ABSTRACT
Climate change in Maine is characterized by increasing rates of warming temperatures and more intense rain events, which lead to altered growing seasons, earlier emergence of pests, increased seasonal drought and large crop losses. These changes have significant impacts on wild blueberry systems and make it more difficult for growers to manage this crop under increasingly unpredictable weather patterns. Mulching is a tool growers use to combat some of these challenges, but the comparative effects of wood mulch particle size in wild blueberry systems has not been studied. The purpose of this study was to determine the effect of wood mulch particle size on wild blueberry soil, plant development, pest pressure, and yield. This study was carried out over a 2-year period (one production cycle) in an organic wild blueberry field in Stockton Springs, ME. There was no mulch particle size treatment used in this study that demonstrated significantly greater overall mean soil moisture compared to the control at the 1.27 cm application thickness. All mulch treatments significantly reduced disease pressure, primarily Sphaerulina leaf spot caused by Sphaerulina vaccinii, compared to the control in year 1. The two finest particle size treatments, sawdust and shavings, resulted in significantly greater yields than the control, and were the least expensive materials. This suggests that growers should consider using finer particle size mulches rather than the current practice, wood chips, but may need to reapply more frequently. From this study, application of softwood mulch of any particle size is better than no application.
Introduction
Wild Blueberry Production
Wild blueberries (Vaccinium angustifolium Aiton.) are a culturally and economically significant perennial fruit crop in the northeastern United States and Canada. Maine and eastern Canada are the world’s leading wild blueberry growing regions where nearly 22,000 and 82,000 metric tons, respectively, were produced in 2020 (Brazelton et al., Citation2021). Growers manage commercial wild blueberry fields on a 2-year production cycle. In year 1 (prune year), fields are mowed or burned to stimulate new vegetative growth, resulting in flower buds. In year 2 (crop year), plants bloom and berries develop for harvest in August. The 2-year cropping cycle is used as a pest management tool to reduce weed, insect pest, and plant pathogen populations in addition to promoting new shoot and bud development for increased yield and quality (Drummond et al., Citation2009).
Climate Change Impacts on Wild Blueberry
The Northeast is warming faster than any other region in the U.S. (Fernandez et al., Citation2020). The average annual temperature in Maine, as of 2020, has increased by 1.78°C since 1895 with the greatest temperature increases occurring along the coast, where most wild blueberries are grown in the state (Fernandez et al., Citation2020; Tasnim et al., Citation2021). Previous research suggests hotter temperatures may cause earlier emergence of insect pests, such as spotted wing Drosophila, Drosophila suzukii (Matsumura) (Diptera: Drosophilidae) (Drummond et al. Citation2019). Warming temperatures also lead to worsening periods of drought as they favor drying and amplify soil moisture deficits (Gu et al., Citation2019; Naumann et al., Citation2018; Samaniego et al., Citation2018). Sufficient soil moisture is necessary for optimal morphological and physiological growth, including bud development, fruit ripening, and yield for many small fruit crops, including wild blueberries (Benoit et al., Citation1984; Davies and Albrigo, Citation1983; Glass et al., Citation2003; Hicklenton et al., Citation2000). Wild blueberries are grown in sandy, well-draining soils that have a low water-holding capacity and, therefore, may experience large crop losses if they are unable to maintain adequate soil moisture. Drought conditions in 2020 resulted in 43.7% mean crop loss in Maine (Schattman et al., Citation2021).
Average annual precipitation in Maine has increased in the intensity of rain events (Birkel and Mayewski, Citation2018) and total amount (14.73 cm since 1895) (Fernandez et al., Citation2020). This increased precipitation is associated with September and October tropical cyclones, as well as warming temperatures in the Atlantic Ocean (Frei et al., Citation2015; Huang et al., Citation2018, Citation2017). Therefore, the timing of these events does not always occur at ideal points during the wild blueberry growing season when plants require the most water.
This crop requires 2.54 cm of water each week from April through October to meet plant demand (Hunt et al., Citation2008). Historical rainfall from 1959 to 1998 across the Maine wild blueberry growing region shows the probability of reaching this rainfall requirement each week is less than 50% throughout the growing season, and less than 20% during the critical fruiting period, mid-July through August (Dalton and Yarborough, Citation2004). According to these long-term weather trends, wild blueberries will lack sufficient water in August in 4 of every 5 years (Hunt et al., Citation2009).
Mulching Wild Blueberry
Mulch can be any material spread on top of the soil, rather than incorporated into it (Chalker-Scott, Citation2007). Mulch can be used for several purposes, including reduction of water loss, moderation of soil temperature, suppression of weed growth, prevention of disease spread, and stimulation of rhizome growth (Drummond et al., Citation2009; Yarborough, Citation2012). Organic mulches are best-suited for achieving overall plant performance compared to inorganic or synthetic materials, such as gravel or plastic mulches (Chalker-Scott, Citation2007). Mulching with organic matter covers the soil surface, preventing it from drying out, sealing shut, and losing porosity (Bot and Benites, Citation2005), promoting a more drought-resistant soil.
Mulching and mowing are the primary methods that wild blueberry farmers use to build organic matter by increasing the thickness of the “organic pad,” thereby improving soil health. This O horizon, or “organic pad,” serves as a substrate with high water-holding capacity due to high levels of organic matter between 11% and 12% (Argall et al., Citation1998; Eaton et al., Citation2009). Organic growers use prescribed burning to double as a pruning and pest management tactic. However, burning for long periods of time or at very hot temperatures can reduce the amount of valuable organic matter on the soil surface, which may result in leaching of added nutrients from the blueberry root zone. Increasing soil organic matter can increase water-holding capacity and retention of soil moisture, allowing more nutrients to dissolve in the soil solution. This makes essential nutrients more readily available for plant uptake, especially when the acidic pH of wild blueberry soils (4.0–5.0) limits the availability of macronutrients, such as nitrogen and phosphorous (Jones and Jacobsen, Citation2005).
On Maine wild blueberry farms, wood mulch, usually in the form of wood chips, has traditionally been applied in thick patches between five and ten centimeters deep to cover bare spots, encourage rhizome expansion, and prevent weed growth (DeGomez and Smagula, Citation1990b; NRCS (Natural Resources Conservation Service), Citation2017). Mulching increases growth and survival of blueberry seedlings (Smagula and Goltz, Citation1988) and improves soil water retention during wet and dry periods (Hunt et al., Citation2010). Sanderson and Cutcliffe (Citation1991) found that a sawdust mulch applied at a thickness of 5 cm increased total wild blueberry yield by nearly 30% compared to 0 cm and 10 cm treatments. The abundance of soft wood materials in Maine coupled with ease of spreading material by manure spreaders across fields makes mulching a viable cultural management option for farmers interested in increasing soil water availability by reducing water loss and increasing organic matter rather than increasing water inputs. However, research on the comparative effects of mulch particle size has not, to our knowledge, been investigated in the wild blueberry cropping system.
