Similarly, our results showed that the pre-storage atmospheres include high levels of CO2 or O2 (20%/40%) in MAP5 or (80%/5%) in MAP3 had protective effects against the physical deterioration of apricot fruit after 28 days of cold storage. A recent study, have reported similar results on peach (Belge et al., Citation2019).
Shelf Life
As shown in , the shelf life significantly extended in all active MAP treatments except MAP4 compared to the control. The highest shelf-life (29.6 days after cold storage) was obtained in MAP5 treatment. The shelf life or fresh fruit consumption survival is a very important trait from the postharvest viewpoint (Thompson et al., Citation2018). Fruit shelf life is affected by many factors such as temperature, humidity, mechanical pressures, enzymes, and ethylene. Ethylene plays a vital role in fruit quality, and it is recognized that the process of ripening in climacteric fruits is related to ethylene; one of the compounds that can inhibit the action of ethylene is carbon dioxide gas (Thompson et al., Citation2018). Other authors found that application of high O2 (80 or 90%) and CO2 (10 or 20%) concentrations in MAP extended the shelf life of strawberries, maintained tissue hardness and reduced decay caused by Botrytis fungi (Ballard and Junior, Citation2019). Consistent with our results, the effect of modified atmosphere on the quality and shelf life of apricot cv. ‘Palmer’ was studied, the results showed that high CO2 (20%) significantly affected tissue hardness and pectin content and extended the shelf life through a decrease in respiration rate by high CO2 (20%) (de Almeida Teixeira et al., Citation2018).
Sensory Evaluation
All treatments except MAP1 significantly (P ≤ .01) increased the sensorial assessments compared to control (). After 28 days of cold storage samples of MAP2 and MAP5 treatments, were acceptable in terms of taste and appearance. The control fruit had bad taste and appearance as scored lower by the panelists (). Other treatments also did not receive acceptable scores.
Figure 3. The appearance of apricot fruit cv. ‘Shahroudi.’ Fruit were exposed to different MAP treatments for 6 days at 2°C prior to 4 weeks cold storage at 2°C.
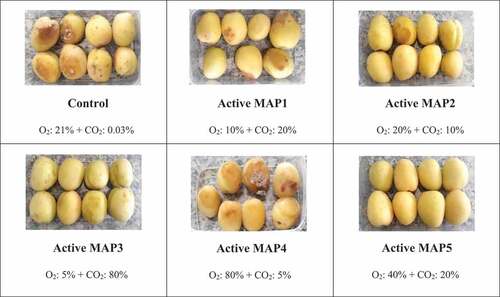
Taste is a sensory effect of products that are often determined by the flavor and smell. Taste is one of the important characteristics of products that indicates maturity. On the other hand, if the ripening process is too slow during cold storage, the apricot fruits will not sweeten. The use of high carbon dioxide reduces fruit respiration, but high levels of carbon dioxide cause anaerobic respiration and produce bad taste in fruits (Pérez and Sanz, Citation2001). Our results also showed that samples of MAP3 (CO2, 80%) treatment scored high taste, which is in coincident with the findings of Yarılgaç et al. (Citation2019) on cornelian cherry fruit.
The interesting point of this experiment is that CO2 levels were similar (20%) in MAP1 and MAP5 treatments, whereas MAP5 had the highest nutritional quality, but that did not occur on MAP1. Our results clearly showed that high O2 (40%) content in MAP5 could reduce the adverse effects of high CO2 and increase its positive impact on the fruit texture and taste.
Fruit appearance is major criteria of quality from consumers’ point of view because consumers judge the product using color, decay, defect, shape, and size assessments. As mentioned earlier, high CO2 can decay and destroy fruit tissue by producing harmful substances such as acetaldehyde, which, lead also to change the appearance. High O2 also, by activating the cell wall hydrolyzing enzymes results in promoting fruit ripening process, and appears to be watery and very soft (, MAP4). The results showed that when using high CO2 with high O2, its adverse effects of high CO2 are reduced, and the appearance of the fruit is acceptable. Other researchers have found similar results on papaya fruit (Fahmy et al., Citation2019), and on mango fruit (Ntsoane et al., Citation2020).
