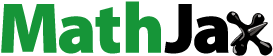
ABSTRACT
Grape downy mildew is a major grape disease that typically occurs when grape leaves are subjected to high temperature. In this study, downy mildew incidence in ‘Red Globe’ grape was investigated. To clarify the effects of grape downy mildew on grape leaves under high-temperature stress. Grape downy mildew infestation and high-temperature treatment alone or combinations were applied to ‘Red Globe’ grape. The gas exchange and chlorophyll fluorescence parameters of grape leaves were measured. The results showed that logistic growth period of grape downy mildew in the Jinzhong, China was from July 20 to August 10. After the combined stress of high temperature and grape downy mildew, photosynthetic rate (Pn), stomatal conductance (Gs), intercellular carbon dioxide concentration (Ci), maximum photochemical efficiency(Fv/Fm), actual photochemical efficiency(ΦPSII), and performance index on absorption basis (PIABS) of grape leaves were all decreased more than the sum of those indexes under the two stresses individually. At 28°C, the damage of downy mildew had no significant effect on the degree of photosystem closure of leaves, but it was significantly increased with the combined stresses. The electron transfer efficiency from photosystem II to photosystem I was decreased significantly under the combined stresses. Under 38°C high-temperature stress, the energy dissipated per active reaction center of the leaves affected by grape downy mildew was significantly increased. High temperature aggravated adverse effects of downy mildew on the structure and function of photosystem II (PSII) of grape leaves and then further exacerbated reduction of photosynthesis caused by heat stress.
Introduction
Grape downy mildew is a major grape disease that seriously affects the production of grapes and the wine industry (Blasi et al., Citation2011; Park et al., Citation1997). The main pathogen of grape downy mildew is Plasmopara viticola (Berk. et Curtis) (Gessler et al., Citation2011). Grape downy mildew caused the chlorophyll content and stomatal conductance decrease of various grape varieties, such as ‘Cabernet Sauvignon’ and ‘Sangiovese.’ Grape downy mildew also reduced the photosynthetic efficiency of grapes (Cséfalvay et al., Citation2009; Giuntoli and Orlandini Citation2000; Lu, Citation2013; Moriondo et al., Citation2005; Shtienberg, Citation1992). However, effect of grape downy mildew on the maximum photochemical efficiency (Fv/Fm) of PSII in ‘Cabernet Sauvignon’ leaves was not obvious (Lu, Citation2013).
Warmer temperature regimes in critical early period can significantly increase the opportunities for the pathogen to growth, leading to increases of downy mildew pressure in grapevine in the coming decades, even if precipitation decreases (Salinari et al., Citation2006). In China, grape downy mildew began to occur from May to June and peaked from July to September (Gilardi et al., Citation2016; Zhao, Citation2004). July and August were the period, when grape downy mildew and high temperatures occur simultaneously (Luo et al., Citation2010). Accordingly, high-temperature stress affected PSII of plant leaves (Aminaka et al., Citation2006; Lu and Zhang, Citation2000; Mathur et al., Citation2011; Tiwari and Yadav, Citation2019). high-temperature stress decreased plant leaves light efficiency (Sun et al., Citation2013; Yu et al., Citation2016a, Citation2016b) and ultimately reduced plant photosynthesis (Huang et al., Citation2017; Islam, Citation2015; Liu and Fang, Citation2020; Machino et al., Citation2021). When grape leaves were subjected to high-temperature stress, the net photosynthetic rate (Pn) decreases significantly (Greer and Weedon, Citation2013), and the structure and function of the photosystem were very adversely affected (Zhang et al., Citation2018), especially the PSII donor side and reaction center activity and the energy distribution of the photosystem (Geng et al., Citation2019; Xiao et al., Citation2017).
Under natural summer conditions, grape downy mildew and high temperatures often occur at the same time. Grapes suffered from the combined stress of these two adverse factors. Many previous studies focused on effects of either biotic or abiotic stress. There was little of research on the interaction between biotic and abiotic stress factors. There has been no report on the combined effects of biotic and abiotic stresses on grape leaves. Thus, this study used ‘Red Globe’ grapevines to test the effects of grape downy mildew on the photosynthetic efficiency and chlorophyll fluorescence parameters of grape leaves under different temperature treatments. To clarify whether grape downy mildew aggravated effects on photosynthesis of grape leaves under high temperatures. Moreover, to explore the influence mechanism of grape downy mildew and high temperature combined injury on grape photosystem. It would provide new theoretical basis for the mechanism of grape downy mildew damage.