Due to the lack of research on mulch particle size effects in wild blueberry, this study evaluated the effects of varying particle sizes of organic pine mulch on wild blueberry growth and soil characteristics. Specifically, our objectives were to determine the effects of mulch particle size on (i) the wild blueberry soil environment (soil moisture, temperature, nutrients, and organic matter), (ii) wild blueberry plant growth (vegetative and reproductive growth, i.e., foliar nutrients, stem height, bud/flower/fruit development), and (iii) pest pressure (density and cover of weeds, insects, and disease). We hypothesized that mulch treatments would increase soil moisture, soil organic matter, plant growth, and yield as well as reduce pest pressure compared to the control treatment. Specifically, we hypothesized that finer particle sizes would be more effective in increasing soil moisture by contributing a greater fraction to soil organic matter as they decomposed compared to larger particle sizes.
Materials and Methods
Study Site
The study took place in a MOFGA (Maine Organic Farmers and Gardeners Association) certified organic field in Stockton Springs, ME, USA. The field was in the prune year in 2020 and crop year in 2021. The experiment began with mulch application in May 2020 and continued through September 2021 (one production cycle). The experimental design was a randomized complete block design with six replicates. Each plot was 1.83 m by 9.14 m (16.72 m2) separated by 0.91 m buffers.
Treatments
Wild blueberry plant growth and soil characteristics were measured under five treatments including four different mulch particle sizes and a control (no mulch). Mulches were all from the same material, untreated white pine (Pinus strobus L.), purchased as by-products from N.C. Hunt Lumber, a sawmill and manufacturing company in Jefferson, Maine. All products were applied once during the prune cycle on May 19 and 20, 2020 at a thickness of 1.27 cm.
Particle size was determined using 2.0-, 4.0-, 6.3-, 9.5-, and 12.5-mm soil sieves. Samples of each mulch type were collected in one-liter resealable bags on May 20, 2020, and the total amount of material collected was used in the particle size analysis. The fraction of material able to pass through each sieve was weighed to calculate the percent of material within each sieve size range (). The median particle size (D50), the sieve size that allows 50% of particles to pass through, was used to rank mulch treatments by the most abundant particle size in each (Corey and Kemper, Citation1968; Diaz et al., Citation2005). Mulch treatments are ranked by increasing size as follows: sawdust (smallest, D50 < 2 mm), wood shavings (medium, 2.0 mm < D50 < 4.0 mm), bark mulch (large, 4.0 mm < D50 < 6.3 mm), and wood chips (extra-large, 6.3 mm < D50 < 9.5 mm).
Table 1. Particle size distribution for each mulch type by percent of dry matter within each sieve size range. Mulches were applied to an organic wild blueberry field during the prune year in Stockton Springs, ME on May 20, 2020.
A separate sample of each mulch type was collected on May 20, 2020 and submitted to the Maine Agricultural and Forest Experiment Station Analytical Laboratory for analysis of pH, nitrogen, calcium, potassium, magnesium, phosphorus, aluminum, boron, copper, iron, manganese, and zinc. A separate sample of each mulch type was weighed before and after drying in a gravity convection oven (Thermo Scientific PR305225G, Columbia, MD, USA) for 48 h to determine the percent moisture of each material ().
Table 2. Mulch treatment characteristics (pH, chemical composition, mean moisture content, dry matter application rate, and cost) for material applied to organic wild blueberry in Stockton Springs, ME. Mulch samples were collected on May 20, 2020, the same day as application. Costs represent those incurred during the current study but do not include labor costs. Prices are based on one application of each material applied at a 1.27 cm thickness.
Data Collection
Soil Environment
Soil samples were taken using a hand-held soil probe to a depth of 10 cm before treatments were applied on May 19, 2020. Each plot was soil sampled again after harvest on July 30, 2021, the final year of the project. Any visible mulch material that mixed with the soil during sampling was removed from soil samples collected after harvest prior to analysis. All samples were analyzed using standard field soil tests by the Maine Agricultural and Forest Experiment Station Analytical Laboratory (Hoskins, Citation1997).
Soil temperature (°C) and moisture (% volumetric water content [VWC]) were monitored weekly using TDR-150 (time domain reflectometry) probes (FieldScout TDR 150 Soil Moisture Meter, Spectrum Technologies, Inc., Aurora, IL, USA) by inserting them 12 cm (4.8 in.) into the soil of the blueberry root zone. Eight random readings were recorded per plot between 10:00 am and 2:00 pm on each collection date to maintain relatively consistent mid-day sampling conditions. Collection dates were performed weekly and, when possible, twice weekly before and after rain events. Collection dates for 2020 were June 2, 4, 8, 16; July 1, 10, 15, 24, 31; and August 10, 20, 31. Collection dates for 2021 were June 1, 10, 18, 24; and July 1, 6, 16, 22, 28.
Plant Growth
Prune Year Measures: Stem density was measured on June 19 and August 31, 2020 by counting the number of stems in each of two 0.37-m2 quadrats randomly placed in each plot at the beginning of the season and flagged for repeated use.
Crop Year Measures: Stem height and bud number per stem were recorded for each of the five stems within each quadrat on April 23, 2021. These five stems were marked with metal tags and used to measure fruit development. The number of open flowers per stem was recorded during peak bloom on May 21, 2021. The number of green, red, and blue fruit per stem was recorded three times, once prior to full ripening on June 18, 2021, once during ripening on July 6, 2021, and once during harvest on July 28, 2021.
Berries were harvested by hand-raking plots on July 28, 2021. Quadrat yield was raked and weighed separately before being added to the whole plot yield. Measures of berry quality included 100-berry weights as an indication of berry size and °Brix as an indication of sugar content. For 100-berry weights, a representative sample of 100 berries were randomly counted and weighed. This sample was then blended using a Ninja Express Chop blender (SharkNinja Operating LLC, Newton, MA, U.S.A.). A subsample was deposited on a handheld PAL-BRIX/ACID F5 refractometer (Atago, Saitama, Japan) for the Brix measurements taken on July 30, 2021.
Both Year Measures: Blueberry cover was visually estimated per quadrat using a 0–6 scale ranking system with even intervals that sum to 100%, where: 0 = not present, 1 = ≤1%–16.67%, 2 = >16.67%–33.33%, 3 = >33.33%–50%, 4 = >50%–66.67%, 5 = >66.67%–83.33% and 6 = >83.33%–100%. Blueberry cover was recorded in 2020 on June 19, July 15, and August 20 and in 2021 on June 18 and July 13.