Conclusions
In the present study, we concluded that in apricots packaged in MAP5 (40% O2 + 20% CO2), the shelf life extended and doubled, and the qualitative attributes preserved during 28 days of storage at 2°C. Besides active MAP5 also has benefits from a nutritional viewpoint as vitamin C content and carotenoid were the highest in MAP5 fruits. In addition, sensory evaluation of pre-storage packaging of apricot fruit in an atmosphere content high O2 and high CO2 reduced the harmful effects of using either high O2 or high CO2 and had better taste and appearance. So, it can be recommended to prolong postharvest life of fresh apricot fruit cv. Shahroudi. This method provides a relatively low-cost alternative to controlled atmosphere storage.
Disclosure Statement
No potential conflict of interest was reported by the author(s).
References
- Agar, I. T., J. Streif, and F. Bangerth. 1997. Effect of high CO2 and controlled atmosphere (CA) on the ascorbic and dehydroascorbic acid content of some berry fruits. Postharvest Biol. Technol. 11(1):47–55. doi: 10.1016/S0925-5214(97)01414-2.
- Allende, A., A. Marín, B. Buendía, F. Tomás-Barberán, and M.I. Gil. 2007. Impact of combined postharvest treatments (UV-C light, gaseous O3, superatmospheric O2 and high CO2) on health promoting compounds and shelf-life of strawberries. Postharvest Biol. Technol. 46(3):201–211. doi: 10.1016/j.postharvbio.2007.05.007.
- Álvarez-Hernández, M. H., G. B. Martínez-Hernández, F. Avalos-Belmontes, F.D. Miranda-Molina, and F. Artés-Hernández. 2020. Postharvest quality retention of apricots by using a novel sepiolite–loaded potassium permanganate ethylene scavenger. Postharvest Biol. Technol. 160:111061. doi: 10.1016/j.postharvbio.2019.111061.
- Arnon, A.N. 1967. Method of extraction of chlorophyll in the plants. Agron J. 23(1):112–121.
- Assis, J.S., R. Maldonado, T. Muñoz, M.I. Escribano, and C. Merodio. 2001. Effect of high carbon dioxide concentration on PAL activity and phenolic contents in ripening cherimoya fruit. Postharvest Biol. Technol. 23(1):33–39. doi: 10.1016/S0925-5214(01)00100-4.
- Ayhan, Z., O. Eştürk, and F. Müftüoğlu. 2009. Effects of coating, modified atmosphere (MA) and plastic film on the physical and sensory properties of apricot. In X International Controlled and Modified Atmosphere Research Conference 876:143–150. doi: 10.17660/ActaHortic.2010.876.17
- Ballard, C.R., and M.R.M. Junior. 2019. Health benefits of flavonoids, p. 185–201. In: M. Campos (ed.). Bioactive compounds. Woodhead Publishing, Campinas, Brazil. doi: 10.1016/B978-0-12-814774-0.00010-4.
- Bang, J., S. Lim, G. Yi, J.G. Lee, and E.J. Lee. 2019. Integrated transcriptomic-metabolomic analysis reveals cellular responses of harvested strawberry fruit subjected to short-term exposure to high levels of carbon dioxide. Postharvest Biol. Technol. 148:120–131. doi: 10.1016/j.postharvbio.2018.11.003.
- Belge, B., L.F. Goulao, E. Comabella, J. Graell, and I. Lara. 2019. Postharvest heat and CO2 shocks induce changes in cuticle composition and cuticle-related gene expression in ‘October Sun’peach fruit. Postharvest Biol. Technol. 148:200–207. doi: 10.1016/j.postharvbio.2018.11.005.
- Chuah, A.M., Y.C. Lee, Y. Amaguchi, H. Takamura, L.J. Yin, and T. Matoba. 2008. Effect of cooking on the antioxidant properties of coloured peppers. Food Chem. 111(1):20–28. doi: 10.1016/j.foodchem.2008.03.022.
- Davarynejad, G., S. Khorshidi, J. Nyéki, Z. Szabó, and J. Gal-Remennyik. 2010. Antioxidant capacity, chemical composition and physical properties of some apricot (Prunus armeniaca L.) cultivars. Hortic Environ Biotechnol 51(6):477–482.
- de Almeida Teixeira, G.H., L.O. Santos, L.C.C. Júnior, and J.F. Durigan. 2018. Effect of carbon dioxide (CO2) and oxygen (O2) levels on quality of ‘Palmer’mangoes under controlled atmosphere storage. J Food Sci Technol 55(1):145–156. doi: 10.1007/s13197-017-2873-4.
- Fahmy, K., F. Violalita, O.C. Chatib, R. Yulia, and K. Nakano. 2019. The individual influences of high CO2 on chilling injury suppression of ‘Merah Delima’ papaya fruit. IOP Conf. Ser. 230(1):1–9. doi: 10.1088/1755-1315/230/1/012016.