Materials and Methods
Investigation of the Incidence of Grape Downy Mildew in the Field
In this experiment conducted at the National Grape Resource Nursery in Taigu, Shanxi, China, three plots were established, which were arranged along a north–south orientation. Each plot was 50 m long and 6.0 m wide. Two hundred ‘Red Globe’ grape (Vitis vinifera L. cv. ‘Red Globe’) seedlings were planted in each plot. Additionally, the row spacing and plant spacing are 1.5 m and 1.5 m, respectively. The seedlings were planted on April 15, 2016, and conventional cultivation management was performed in the field after transplantation. Two rows of seedlings of the highly downy mildew-resistant variety ‘Beida’ were set up at the edge of the plot as protection rows.
The investigation of diseased instance followed the methods of Yu et al. (Citation2016a). With a 5-point sampling method. Fifteen fixed grape plants in each plot were selected for downy mildew assessment. We consider two grapes were randomly selected from each plant, with all leaves investigated from the bottom up. From June 15 onward, the survey was conducted every 15 days to record the diseased leaf rate and disease index of the grape plants over two consecutive years. The field efficacy standards of the Ministry of Agriculture were used as reference for classifying diseased leaves with grape downy mildew, that is, the diseased leaves were classified into six grades according to the proportion of diseased spots across the entire leaf area (%); grade 0 corresponded to no diseased spots while grades 1, 3, 5, 7, and 9 corresponded to diseased spot areas accounting for less than 5%, 6% to 25%, 26% to 50%, 51% to 75%, and more than 75% of the entire leaf area, respectively. At the same time, the daily temperature and rainfall from May onward in each year were recorded by a small-scale weather station in the test garden.
The calculation formula for the disease index of grape downy mildew was as follows:
Determination the Photosynthesis of Grape Leaves under Different Temperature Conditions
Annual ‘Red Globe’ seedlings of the same size were chosen and planted in flowerpots with a diameter of 25 cm and a height of 35 cm filled with a 2:1:1 soil:sand:substrate mixture. When 10–12 fully expanded leaves appeared, the grape were used for treatment.
Preparation of Grape Downy Mildew Inoculum
Grape leaves with grade 3 grape downy mildew were collected from the field were used to prepare a grape downy mildew inoculum. The old sporangia on the leaves were washed away with clean water, and sterile water was then used to clean the leaves repeatedly; then, samples were kept moisturized (with the humidity controlled to 85–90%) and warm (22 ± 1°C) in a darkened incubator for 24 hours. After new sporangia grew, the sporangia were brushed into a 50 ml sterile tube with a brush treated with 75% ethanol, and sterile water was added to make a sporangia suspension. The number of spores was counted under a microscope with a hemocytometer, and then, a 2 × 104 sporangia/mL bacterial solution was prepared for later use.
Inoculation of Grape Leaves with Grape Downy Mildew
Forty ‘Red Globe’ seedlings with similar growth were prepared for the experiment. Then, five fully expanded leaves (adjacent leaves in the middle part of the plant) were chosen for inoculation with grape downy mildew. On the backs of the five leaves, a hand-held sprayer was used to spray and thus inoculate the plants with the prepared sporangia suspension. After inoculation, the leaves covered by moisturizing bags. The bags were removed after 48 h. The morbidity of grape leaves was recorded.
High Temperature Treatment
Downy mildew-inoculated plants and control plants with similar vigor (the treated plants were selected from among plants with a disease index of grade 5) were selected and placed in an illuminated incubator for 2 d (16-h days, 8-h nights). The light intensity was 800 μmol·m−2·s−1, which is a light intensity particularly suitable for grapes, according to research by (Sun et al., Citation2013), and the temperature was set to 28°C and 38°C for the control and high-temperature treatments, respectively. After treatment, the corresponding photosynthetic and fluorescence parameters of the healthy parts of the diseased leaves with grape downy mildew were measured immediately. Three leaves in the middle of each plant were selected, and six to eight plants were measured repeatedly each time.
Determination of Photosynthetic Efficiency
The healthy parts of the diseased leaves after treatment were selected, and the net photosynthetic rate (Pn), stomatal conductance (Gs), and intercellular carbon dioxide concentration (Ci) were measured using the CIRAS-2 portable photosynthetic system (PP Systems, Amesbury, MA, USA). The control light intensity was 800 μmol·m−2·s−1 and the CO2 concentration was 360 μl·L−1, with a temperature of 25°C.