Pest pressure was measured monthly using the same existing quadrats and ranking system used for estimating blueberry cover. Each quadrat was evaluated for cover and density of weeds, insects, and disease. Weed plants were recorded as broadleaf or grasses. Broadleaf plants were identified as species, while all grass species were grouped together. Disease and insects were identified as species. Only visibly infected or infested stems indicated by physical damage to plant tissue (leaf spots, mummy berries, chewing, galling, or webbing) characteristic of a single species were counted for disease and insect measures. Therefore, observations of live insects or possible larvae residing within plant structures were not included in the current study. Pest pressure counts were extrapolated from quadrat measurements to present the number of weed plants, diseased stems, or stems with visible insect damage per m2. Pest pressure measurements were recorded on the same dates as blueberry cover.
Twenty stems from each plot were randomly selected and marked with flagging tape to record leaf chlorophyll content in SPAD units monthly. This was measured as an indicator of leaf nitrogen (Xiong et al., Citation2015) using a SPAD Chlorophyll Meter (SPAD 502; Minolta Corp, Osaka, Japan). One measurement was taken on each of the two leaves per stem, one on the lowermost portion and one on the uppermost portion. These measurements were recorded on June 19, July 15, and August 20 in 2020 and on June 18 and July 16 in 2021.
End of Data Collection
Wild blueberry plant development during 2021 was at least 2 weeks ahead of what was observed in 2020, which was also several weeks ahead in development compared to past years. Therefore, the harvest took place much earlier in the season. Any measurements planned for August 2021 were terminated after harvest.
Data Analysis
The effects of mulch treatments on soil (moisture, temperature, nutrients), pest pressure (insects, disease, weeds), plant growth (stem height, bud/flower/berry development, stem density, blueberry cover, chlorophyll content), yield, and berry quality were statistically analyzed using JMP software (JMP® Pro, Version 15.2.0) (SAS Institute, Citation2020b). One-way analysis of variance was used to analyze datasets for only one date, including stem density, stem height, bud number per stem, flowers per stem, berries per stem, and soil nutrients. Randomization testing was used to analyze data that did not follow a normal distribution and confirm results of analyses of variance that did not meet standard parametric assumptions.
All repeated measures were analyzed using a mixed model. Data that met assumptions of normality and equal variances were analyzed using a linear mixed model of standard least squares. Data that could not meet assumptions of normality and equal variances were analyzed using a generalized linear mixed model (GLMM) after concluding that there were no other major problems with the data set (SAS Institute, Citation2020a). In the GLMM, count data were modeled with a Poisson distribution and log link. Proportion data (ranks) were modeled with a binomial distribution and logit link. To model rank data, ranks were first converted to the percent midpoint of the range that each rank represents. In each model, “block” was used as a random effect and all other variables were considered fixed effects. All fixed-by-fixed effect interactions were included in each model for statistical analysis. Treatment effects were separated by Tukey’s Highly Significant Differences test at the 0.05 significance level.
Results
The Maine statewide 2020 and 2021 summer (June–August) temperature and rainfall averages were warmer and drier than normal (Birkel, Citation2020a, Citation2021). The average summer temperature in 2020 was 18.72°C, the third warmest since 1895. The average summer temperature in 2021 was 18.67°C, the fourth warmest since 1895, following 2020. Total precipitation for the 2020 summer period was 22.35 cm, the 14th driest since 1895. Total precipitation for 2021 was 26.00 cm, 2.03 cm less than the historical mean.
Soil Environment
In year 1, mean soil percent organic matter (% OM) was 9.88% prior to treatment application. Chemical analysis showed variability across treatments for pH and chemical composition (). Soil nutrient test results taken in year 2 indicated that there was a significant treatment effect on soil buffer pH (F = 3.79; df = 4, 29; P = .0153), % OM (F = 7.19; df = 4, 29; P = .0005), soil calcium (F = 3.91; df = 4, 29; P = .0134), soil manganese (F = 2.48; df = 4, 29; P = .0694), and cation exchange capacity (CEC) (F = 6.13; df = 4, 29; P = .0014) (). Buffer pH was significantly greater in bark mulch and shaving treatments compared to the control treatment. The sawdust treatment had significantly greater mean % OM than shaving, bark, and wood chip treatments and significantly greater soil calcium, soil manganese, and CEC compared to the wood chip treatment. The control treatment also had significantly greater % OM and CEC compared to the wood chip treatment.
Table 3. Soil analysis treatment means from soil sampling wild blueberry plots on July 30, 2021 after harvest in Stockton Springs, ME. Results include soil pH and buffer pH, percent organic matter (% OM), all major nutrients (nitrate [NO3], ammonium [NH4], phosphorus [P], potassium [K], and calcium [Ca]), micronutrients with statistical significance (manganese [Mn]), and cation exchange capacity (CEC). Rows not connected by the same letter are statistically different at the 0.05 level of significance based on Tukey’s HSD test.
There was a significant effect of treatment (P < .0001) and date (P < .0001) on soil moisture during both years of the study (). There was also a significant interaction of treatment by date in 2020 (P < .0001). No treatment had significantly greater mean soil moisture compared to the control, except for bark mulch on June 16, 2020 (bark mean = 25.75% VWC, control mean = 22.78% VWC, P = .0138). Mean soil moisture for the shaving treatment was significantly greater than the wood chip treatment during 50% of the 2020 sampling dates. Overall mean soil moisture was significantly greater in the shaving and control treatments compared to the wood chip treatment during both years. Soil moisture ranged from 2.8% to 46.6% in 2020 and from 10.4% to 45.3% in 2021. No significant treatment effects on soil temperature were detected throughout the 2020 and 2021 field seasons. Only date had a significant effect on soil temperature (P < .0001) during both years of the study. Soil temperature ranged from 22.2°C to 37.6°C in 2020 and from 16.4°C to 43.7°C in 2021.
Figure 1. Mean soil moisture content by date and mulch type for wild blueberry prune (2020) and crop (2021) years in Stockton Springs, ME. The blue line represents total rainfall and melted snow amounts. Error bars represent the SEM (n = 240). Points not connected by the same letter are statistically different at the 0.05 level of significance based on Tukey’s HSD test. Letters representing statistical significance are ordered from top down to correspond to the same order of treatments listed in the legend from top down to help distinguish among treatments. Precipitation data is from NOAA National Environmental Satellite, Data, and Information Service record of climatological observations for prospect, ME weather station.