- Fan, S., J. Li, Y. Xia, X. Tian, Z. Guo, and W. Huang. 2019. Long-term evaluation of soluble solids content of apples with biological variability by using near-infrared spectroscopy and calibration transfer method. Postharvest Biol. Technol. 151:79–87. doi: 10.1016/j.postharvbio.2019.02.001.
- González, J., A. Ferrer, R. Oria, and M.L. Salvador. 2008. Determination of O2 and CO2 transmission rates through microperforated films for modified atmosphere packaging of fresh fruits and vegetables. J Food Eng 86(2):194–201. doi: 10.1016/j.jfoodeng.2007.09.023.
- Kader, A.A., and M.E. Saltveit. 2003. Atmosphere modification, p. 229–246. In: J.A. Bartz and J.K. Brecht (eds.). Postharvest physiology and pathology of vegetables. CRC Press, USA.
- Kader, A.A., and C.B. Watkins. 2000. Modified atmosphere packaging toward 2000 and beyond. J. Hort. Technol. 10(3):483–486. doi: 10.21273/HORTTECH.10.3.483.
- Kim, Y., J.K. Brecht, and S.T. Talcott. 2007. Antioxidant phytochemical and fruit quality changes in Mango (Mangifera indica L.) following hot water immersion and controlled atmosphere storage. Food Chem. 105(4):1327–1334. doi: 10.1016/j.foodchem.2007.03.050.
- Mandić, A.I., A.J. Meléndez-Martínez, F. Bantis, V. Böhm, G.I.A. Borge, M. Brnčić, and N. O’Brien. 2021. A comprehensive review on carotenoids in foods and feeds: Status quo, applications, patents, and research needs Crit. Rev. Food Sci. Nutr. 1–51 . doi: 10.1080/10408398.2020.1867959.
- Manganaris, G.A., and C.H. Crisosto. 2020. Stone fruits: Peaches, nectarines, plums, apricots, p. 311–322. In: M.I. Gil and R.M. Beaudry (eds.). Controlled and modified atmospheres for fresh and fresh-cut produce. Academic Press, United Kingdom. doi: 10.1016/B978-0-12-804599-2.00017-X.
- Manganaris, G.A., M. Vasilakakis, G. Diamantidis, and I. Mignani. 2007. The effect of postharvest calcium application on tissue calcium concentration, quality attributes incidence of flesh browning and cell wall physicochemical aspects of peach fruits. Food Chem. 100(4):1385–1392. doi: 10.1016/j.foodchem.2005.11.036.
- Mapelli-Brahm, P., F.J. Barba, F. Remize, C. Garcia, A. Fessard, A.M. Khaneghah, and A.J. Meléndez-Martínez. 2020. The impact of fermentation processes on the production, retention and bioavailability of carotenoids: An overview. Trends Food Sci. Technol. 99:389–401. doi: 10.1016/j.tifs.2020.03.013.
- Mitcham, E.J., and R.B. Elkins. 2007. Pear production and handling manual. University Division of Agriculture and Natural Resources (UCANR) Publications, California.
- Moradinezhad, F., E. Ansarifar, and M. Mohammadian Moghaddam. 2020. Extending the shelf life and maintaining quality of minimally-processed pomegranate arils using ascorbic acid coating and modified atmosphere packaging. J. Food Meas. Charact. 14(6):3445–3454. doi: 10.1007/s11694-020-00591-1.
- Moradinezhad, F., and M. Dorostkar. 2020. Effectiveness of pre-storage oxygen, carbon dioxide and nitrogen‐enriched atmospheres on shelf-life, quality and bioactive compounds of fresh apricot fruit. South West. J. Hortic. Biolo. Environ. 11(2):111–130.
- Moradinezhad, F., and M. Dorostkar. 2021. Effect of vacuum and modified atmosphere packaging on the quality attributes and sensory evaluation of fresh jujube fruit. Int. J. Fruit Sci. 21(1):82–94. doi: 10.1080/15538362.2020.1858470.
- Moradinezhad, F., and M. Jahani. 2016. Quality improvement and shelf life extension of fresh apricot fruit (Prunus Armeniaca cv. Shahroudi) using postharvest chemical treatments and packaging during cold storage. Int. J. Hortic. Sci. Technol. 3(1):9–18. doi: 10.22059/ijhst.2016.58156.