Determination of Fluorescence Parameters
Healthy parts of the diseased leaves after treatment were selected, and an FMS-2 portable pulse modulation fluorometer (Hansatech, King’s Lynn, UK) was used to measure the fluorescence parameters Fo, FmFm, Fs, and Fo. Before measuring the chlorophyll fluorescence of dark-adapted leaves, the leaves were clamped in a dark adaptation clip for 20 min. To measure Fs and Fm, the FMS-2 system light source was used. Under 600 μmol·m−2·s−1 light, the material was fully photochemically activated to reach a steady state, and the steady-state fluorescence Fs was measured. Then, saturated pulse light was activated to measure Fm, and far-red light was initiated to measure Fo.
A continuous excitation fluorometer (Handy PEA, Hansatech) was used to determine the rapid chlorophyll fluorescence induction curve. The following parameters were directly obtained from the OJIP curve: Fo, the minimum fluorescence (20 μs); Fk, the fluorescence of point K (300 μs); Fj, the fluorescence of point J (2 ms); Fi, the fluorescence of point I (30 ms) fluorescence; and Fm, the maximum fluorescence between 0.3 s and 2 s. From the polyphasic chlorophyll fluorescence transient (OJIP) curve obtained by the JIP-test analysis, the following parameters were obtained: the maximum photochemical efficiency of PSII, φPo = TRo/ABS = Fv/Fm; the initial slope of the relative fluorescence curve, indicating the rate of Quinone A (QA) reduction, Mo = 4 × (Fk – Fo)/(Fm – Fo); J-point relative variable fluorescence, Vj = (Fj – Fo)/(Fm – Fo); photosynthetic performance index, PIABS = RC/ABS × [φPo/(1 – ΦPo) × φEo/(1 – φEo)]; and the number of active reaction centers per unit area, RC/CSm = Fm × φPo × (Vj/Mo) (MO is the initial slope of the OJIP curve, Mo = 4(F300μs·FO)/(FM-FO)).
Results
The Incidence of Downy Mildew on ‘Red Globe’ Grape in the Field
In 2015, 2016, 2017, and 2018, ‘Red Globe’ grape plants cultivated in the field of the National Grape Resource Nursery in Taigu, Shanxi, China began to be infected with grape downy mildew disease in June, and it became active in July and August (). The downy mildew disease index in different years had a first obvious peak from July 20 to August 10, and the incidence regularity was consistent with logistic growth. The second peak period appeared on August 29. Daily meteorological data showed that from July 15 to August 20 each year the average field temperature was stable at around 30°C for a long time (), and the maximum temperature exceeded 38°C. These data indicate confirmed that the grape leaves in the field were under the combined stress of high temperature and downy mildew.
Gas Exchange Parameters
Under different temperature conditions, grape downy mildew caused a decrease in net photosynthetic rate (Pn) of leaves (). At 28°C and 38°C, grape downy mildew caused a decrease in Pn of 23.59% and 45.63%, respectively, compared to the control, while the stomatal conductance (Gs) and intercellular carbon dioxide concentration (Ci) were not significantly different from the control (). Pn after high-temperature stress decreased by 35.54% compared with the control. Under 38°C high-temperature stress, Pn, Gs, and Ci of ‘Red Globe’ grape leaves infected with grape downy mildew reduced by 71.28%, 64.29%, and 29.85%, respectively, compared with healthy plants at 28°C (). This indicated that the decline in Pn for grape leaves under the combined stress of high temperature and downy mildew was greater than the sum of the decline under each stress individually.
Figure 3. Effects of grape downy mildew on net photosynthetic rate, stomatal conductance and intercellular CO2 concentration of leaves after different temperature treatments. DM+HT represents downy mildew and high temperature treatment, CK+HT represents only high temperature treatment, DM+LT represents downy mildew and normal temperature treatment, CK+LT represents normal temperature treatment. Data in the figure are mean ±standard error. The numerical values with different letters are significantly different (P < .05).