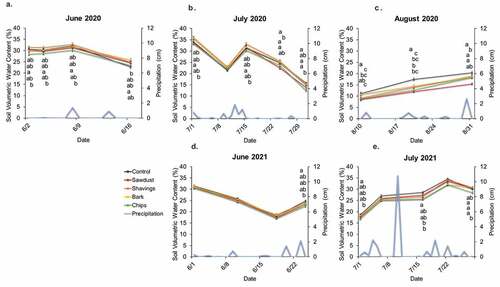
Plant Growth
Prune Year Measures
There was no significant treatment effect on stem density measured at the end of the prune year (F = 0.51; df = 4, 59; P = .7273). There was a significant treatment effect on the change in stem density from the beginning to the end of the prune year (F = 4.97; df = 4, 59; P = .0017). The change in stem density for sawdust and shaving treatments was significantly more than that of the control treatment. There was a trend toward increased stem density for all mulch treatments (sawdust by 23.93%, shavings by 34.07%, bark by 11.47%, chips by 18.74%) observed from June to August 2020. Control plots decreased in stem density by 9.47% in the same time span.
Crop Year Measures
There was a significant treatment effect on both stem height (F = 4.82; df = 4, 299; P = .0009) and number of buds per stem (F = 4.04; df = 4, 299; P = .0033). Stems were significantly taller in sawdust and shaving treatments compared to the control (). Mean number of buds per stem was also significantly greater in all mulch treatments compared to the control.
Figure 2. Mean wild blueberry stem height and number of buds per stem by mulch type. Error bars represent the SEM (n = 300). Bars not connected by the same letter are statistically different at the 0.05 level of significance based on Tukey’s HSD test.
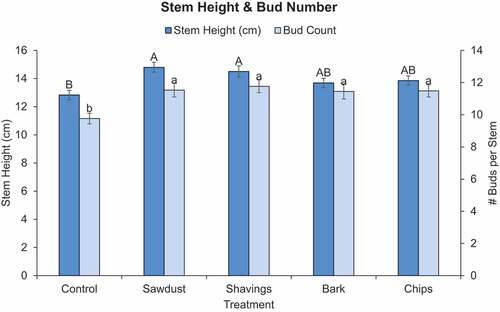
There was a significant treatment effect on the number of flowers per stem (F = 3.81; df = 4, 299; P = .0049), blue fruit per stem (F = 2.99; df = 4, 299; P = .0191), and yield (F = 3.66; df = 4, 29; P = .0176). The sawdust treatment had significantly more flowers per stem compared to the control treatment during bloom 2021 (). The bark mulch treatment had significantly more blue fruit per stem compared to the control treatment by the final date of fruit counts during harvest. Shaving and sawdust treatments had the greatest mean total yield. No significant differences were detected among treatments for the number of green fruit per stem, 100-berry weights, or sugar content as indicated by Brix measurements.
Table 4. Wild blueberry flower and fruit counts, plot yield, berry sample weights, and Brix sugar content as affected by mulch treatments during wild blueberry crop year (2021) growing season in Stockton Springs, ME. Flower, green fruit, and blue fruit counts were taken on 21 May, 18 June, and 28 July 2021, respectively. Plot yields and 100 berry weights were also taken on 28 July 2021. Brix measurements were taken 30 July 2021. Rows not connected by the same letter are statistically different at the 0.05 level of significance based on Tukey’s HSD test.
Both Year Measures
There was a significant effect of treatment, date, and the interaction of treatment by date for blueberry cover during 2020. Control plots had significantly greater percent blueberry cover than all mulch treatments in June 2020. By July and August, there were no statistically significant differences in blueberry cover among treatments. All mulch treatments increased in blueberry cover by August 2020, while blueberry cover in the control treatment decreased. This trend was also reflected in treatment changes in stem density during the prune year. There were no significant differences detected in blueberry cover in 2021.
Because insect damage did not vary in percent cover, only insect density data is presented to show the number of damaged stems/m2 (). In 2020, there was no significant treatment effect on weed density, weed cover, or insect density. There was a significant effect of date on weed density, weed cover, and insect density during 2020. These measures peaked in July, the middle of the growing season. The most abundant broadleaf weeds observed in 2020 were common cinquefoil (Potentilla simplex Michaux.), goldenrod (Solidago spp.), and golden clover (Trifolium aureum Pollich.). Grasses also made up a large fraction of weed plants observed. The most abundant insects observed were blueberry gall midge (Dasineura oxycoccana Johnson), red-striped fireworm (Aroga trialbamaculella Chambers), and flea beetle (Altica sylvia Malloch). There were no significant interactions for weeds or insects.
Table 5. Wild blueberry pest pressure as affected by mulch treatments during wild blueberry prune (2020) and crop (2021) years in Stockton Springs, ME. All measures were collected once per month in June, July, and August in 2020 and 2021. Rows not connected by the same letter are statistically different at the 0.05 level of significance based on Tukey’s HSD test. *significant at the 0.05 level of significance.
There was a significant effect of treatment, date, and the interaction of treatment by date on disease density and cover in 2020. No significant differences were detected in June 2020 (). In July and August 2020, the control treatment had significantly greater disease density and cover compared to all mulch treatments. In July, the control had 141%, 157%, 141%, and 129% more disease cover than sawdust, shaving, bark, and wood chip treatments, respectively. In August, control plots had 75%, 118%, 91%, and 91% more disease cover than sawdust, shaving, bark, and wood chip treatments, respectively. The most abundant diseases observed were leaf spot diseases, primarily Sphaerulina leaf spot caused by Sphaerulina vaccinii, which accounted for 99.7% of disease observations per stem and, therefore, accounted for significant differences among treatments.
Figure 3. Monthly mean wild blueberry disease cover as a percent representing the midpoint of the mean rank range. Data collected on June 19, July 15, and August 20 in 2020 during the prune cycle. Error bars represent the SEM (n = 60). Bars not connected by the same letter are statistically different at the 0.05 level of significance based on Tukey’s HSD test.
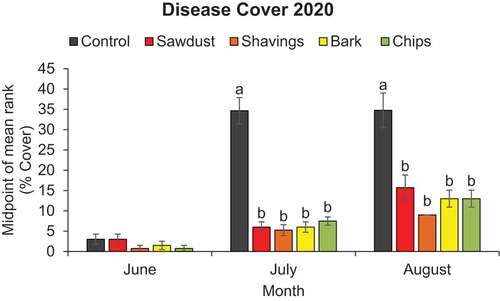
In 2021, there were no significant treatment effects on density or cover for weeds, insects, and diseases. There was a significant effect of date on weed density. The total number of weeds significantly increased from June to July 2021. The most abundant broadleaf weeds observed in 2021 were common cinquefoil (Potentilla simplex Michaux.), goldenrod (Solidago spp.), and common vetch (Vicia sativa L.). Grasses again made up a large fraction of weed plants observed. The most abundant insects observed were blueberry gall midge (Dasineura oxycoccana Johnson) and blueberry thrips (Frankliniella vaccinii Morgan and Catinathrips kainos O’Neill). There was a significant effect of date on disease density and cover in 2021, which significantly increased from June to July 2021 across all plots. The most abundant diseases observed were again leaf spot diseases, which accounted for 97.6% of disease observations per stem.