- Moradinezhad, F., and M. Jahani. 2019. Effect of potassium permanganate, 1-Methylcyclopropene and modified atmosphere packaging on postharvest losses and quality of fresh apricot cv. Shahroudi. J. Hortic. Postharvest Res. 2:39–48. doi: 10.22077/JHPR.2018.1207.1007.
- Moradinezhad, F., M. Khayyat, F. Ranjbari, and Z. Maraki. 2018. Physiological and quality responses of Shishe-Kab pomegranates to short-term high CO2 treatment and modified atmosphere packaging. Int. J. Fruit Sci. 18(3):287–299. doi: 10.1080/15538362.2017.1419399.
- Muftuoğlu, F., Z. Ayhan, and O. Esturk. 2012. Modified atmosphere packaging of Kabaaşı apricot (Prunus armeniaca L. ‘Kabaaşı’) effect of atmosphere, packaging material type and coating on the physicochemical properties and sensory quality. Food Bioprocess Technol. 5(5):1601–1611. doi: 10.1007/s11947-010-0482-6.
- Nielsen, S.S. 2010. Determination of moisture content, p. 17–27. In: S.S. Nielsen (ed.). Food analysis. Springer, New York.
- Ntsoane, M.L., D. Sivakumar, and P.V. Mahajan. 2020. Optimisation of O2 and CO2 concentrations to retain quality and prolong shelf life of ‘shelly’ Mango fruit using a simplex lattice mixture design. Biosyst. Eng. 192:14–23. doi: 10.1016/j.biosystemseng.2020.01.009.
- Pérez, A.G., and C. Sanz. 2001. Effect of high-oxygen and high-carbon-dioxide atmospheres on strawberry flavor and other quality traits. J. Agric. Food Chem. 49(5):2370–2375. doi: 10.1021/jf001438l.
- Siracusa, V. 2012. Food packaging permeability behaviour: A report. Int. J. Polym. Sci. 1–12. doi: 10.1155/2012/302029.
- Sun, T., Y. Tadmor, and L. Li. 2020. Pathways for carotenoid biosynthesis, degradation, and storage, p. 3–23. In: Rodríguez-Concepción M., Welsch R. (eds.). Plant and food carotenoids, Methods in Molecular Biology. Vol. 2083 . Humana, New York, NY. doi: 10.1007/978-1-4939-9952-1-1.
- Thompson, A.K., R.K. Prange, R. Bancroft, and T. Puttongsiri. 2018. Controlled atmosphere storage of fruit and vegetables. 3rd ed. CABI, USA.
- Van de Velde, F., M.P. Méndez-Galarraga, M.H. Grace, C. Fenoglio, M.A. Lila, and M.É. Pirovani. 2019. Changes due to high oxygen and high carbon dioxide atmospheres on the general quality and the polyphenolic profile of strawberries. Postharvest Biol. Technol. 148:49–57. doi: 10.1016/j.postharvbio.2018.10.015.
- Van de Velde, F., M.P. Méndez-Galarraga, and M.É. Pirovani. 2020. Effect of enriched O2 and CO2 atmospheres on the overall quality and the bioactive potential of fresh blackberries. Postharvest Biol. Technol. 164(1):1–9. doi: 10.1016/j.postharvbio.2020.111166.
- Wang, J., P. Li, B. Gong, S. Li, and H. Ma. 2017. Phenol metabolism and preservation of fresh in‐hull walnut stored in modified atmosphere packaging. J. Sci. Food Agric. 97(15):5335–5342. doi: 10.1002/jsfa.8422.
- Yahia, E.M. Ed. 2009. Modified and controlled atmospheres for the storage, transportation, and packaging of horticultural commodities. 1st ed. CRC press, Queretaro, Mexico. doi: 10.1201/9781420069587.
- Yang, M., Z. Ban, Z. Luo, J. Li, H. Lu, D. Li, and L. Li. 2020. Impact of elevated O2 and CO2 atmospheres on chemical attributes and quality of strawberry (Fragaria× ananassa Duch.) during storage. Food Chem. 307(1):1–36. doi: 10.1016/j.foodchem.2019.125550.
- Yarılgaç, T., H. Kadim, and B. Ozturk. 2019. Role of maturity stages and modified‐atmosphere packaging on the quality attributes of cornelian cherry fruits (Cornus mas L.) throughout shelf life. J. Sci. Food Agric. 99(1):421–428. doi: 10.1002/jsfa.9203.