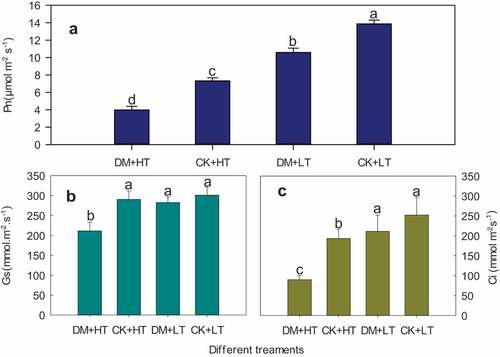
ΦPSII, PIABS, Fv/Fm, and Fv/FO of Grape Leaves
The ΦPSII reflects the actual photochemical efficiency of the leaves when the PSII reaction center is partially closed under illumination, that is, the proportion of the energy used by the plant leaves for electron transfer under illumination to be captured as light energy. Grape downy mildew caused decrease in ΦPSII of grape leaves. At 28°C and 38°C, the downy mildew damage caused decreases of 33.94% and 67.77%, respectively (). Under 38°C, ΦPSII of leaves infested by grape downy mildew reduced by 80.58% (). It indicated that grape downy mildew reduced the ratio of energy used for electron transfer to light energy captured of leaves, and high temperature aggravated this adverse effect.
Figure 4. Effects of grape downy mildew on ΦPSII, PIABS, Fv/Fm and Fv/Fo of leaves after different temperature treatments. DM+HT represents downy mildew and high temperature treatment, CK+HT represents only high temperature treatment, DM+LT represents downy mildew and normal temperature treatment, CK+LT represents normal temperature treatment. Data in the figure are mean ±standard error. The numerical values with different letters are significantly different (P < .05).
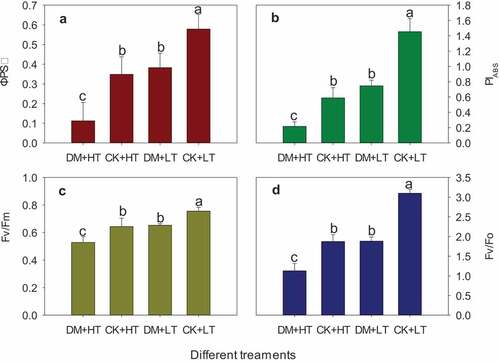
PIABS is a parameter that reflects the comprehensive photosynthetic performance of plants (Ghorbanzadeh et al., Citation2020; Hermans et al., Citation2003; Moosavi-Nezhad et al., Citation2021). It is also one of the most sensitive indicators reflecting possible damage to the photosynthetic apparatus (Bussotti et al., Citation2007; Li et al., Citation2005). Grape downy mildew damage caused a decrease in the PIABS value of grape leaves (). The PIABS values of ‘Red Globe’ leaves at 28°C and 38°C reduced by 45.67% and 71.21%, respectively (). Under combined stress, the PIABS value of ‘Red Globe’ grape leaves was reduced by 85.20% compared to healthy leaves at 28°C. High temperature aggravated the effect of grape downy mildew on the photosynthetic apparatus of grape leaves.
Fv/Fm is the maximum photochemical quantum yield of PSII, or the maximum light energy conversion efficiency of PSII. Its value represents the maximum efficiency of the photosynthetic apparatus using absorbed light energy for photochemical reactions, and it reflects the integrity of the light energy absorption and conversion mechanism (Ghorbanzadeh et al., Citation2020; Johnson et al., Citation1993; Munekage et al., Citation2004). The damage of grape downy mildew under different temperature all resulted in a decrease in the Fv/Fm value of grape leaves. The Fv/Fm values of ‘Red Globe’ leaves at 28°C and 38°C were reduced by 17.99% and 19.08%, respectively (). At 38°C, the Fv/Fm value of ‘Red Globe’ leaves damaged by grape downy mildew was reduced by 33.64% compared with healthy leaves at 28°C ().
The potential activity of the light reaction center of PSII (Fv/Fo) represents the potential activity of PSII. Grape downy mildew reduced the Fv/Fo of leaves under different temperature conditions (). At 38°C, the Fv/Fo of ‘Red Globe’ grape leaves damaged by grape downy mildew was decreased by 63.56% compared to healthy leaves at 28°C ().
These results indicated that both downy mildew and high temperature can reduce the PSII maximum photochemical efficiency and potential activity of grape leaves, and the degree of inhibition was increased when grapes were under the combined stresses of high temperature and downy mildew.