In 2020, there was a significant effect of treatment (P < .0001), date (P < .0001), and the interaction of treatment by date (P = .0004) on foliar chlorophyll content. Overall mean chlorophyll content in 2020 was significantly greater in sawdust (31.48 SPAD units), shaving (30.71 SPAD units), and wood chip (30.74 SPAD units) treatments compared to the control (29.48 SPAD units). No significant differences were detected in June 2020. Control plots were significantly lower in chlorophyll content than sawdust, shaving, and wood chip treatments during July 2020 and significantly lower than the wood chip treatment during August 2020 (). In 2021, there was a significant effect of treatment (P < .0001) and date (P < .0001) on foliar chlorophyll content. Sawdust treatments had significantly greater overall mean chlorophyll content (26.19 SPAD units) compared to all other treatments in 2021.
Figure 4. Monthly mean wild blueberry leaf tissue chlorophyll content in spad units by mulch type. Error bars represent the SEM (n = 1,200). Points not connected by the same letter are statistically different at the 0.05 level of significance based on Tukey’s HSD test. Letters representing statistical significance are ordered from top down to correspond to the same order of treatments listed in the legend from left to right to help distinguish among treatments.
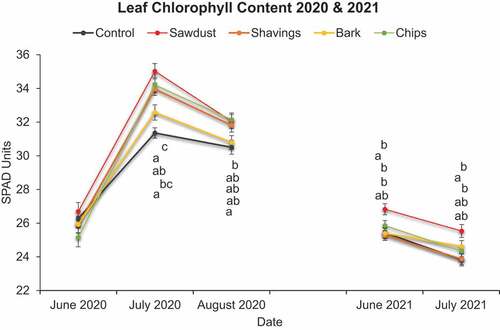
Discussion
Overall, mulching was beneficial to wild blueberry plant growth in both prune and crop years. All mulch treatments resulted in significantly fewer diseased stems with less disease cover in the first year and significantly more buds per stem by the second year compared to the control treatment. Sawdust and shaving treatments had significantly taller stems, a significant increase in stem density, and significantly greater yields compared to the control treatment. However, no mulch treatment demonstrated significantly greater overall mean soil moisture compared to the control, suggesting that the 1.27 cm mulch layer used in this study was not thick enough to conserve significantly more soil moisture than the control treatment. This thickness of mulch was chosen as an economically and logistically feasible amount of material for growers to apply to entire fields, yet further research should investigate different thicknesses of these mulches. Smaller particles sizes promoted overall blueberry plant growth and can be a useful tool for growers to increase yields more effectively than larger particle sizes such as wood chips.
Soil Environment
Our soil analysis showed soil organic matter, calcium, manganese, and CEC were significantly greater for the sawdust treatment, the finest particle size, than the wood chip treatment, the largest particle size. These differences may have been impacted by sampling error if mulch particles, along with blueberry roots and leaves, were not completely removed from soil cores during sampling. Smaller particles of sawdust were especially difficult to remove from samples compared to larger wood chips if they were mixed into soil cores during sampling. Decomposition rate may have also impacted these results. Duryea et al. (Citation1999) reported that wood mulches such as pine bark (Pinus elliottii Engelm. and P. taeda L.) showed 3% to 7% decomposition after 1 year. However, Allison (Citation1965) showed that decomposition rates were faster for finer shortleaf pine (Pinus echinata Mill.) wood particles. The sawdust in this experiment may have decomposed slightly faster than the larger particle size treatments, increasing organic matter and therefore CEC (Helling et al., Citation1964; Parfitt et al., Citation1995). Because the results of the soil analysis may be influenced by the mulches mixing with the soil during sampling and the difficulty of separating finer particles from samples, future research should follow mulch materials for more than one production cycle to record the effects of their decomposition on the soil environment.
The control treatment performed similarly or better than mulch treatments regarding soil moisture during both years (). Some studies have found that finer particle sizes of gravel mulch were more effective than larger particles in preserving soil temperature and reducing soil water evaporation (Qiu et al., Citation2014; Xie et al., Citation2010). Larger particle sizes of gravel mulch increased overall porosity of the mulch layer, lowering soil temperature and increasing evaporation. This supports our hypothesis that the finer particle size treatments in this study, sawdust and shavings, would also be more effective in increasing soil moisture compared to the larger particle sizes, regarding their porosity in addition to their contribution to the soil organic matter fraction. The susceptibility of finer particle sizes of wood mulch to wind and rain erosion may have contributed to the minimal differences observed in soil moisture among mulch treatments in this study compared to heavier gravel mulches (DeGomez and Smagula, Citation1990ab). This suggests that a heavier or thicker application than what was used in this study, 1.27 cm, may be necessary to observe greater treatment differences. Diaz et al. (Citation2005) found that reduction in soil water evaporation was proportional to tephra mulch thickness, where 10 cm of mulch resulted in the greatest reduction (92%) compared to 5 cm (83%) and 2 cm (52%). Gilman and Grabosky (Citation2004) found that pine bark mulch thickness (8–16 cm) and particle size did not significantly affect stem xylem water potential or trunk diameter in newly planted live oaks (Quercus virginiana Mill.).
Soil temperature varied by date throughout each season, as expected, but there were no significant differences among treatments. This suggests that particle size, at the thickness applied in this study (1.27 cm), did not affect soil temperature. A thicker application may have improved soil moisture retention and shown differences in soil temperature. Pakdel et al. (Citation2011) found significant reductions in soil temperature and increases in soil moisture with sawdust, wood chip, and gravel mulch at thicknesses of 5–15 cm. van Donk et al. (Citation2011) found that addition of wood chip mulch at 2.5, 5, and 10 cm thicknesses increased soil water content with the most significant differences measured at the 5 and 10 cm thicknesses. Economically, a shallower application may be required for whole-field mulching, yet a thicker application may be used to increase soil moisture in lower-yielding fields with greater water deficits. When they do apply mulch, Maine growers primarily apply pine wood chips. However, because this particle size retained the least soil moisture, growers should consider using a finer particle size when applying mulch.
Soil moisture in this study increased following precipitation events, especially those with more than 1 cm of cumulative rain, which occurred during June through August in 2020 and May through July in 2021 (). Future research should measure soil moisture at more frequent intervals to determine how long after rain events the ground stays moist under each treatment.
Plant Growth
Mulch can improve wild blueberry growth, especially rhizome spread (Kender and Eggert, Citation1966; Yarborough, Citation2012). This spread contributes to new stem growth, which was confirmed in terms of the increased stem density and blueberry cover in the current study. Although control treatments started with significantly more blueberry cover in June 2020, only mulch treatments had a positive change in stem density by the end of the prune year.