1-qP, RC/CSm, and WK of Leaves under Different Temperature Treatments
The 1-qP value reflects the closure degree of the photosystem reaction center and serves as an indicator of the redox state within the electron transport chain (Maxwell and Johnson, Citation2000). At 28°C, grape downy mildew damage had no significant effect on the 1-qP of ‘Red Globe’ grape leaves, while the 1-qP of ‘Red Globe’ grape leaves damaged by grape downy mildew increased by 39.12% and 115.08% compared to the control plants under 38°C high-temperature stress and 28°C, respectively (). However, high-temperature stress alone caused an increase of 54.61% in the 1-qP (). This indicated that grape downy mildew had no effect on the closure degree of the photosystem reaction center of grape leaves under moderate temperature conditions. High temperatures can cause the closure of the photosystem center of grape leaves, and the combined stress of high temperature and grape downy mildew intensified the closure degree of the photosystem reaction center.
Figure 5. Effects of grape downy mildew on 1-qP, RC/CSm and WK of leaves after different temperature treatments. DM+HT represents downy mildew and high temperature treatment, CK+HT represents only high temperature treatment, DM+LT represents downy mildew and normal temperature treatment, CK+LT represents normal temperature treatment. Data in the figure are mean ±standard error. The numerical values with different letters are significantly different (P < .05).
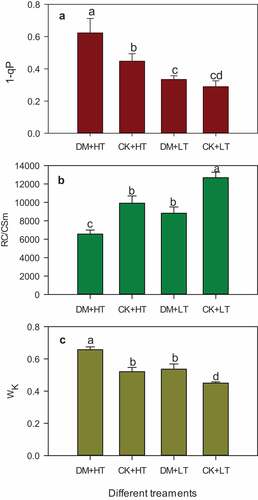
The density of PSII reaction centers per excited cross-section (RC/CSm) represents the number of active photosynthetic reaction centers per unit intercept area. Under 28°C and 38°C, the RC/CSm of leaves with grape downy mildew were reduced by 30.37% and 33.77%, respectively, compared with their control. Under 38°C high-temperature stress, RC/CSm of leaves infested by grape downy mildew was reduced by 48.25% compared with the healthy leaves at 28°C ().
WK reflects the relative fluorescence of point K. It can reflect the degree of damage to the oxygen-evolving complex (OEC) (Strasser, Citation1997). Under suitable temperature conditions, the WK of leaves damaged by grape downy mildew was increased by 19.17%; under the combined stress, the WK of leaves was increased by 45.91% compared with that of healthy leaves at 28°C ().
PSII Function
ETR is the quantification of electron transfer efficiency from PSII to PSI (Munekage et al., Citation2004). Under 28°C, the ETR of ‘Red Globe’ grape leaves damaged by grape downy mildew was not significantly different from that of healthy controls. Under 38°C, it was reduced by 28.51% (). The ETR of leaves damaged by grape downy mildew at 38°C was decreased by 44.72% compared to healthy leaves at 28°C (). This indicated that grape downy mildew had no significant effect on the electron transfer from PSII to PSI in grape leaves under suitable temperature, while grape downy mildew significantly reduced the electron transfer efficiency from PSII to PSI in grape leaves under high-temperature stress.
Figure 6. Effects of grape downy mildew on ETR and energy distribution of leaves after different temperature treatments. DM+HT represents downy mildew and high temperature treatment, CK+HT represents only high temperature treatment, DM+LT represents downy mildew and normal temperature treatment, CK+LT represents normal temperature treatment. Data in the figure are mean ±standard error. The numerical values with different letters are significantly different (P < .05).
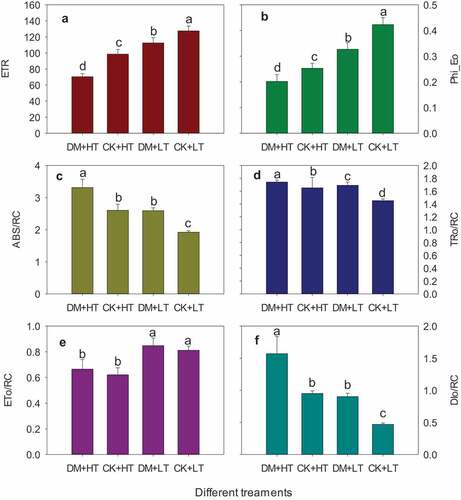
Under different temperature conditions, the damage of downy mildew caused an increase in the absorption per active reaction centers (ABS/RC) of grape leaves. At 28°C and 38°C, the ABS/RC of leaves damaged by grape downy mildew increased by 34.95% and 27.36% (). Under 38°C, the ABS/RC of leaves infested by grape downy mildew increase by 72.48% compared with healthy leaves at 28°C ().