Mulch reduces diseases such as leaf spot and mummy berry by covering infected tissues, reducing inoculum, and preventing dispersal of overwintering spores (Alfieri, Citation1991; Drummond et al., Citation2009). The results of this study confirm these previous findings. All mulch treatments significantly reduced disease relative to the control in the first year. By reducing disease pressure, mulch can mitigate plant stress, likely leading to the increased stem density and number of buds per stem for all mulches, as well as increased stem height and yield for sawdust and shaving treatments compared to the control treatment. These results agree with previous research that showed leaf spot incidence in wild blueberry fields was inversely related to flower bud set and berry production (Annis and Stubbs, Citation2004; Ojiambo et al., Citation2002). A trend toward greater leaf spot disease cover in control plots during both years and fewer mean number of buds, flowers, and blue fruit per stem in year 2 was observed. Our results also confirm current understanding of the typical phenologies of common weed, insect, and disease pests in wild blueberry (Yarborough et al., Citation2009). While weed growth and emergence of adult insects peaked mid-season, disease infection significantly increased each month until the end of the growing season.
Nitrogen immobilization or “locking” has been suggested as a concern when applying mulches to crops. Sønsteby et al. (Citation2004) showed that bark mulch slightly reduced leaf tissue nitrogen and significantly reduced soil nitrate and ammonium levels in strawberry. Krewer et al. (Citation2008) suggested pine bark mulch tied up more applied nitrogen than pine straw in rabbiteye blueberry. As soil microbes use nitrogen to break down the applied organic matter, a shallow depletion zone may form at the interface of the soil surface and mulch layer. Our results showed that control plots had the lowest overall mean chlorophyll content in year 1 and were among the lowest in year 2 (). There were also no significant differences in soil NO3 or NH4 among treatments (). Therefore, nitrogen locking, which would have been indicated by lower leaf chlorophyll levels for plants in mulch treatments compared to the control, was not observed in the current study. This may be due to improved overall plant condition in mulched plots resulting from reduced disease pressure and therefore greater photosynthetic capacity and higher chlorophyll and leaf nitrogen levels. These results agree with other studies that have shown wood mulches to increase nitrogen levels in soil and foliage (Arthur and Wang, Citation1999; Cregg and Schutzki, Citation2009) rather than tie-up soil nitrogen.
Implications for Farmers
Wild blueberry farmers face many climate challenges, including erratic frost and drought events (Fernandez et al., Citation2020). Summer 2020 in Maine was the most severe short-term drought since the early 2000s and the worst fire season in the last decade (Birkel, Citation2020a). The summer of 2021 was similar in its temperature anomalies. In temperate regions, such as Maine, irrigation use may range from “not at all” to “frequent” due to uneven rainfall and unpredictable weather patterns, making it difficult to predict irrigation demand and total cost from year to year (Dalton and Yarborough, Citation2004). Given the highly variable rainfall patterns across the wild blueberry production regions of Maine, irrigation may help increase yields when rainfall is limited but poses a financial risk to farmers when rainfall events are largely sporadic. Cultural management options such as mulching can in turn be a relatively easy way to improve wild blueberry production.
Further research is needed to determine the minimal thickness of mulch needed to significantly increase soil moisture. Depending on the amount of organic matter already on the soil surface, up to 10 cm of mulch may be applied (DeGomez and Smagula, Citation1990b). If a thicker mulch application rate is used, it should be focused toward lower-yielding portions of fields. A thicker application will increase the amount of material needed and, therefore, cost for material but could improve overall productivity. The standard practice used by the Natural Resources Conservation Service is a 5.08 cm application (NRCS (Natural Resources Conservation Service), Citation2017). Growers might consider top-dressing finer particles with a larger particle size to weigh down the lightweight material, increase stability, and slow decomposition rate to reduce the need for reapplication. Applying a second material would be more expensive, however, the finest particle size treatments were the least expensive materials to purchase for this study () and significantly increased yield compared to the control (). Wood chips were the most expensive material purchased. Costs may vary depending on the source, especially if growers can produce their own mulch on-site. Future research should investigate the effectiveness of different combinations of mulch thicknesses and particle sizes on crop productivity and beneficial organisms in wild blueberry systems. Research on the comparative effects of mulching with materials that have a high decomposition rate to increase soil organic matter versus materials with a slow decomposition rate to maintain a top layer would also be valuable.
If interested in using mulch as a crop management tool, growers should consider using finer particle sizes rather than wood chips. Over time, however, larger particle size mulches may perform better than finer particle size mulches if the finer sizes are not reapplied due to their tendency to erode (DeGomez and Smagula, Citation1990b). From this study, application of softwood mulch of any particle size will improve plant health.
Acknowledgments
We would like to thank University of Maine faculty members Ellen Mallory, Yongjiang Zhang, Frank Drummond, Phil Fanning, and Bill Halteman for their assistance during the completion of this work. We thank undergraduates Sydney Abramovich, Abby Cadorette, Erica Carpenter, and Aidan Lurgio, graduate student Anthony Ayers and lab technicians Brogan Tooley and Mara Scallon for their assistance with data collection and entry. We are thankful for the wild blueberry growers that allowed us to use their fields to conduct our research. This work was supported by the Northeast Sustainable Agriculture Research and Education (SARE) program under grant number LNE19-374, the Maine Food and Agriculture Center, and the Wild Blueberry Commission of Maine.
Disclosure Statement
No potential conflict of interest was reported by the author(s).
Data Availability Statement
The data that support the findings of this study are available from the corresponding author, RG, upon reasonable request. https://doi.org/10.6084/m9.figshare.c.5756594
Additional information
Funding
References
- Alfieri, S.A. 1991. Septoria leaf spot of blueberry. Florida Department Agriculture and Consumer Services. Division of Plant Industry, Gainesville, FL, USA.
- Allison, F.E. 1965. Decomposition of wood and bark sawdusts in soil, nitrogen requirements and effects on plants. No. 1332. US Department of Agriculture, Washington D.C., USA.
- Annis, S.L., and C.S. Stubbs. 2004. Stem and leaf diseases and their effects on yield in Maine lowbush blueberry fields. Small Fruits Rev 3(1–2):159–167. doi: 10.1300/J301v03n01_16.
- Argall, J., G. Chiasson, and Y. Martineau. 1998. Soil fertility and fertilizers for wild blueberry production. Wild blueberry fact sheet D. 2.0. Department of Agriculture and Rural Development, Spring, New Brunswick.
- Arthur, M.A., and Y. Wang. 1999. Soil nutrients and microbial biomass following weed-control treatments in a Christmas tree plantation. Soil Sci. Soc. Am. J 63(3):629–637. doi: 10.2136/sssaj1999.03615995006300030028x.
- Benoit, G.R., W.J. Grant, A.A. Ismail, and D.E. Yarborough. 1984. Effect of soil moisture and fertilizer on the potential and actual yield of lowbush blueberries. Can. J. Plant Sci 64(3):683–689. doi: 10.4141/cjps84-094.