The energy captured by the unit reaction center (TRo/RC)of ‘Red Globe’ grape leaves damaged by downy mildew for reducing QA was significantly increased compared to the control leaves at the suitable temperature, but the differences between temperature treatments were not significant. Under suitable temperature conditions, the ratio of TRo/RC to ABS/RC of healthy leaves was 75.57%, while the ratio of leaves damaged by downy mildew was decreased to 65.25%, and the ratio of leaves damaged by grape downy mildew was 52.57% under high-temperature stress ().
The probability that an absorbed photon will move an electron into the electron transport chain beyond QA− (ψEo) value reflects the energy ratio of light energy captured for electron transfer after QA (Moosavi-Nezhad et al., Citation2021). Under suitable temperature conditions, the ψEo value of leaves damaged by grape downy mildew was reduced by 22.70%. Under the combined stress of high temperature and grape downy mildew, ψEo was reduced by 20.23%, with a decline of 52.32% compared to healthy leaves at 28°C ().
Under different temperature conditions, the energy used for electron transfer (ETo/RC) captured by the unit reaction center of ‘Red Globe’ leaves damaged by grape downy mildew was not significantly different from that of healthy controls. The ETo/RC of leaves damaged by grape downy mildew at 38°C was decreased by 18.00% compared with healthy leaves at 28°C (). Under suitable temperature conditions, the ratio of ETo/RC to ABS/RC of healthy leaves was 42.26%, while the corresponding ratio of leaves damaged by downy mildew was decreased to 32.71%, and the ratio under the combined stress of high temperature and grape downy mildew was 20.09% ().
Under suitable temperature conditions, the energy dissipated by heat dissipation in the unit reaction center of ‘Red Globe’ leaves damaged by downy mildew (DIo/RC) was increased by 92.11% compared to the control. The DIo/RC values of leaves damaged by downy mildew under 38°C high-temperature stress were increased by 65.33% and 234.90%, respectively, compared with control leaves and healthy leaves at 28°C ().
Discussion
Grape downy mildew was one of the main diseases of grapes. Occurring in major grape producing areas in China, it was also one of the factors restricting the high and stable production of grapes in China (Lu, Citation2013). The prevalence of grape downy mildew tended to differ among regions of China. The logistic index growth period in Northeast China was from late July to late August(Bai and Long, Citation2019; Moriondo et al., Citation2005), while in Ningxia and the Beijing area, it was from August 9 to October 10(Li, Citation2019) and late August to early September(Dandan et al., Citation2017), respectively. In this study, the four-year survey in 2015, 2016, 2017, and 2018, revealed the occurrence of “Red Globe” grape downy mildew in the Jinzhong area of Shanxi Province, indicating that the logistic growth period was from July 20 to August 10, which was similar to that in Northeast China.
The prevalence of grape downy mildew was closely related to temperature, rainfall, the number of days with rainfall, and humidity in a vineyard (Burruano, Citation2000; Kennelly et al., Citation2007). Global warming was accelerating in major wine-producing countries (Aoki et al., Citation2021). A simulation model has predicted an average temperature increase of 2°C in viticulture regions over the next 50 years (Jones et al., Citation2005). In the past 10 years, the occurrence of high temperatures in both the north and south of China has increased significantly compared to those in the 1980s and 1990s, and it mainly occurred in July and August (Pan, Citation2010). Long-term high temperatures of 35–40°C have occurred in many provinces, and extreme high temperatures above 40°C have even emerged (Pan, Citation2010). Similarly, a meteorological observatory in Japan has announced that the average temperature in the Yamanashi Prefecture, the major producer of white and red wines in Japan, has risen by 0.042°C every year from 1961 to date (Saito et al., Citation2008). This indicates that grape plants were currently subjected to combined stresses of high-temperature weather and downy mildew during the production process in the field.