- Birkel, S. 2020. Maine climate news – Maine summer 2020 climate summary and drought update. University of Maine Cooperative Extension, Orono, ME, USA. 22 Oct. 2021. https://extension.umaine.edu/maineclimatenews/update/summer-2020/.
- Birkel, S. 2021. Statewide monthly/seasonal temperature and precipitation. University of Maine Climate Change Institute, Orono, ME, USA. 22 Oct. 2021. https://mco.umaine.edu/data_monthly/.
- Birkel, S.D., and P.A. Mayewski. (2018). Coastal Maine climate futures Climate Change Institute, University of Maine: Orono 24 4 Nov. 2021 https://climatechange.umaine.edu/wp-content/uploads/sites/439/2018/11/CoastalMaineClimateFutures-1.pdf
- Bot, A., and J. Benites. 2005. The importance of soil organic matter: key to drought-resistant soil and sustained food production. No. 80. Food and Agriculture Organization of the United Nations, Rome, Italy.
- Brazelton, C., C. Fain, M. Ogg, C. Riquelme, V. Rodriguez, and D. Mulvey. 2021. Global state of the blueberry industry report. Inter Blueberry Organ. 16 Oct. 2021. https://www.internationalblueberry.org/2021-report/.
- Chalker-Scott, L. 2007. Impact of mulches on landscape plants and the environment—a review. J. Environ. Hortic 25(4):239–249. doi: 10.24266/0738-2898-25.4.239.
- Corey, A.T., and W.D. Kemper. 1968. Conservation of soil water by gravel mulches. Hydrology Papers, No. 30, Colorado State University, Fort Collins, CO, USA.
- Cregg, B.M., and R. Schutzki. 2009. Weed control and organic mulches affect physiology and growth of landscape shrubs. Hortscience 44(5):1419–1424. doi: 10.21273/HORTSCI.44.5.1419.
- Dalton, T.J., and D.E. Yarborough. 2004. The economics of supplemental irrigation on wild blueberries. Small Fruits Rev 3(1–2):73–86. doi: 10.1300/J301v03n01_08.
- Davies, F.S., and L.G. Albrigo. 1983. Water relations of small fruits, p. 89–136. In: T.T. Kozlowski (ed.). Water deficits and plant growth, Vol. VII: Additional woody crop plants. Academic Press, New York, NY, USA.
- DeGomez, T., and J. Smagula. 1990a. Filling bare spots in blueberry fields, fact sheet no. 221. University of Maine Cooperative Extension, Orono, ME, U.S.A. 5 Nov. 2021. https://extension.umaine.edu/blueberries/factsheets/production/filling-bare-spots-in-blueberry-fields/.
- DeGomez, T., and J. Smagula. 1990b. Mulching to improve plant cover, fact sheet no. 228. University of Maine cooperative extension, Orono, ME, U.S.A. 5 Nov. 2021. https://extension.umaine.edu/blueberries/factsheets/production/mulching-to-improve-plant-cover/.
- Diaz, F., C.C. Jimenez, and M. Tejedor. 2005. Influence of the thickness and grain size of tephra mulch on soil water evaporation. Agric. Water Manag 74(1):47–55. doi: 10.1016/j.agwat.2004.10.011.
- Drummond, F., S. Annis, J.M. Smagula, and D.E. Yarborough. 2009. Organic wild blueberry production, p. 43. In: Maine agricultural and forest experiment station bulletin 852. University of Maine, Orono, ME, USA.
- Drummond F., E. Ballman,and J. Collins. 2019. Population Dynamics of Spotted Wing Drosophila (Drosophila suzukii (Matsumura)) in Maine Wild Blueberry (Vaccinium angustifolium Aiton). Insects 10(7):205. doi: 10.3390/insects10070205.
- Duryea, M.L., R.J. English, and L.A. Hermansen. 1999. A comparison of landscape mulches: Chemical, allelopathic, and decomposition properties. J. Arboric 25(2):88–97.
- Eaton, L., K. Sanderson, and S. Fillmore. 2009. Nova scotia wild blueberry soil and leaf nutrient ranges. Int. J. Fruit Sci 9(1):46–53. doi: 10.1080/15538360902801312.
- Fernandez, I., S. Birkel, C. Schmitt, J. Simonson, B. Lyon, A. Pershing, E. Stancioff, G. Jacobson, and P. Mayewski. 2020. Maine’s climate future 2020 update. University of Maine, Orono, ME, USA.
- Frei, A., K.E. Kunkel, and A. Matonse. 2015. The seasonal nature of extreme hydrological events in the northeastern United States. J. Hydrometeorol 16(5):2065–2085. doi: 10.1175/JHM-D-14-0237.1.
- Gilman, E.F., and J. Grabosky. 2004. Mulch and planting depth affect live oak (Quercus virginiana mill.) establishment. J. Arboric 30(5):311–317.
- Glass, V.M., D.C. Percival, and J.T.A. Proctor. 2003. Influence of decreasing soil moisture on stem water potential, transpiration rate and carbon exchange rate of the lowbush blueberry (Vaccinium angustifolium Ait.) in a controlled environment. J. Hortic. Sci. Biotechnol. 3:359–364. doi: 10.1080/14620316.2003.11511632.
- Gu, X., Q. Zhang, J. Li, V.P. Singh, J. Liu, P. Sun, C. He, and J. Wu. 2019. Intensification and expansion of soil moisture drying in warm season over Eurasia under global warming. J. Geophys. Res. Atmos 124(7):3765–3782. doi: 10.1029/2018JD029776.
- Helling, C.S., G. Chesters, and R.B. Corey. 1964. Contribution of organic matter and clay to soil cation‐exchange capacity as affected by the pH of the saturating solution. Soil Sci. Soc. Am. J 28(4):517–520. doi: 10.2136/sssaj1964.03615995002800040020x.
- Hicklenton, P.R., J.Y. Reekie, and R.J. Gordon. 2000. Physiological and morphological traits of lowbush blueberry (Vaccinium angustifolium Ait.) plants in relation to post-transplant conditions and water availability. Can. J. Plant Sci 80(4):861–867. doi: 10.4141/P00-003.
- Hoskins, B.R. 1997. Soil testing handbook for professionals in agriculture, horticulture, nutrient and residuals management, 3rd ed. p. 119. Maine Agriculture and Forestry Experiment Station, Orono, ME.
- Huang, H., J.M. Winter, and E.C. Osterberg. 2018. Mechanisms of abrupt extreme precipitation change over the Northeastern United States. J. Geophys. Res. Atmos 123(14):7047–7746. doi: 10.1029/2017JD028136.
- Huang, H., J.M. Winter, E.C. Osterberg, R.M. Horton, and B. Beckage. 2017. Total and extreme precipitation changes over the Northeastern United States. J. Hydrometeorol 18(6):1783–1798. doi: 10.1175/JHM-D-16-0195.1.
- Hunt, J.F., C.W. Honeycutt, G. Starr, and D. Yarborough. 2008. Evapotranspiration rates and crop coefficients for wild blueberry (Vaccinium angustifolium). Int. J. Fruit Sci 8(4):282–298. doi: 10.1080/15538360802597549.
- Hunt, J., W. Honeycutt, and D.E. Yarborough. 2009. Guide to efficient irrigation of the wild blueberry. Wild Blueberry Fact Sheet No. 631. University of Maine Cooperative Extension, Orono, ME, USA. 5 Nov. 2021. https://extension.umaine.edu/blueberries/factsheets/irrigation/guide-to-efficient-irrigation-of-the-wild-blueberry.
- Hunt, J.F., C.W. Honeycutt, and D. Yarborough. 2010. Effect of pine bark mulch on lowbush blueberry (Vaccinium angustifolium) water demand. int. J. Fruit Sci 10(4):390–415. doi: 10.1080/15538362.2010.530117.
- Jones, C., and J. Jacobsen. 2005. Plant nutrition and soil fertility, p. 11. In: Nutrient management module no. 2. Montana State University Extension Services, Bozeman, MT, USA. doi:10.1157/65603.
- Kender, W.J., and F.P. Eggert. 1966. Several soil management practices influencing the growth and rhizome development of the lowbush blueberry. Can. J.Plant Sci 46(2):141–149. doi: 10.4141/cjps66-022.
- Krewer, G., M. Tertuliano, P. Andersen, O. Liburd, G. Fonsah, H. Serri, and B. Mullinix. 2008. Effect of mulches on the establishment of organically grown blueberries in Georgia. Acta Hortic. 810(2):483–488. doi: 10.17660/ActaHortic.2009.810.63.
- Naumann, G., L. Alfieri, K. Wyser, L. Mentaschi, R.A. Betts, H. Carrao, J. Spinoni, J. Vogt, and L. Feyen. 2018. Global changes in drought conditions under different levels of warming. Geophys. Res. Lett 45(7):3285–3296. doi: 10.1002/2017GL076521.
- NRCS (Natural Resources Conservation Service). 2017. Conservation practice standard: mulching code 484. United States department of agriculture. Washington, D.C. 5 Nov. 2021. https://www.nrcs.usda.gov/wps/portal/nrcs/detailfull/national/technical/cp/ncps/?cid=nrcs143_026849#K.
- Ojiambo, P.S., H. Scherm, and P. Brannen. 2002. Septoria leaf spot intensity, defoliation, and yield loss relationships in Southern blueberry (abstr). Phytopathology 92:1025.
- Pakdel, P., A. Tehranifar, S.H. Nemati, A. Lakzian, and S.M. Kharrazi. 2011. Effect of four types of mulches including wood chips, municipal compost, sawdust and gravel in three different thicknesses on the growth of Platanus orientalis L. J. Hortic. Sci. 25(3). doi: 10.22067/jhorts4.v1390i0.11336.
- Parfitt, R.L., D.J. Giltrap, and J.S. Whitton. 1995. Contribution of organic matter and clay minerals to the cation exchange capacity of soils. Commun. Soil Sci. Plant Anal 26(9–10):1343–1355. doi: 10.1080/00103629509369376.
- Qiu, Y., Z. Xie, Y. Wang, J. Ren, and S.S. Malhi. 2014. Influence of gravel mulch stratum thickness and gravel grain size on evaporation resistance. J. Hydrol. 519:1908–1913. doi: 10.1016/j.jhydrol.2014.09.085.
- Samaniego, L., S. Thober, R. Kumar, N. Wanders, O. Rakovec, M. Pan, M. Zink, J. Sheffield, E.F. Wood, and A. Marx. 2018. Anthropogenic warming exacerbates European soil moisture droughts. Nat. Clim. Change 8(5):421–426. doi: 10.1038/s41558-018-0138-5.
- Sanderson, K.R., and J.A. Cutcliffe. 1991. Effect of sawdust mulch on yields of select clones of lowbush blueberry. can. J. Plant Sci 71(4):1263–1266. doi: 10.4141/cjps91-175.
- SAS Institute. 2020a. Fitting linear models, version 15. SAS Institute, Cary, NC.
- SAS Institute. 2020b. Using JMP, version 15. SAS Institute, Cary, NC.
- Schattman, R.E., C. Goossen, and L. Calderwood. 2021. The 2020 Maine drought and agriculture report. University of Maine, Orono. doi:10.6084/m9.figshare.14474055
- Smagula, J.M., and S.M. Goltz. 1988. Mulches affect frost heaving, survival and growth of lowbush blueberry seedlings. Hortscience 23(3):741.
- Sønsteby, A., A. Nes, and F. Måge. 2004. Effects of bark mulch and NPK fertilizer on yield, leaf nutrient status and soil mineral nitrogen during three years of strawberry production. Acta. Agric. Scand. B Soil Plant Sci 54(3):128–134. doi: 10.1080/09064710410030276.
- Tasnim, R., F. Drummond, and Y.-J. Zhang. 2021. Climate change patterns of wild blueberry fields in downeast, Maine over the past 40 years. Water 13(5):594. doi: 10.3390/w13050594.
- van Donk, S.J., D.T. Lindgren, D.M. Schaaf, J.L. Petersen, and D.D. Tarkalson. 2011. Wood chip mulch thickness effects on soil water, soil temperature, weed growth and landscape plant growth. J. Appl. Hortic 13(2):91–95. doi: 10.37855/jah.2011.v13i02.22.
- Xie, Z., Y. Wang, G. Cheng, S.S. Malhi, C.L. Vera, Z. Guo, and Y. Zhang. 2010. Particle-size effects on soil temperature, evaporation, water use efficiency and watermelon yield in fields mulched with gravel and sand in semi-arid loess plateau of northwest china. Agric. Water Manag 97(6):917–923. doi: 10.1016/j.agwat.2010.01.023.
- Xiong, D., J. Chen, T. Yu, W. Gao, X. Ling, Y. Li, S. Peng, and J. Huang. 2015. SPAD-based leaf nitrogen estimation is impacted by environmental factors and crop leaf characteristics. Sci. Rep. 5:(1:1–12. doi: 10.1038/srep13389.
- Yarborough, D.E. 2012. Establishment and management of the cultivated lowbush blueberry (Vaccinium angustifolium). Int. J. Fruit Sci 12(1–3):14–22. doi: 10.1080/15538362.2011.619130.
- Yarborough, D.E., F. Drummond, S. Annis, J. Cote, and A. Dibble. 2009. A pocket guide to IPM scouting in wild blueberries. University of Maine Cooperative Extension, Orono, ME, USA.