Photosynthesis was the most important physiological process of plants (Seif et al., Citation2021; Tan et al., Citation2009) as well as the most important chemical reaction on earth. It was also one of the physiological processes in which plants are most sensitive to various biotic and abiotic stresses (J.y et al., Citation2006). High-temperature stress caused damage to the photosynthetic apparatus of ‘Cabernet Sauvignon’ grape leaves, influencing the photosynthetic efficiency of plants (Nazar et al., Citation2011). High temperature caused a significant reduction in Pn and stomatal conductance of grape leaves (Gilardi et al., Citation2016). In this study, Pn and stomatal conductance of ‘Red Globe’ grape leaves were reduced by high-temperature stress, but the intercellular carbon dioxide concentration did not change significantly. it indicated that the reduction in photosynthesis of ‘Red Globe’ grapes under high temperature was not caused by stomatal limitation, which was consistent with the results of previous studies(Greer and Weedon, Citation2013; Nazar et al., Citation2011). This study also revealed that the damage of grape downy mildew under different temperature conditions always led to a decrease in Pn of leaves, and under suitable temperature conditions, photosynthesis was decreased, even though stomatal conductance and intercellular carbon dioxide concentration did not change significantly. These results indicated that the decrease in Pn of leaves damaged by downy mildew under suitable conditions was not caused by stomatal limitation. The combined stress of high temperature and grape downy mildew damage resulted in decreased stomatal conductance and intercellular carbon dioxide concentration of grape leaves. In turn, it showed that stomatal limitation became one of the main factors causing Pn reductions in grape leaves under the combined stress of high temperatures and downy mildew, while high temperatures aggravated the adverse effects of grape downy mildew on photosynthesis in leaves.
The rapid chlorophyll fluorescence induction curve can be used to nondestructively assay the biochemical process of plant photosynthesis, which is an approach often employed to study the effects of various adverse stresses on plants (Li et al., Citation2005; Shahbazi et al., Citation2021). Grape downy mildew is a major leaf disease of grapes. In this study, it was found that the maximum photochemical efficiency (Fv/Fm) and photosynthetic performance index (PIABS) of grape leaves damaged by grape downy mildew were significantly reduced, indicating that both the structure and function of photosystem II in grape leaves were adversely affected. The number of active reaction centers per unit area (RC/CSm), and the energy ratio (ΨEo) that captures light energy for electron transfer after QA were decreased significantly, while the closure degree of the reaction centers (1-qP) was increased, indicating that after grape downy mildew damage, both the donor side and the receptor side of the photosystem of the grape leaves were subjected to photoinhibition. In particular, the increase in the WK of the grape leaves indicated that the oxygen-evolving complex (OEC) on the donor side was damaged. After downy mildew damage, the proportion of energy used for QA reduction (TRo/RC) to the energy absorbed by the unit reaction center of the leaf photosystem (ABS/RC) and the proportion of the energy used for electron transfer (ETo/RC) to ABS/RC were both decreased significantly. These observations indicated that the proportion of light energy absorbed by the active center of grape leaves used for photochemical reactions was decreased, while the energy dissipated by heat dissipation (DIo/RC) was increased significantly. This showed that the leaves could reduce excess light energy by increasing heat dissipation to protect the photosynthetic structures of grape leaves. Although heat dissipation increased, the actual photochemical efficiency (ΦPSII) was also decreased significantly after grape downy mildew damage, indicating that the utilization rate of excitation energy in the photochemical reaction process was significantly reduced, and the heat dissipation response alone was able to remove a large amount of excess light energy in grape leaves, causing PSII damage of all physiological processes in plants, photosynthesis is among the most sensitive to high-temperature stress. High-temperature stress damages both the donor side and the receptor side of PSII in ‘Cabernet Sauvignon’ grape leaves (Zhao, Citation2004; Gilardi et al., Citation2016). The present study indicates that high-temperature stress has an adverse effect on the structure and function of the photosystem of ‘Red Globe’ grape leaves, and both the donor side and the receptor side of the photosystem were inhibited, with the heat dissipation of the leaves also increased, resulting in an increase in the accumulation of reactive oxygen and damage to PSII, which was basically consistent with the results of previous studies.
Although high-temperature stress can inhibit the occurrence of downy mildew, owing to the coexistence of high temperature and rainfall in summer, grape leaves in summer suffer from the combined stresses of high temperature and downy mildew. This study indicated that high-temperature stress exacerbated the adverse effects of downy mildew on the structure and function of the photosystem of grape leaves. Under high-temperature stress, the reduction amplitude of Fv/Fm and PIABS of downy mildew damaged leaves exceeded the summed effects of the two stresses individually, indicating that high-temperature stress exacerbated the adverse effects of downy mildew on the structure and function of the photosystem of grape leaves. The photosynthetic electron transport chain and cytoplasmic membrane of plants are damaged by high-temperature stress(Wise et al., Citation2004), with damage to D1 proteins and reductions to the activity levels of enzymes related to the dark reaction process of photosynthesis, which further reduces the Pn of plant leaves(Makino and Sage, Citation2007). Under the combined stress of high temperature and downy mildew, the DIo/RC of grape leaves was increased sharply, indicating that the grape leaves handled excess light energy through heat dissipation to protect the photosynthetic structure of leaves. However, owing to the oxidation damage caused by high temperature to the photosynthetic apparatus, cytoplasmic membrane, and photosynthetic electron transport chain, the potential activity of PSII (Fv/Fo) and the electron transfer efficiency (ETR) from PSII to PSI were decreased, and the closure degree of the reaction center of the photosystem was also increased, causing the proportion of TRo/RC and ETo/RC to ABS/RC to be significantly decreased. This indicated that the proportion of light energy absorbed by the photosynthetic apparatus of the grape leaves for photochemical reactions was significantly reduced, and more excess light energy in the photosynthetic structure was thus accumulated. The heat dissipation under the stress of downy mildew alone was insufficient to consume the excess light energy, and in conjunction with high-temperature stress, the excess light energy was accumulated sharply, producing excessive levels of reactive oxygen species and destroying the antioxidant system and photosynthetic mechanism of grape leaves, thereby increasing the damage to the photosystem.
Conclusion
The logistic growth period of ‘Red Globe’ grape downy mildew in the Jinzhong region of Shanxi Province in China was determined to span from July 20 to August 10. Under the combined stress of high temperature and downy mildew infection, the decrease in Pn of grape leaves was greater than the summed effect of the two factors individually, and stomatal limitation was one of the major mechanisms affecting Pn.
After grape downy mildew damaged, the structure and function of PSII were adversely affected. The donor and receptor sides of the photosystem both subjected to photoinhibition; both the energy distribution and electron transfer of the photosystem of grape leaves were affected. The heat dissipation of the leaves was increased, but the heat dissipation method could not handle the large excess of light energy in the grape leaves, resulted in PSII damage. After the dual stress of high temperature and grape downy mildew, the excess light energy increased sharply, while the reductions in photosystem electron transfer efficiency and the potential activity of the photosystem were intensified. Additionally, the degree of closure of photosystem was significantly increased, which increased the reactive oxygen species content, thereby increasing damage to the photosynthetic apparatus. Thus, the reduction in the photosynthesis of grape leaves under the combined stress was greater than the sum of the stresses of high temperature and grape downy mildew individually. It indicated that high temperature aggravated the adverse effects of grape downy mildew on photosynthesis in grape leaves.
Acknowledgments
We thank Mr. Qifeng Zhao from Pomology Institute, Shanxi Academy of Agricultural Sciences for providing seedlings of the ‘Red Globe’ grape (Vitis vinifera L. cv. ‘Red Globe’).
Disclosure Statement
No potential conflict of interest was reported by the author(s).
Additional information
Funding
References
- Aminaka, R., Y. Taira, Y. Kashino, H. Koike, and K. Satoh. 2006. Acclimation to the growth temperature and thermosensitivity of photosystem II in a mesophilic cyanobacterium, synechocystis sp PCC6803. Plant Cell Physiol. 47(12):1612–1621. doi: 10.1093/pcp/pcl024.
- Aoki, Y., A. Usujima, and S. Suzuki. 2021. High night temperature promotes downy mildew in grapevine via attenuating plant defence response and enhancing early Plasmopara viticola infection. Plant Prot. Sci. 57(1):21–30. doi: 10.17221/92/2020-PPS.
- Bai, Y.J., and M.G. Long. 2019. Studies on the temporal dynamic model of epidemic and economic threshold of grape downy mildew. J. Jilin Agric. Univ. 42(3):7.
- Blasi, P., S. Blanc, S. Wiedemann-Merdinoglu, E. Prado, E.H. Ruhl, P. Mestre, and D. Merdinoglu. 2011. Construction of a reference linkage map of Vitis amurensis and genetic mapping of Rpv8, a locus conferring resistance to grapevine downy mildew. Theor. Appl. Genet. 123(1):43–53. doi: 10.1007/s00122-011-1565-0.
- Burruano, S. 2000. The life-cycle of Plasmopara viticola, cause of downy mildew of vine. Mycologist 14(4):179–182. doi: 10.1016/S0269-915X(00)80040-3.
- Bussotti, F., R.J. Strasser, and M. Schaub. 2007. Photosynthetic behavior of woody species under high ozone exposure probed with the JIP-test: A review. Environ. Pollut. 147(3):430–437. doi: 10.1016/j.envpol.2006.08.036.
- Cséfalvay, L., G.G. Di, K. Matouš, D. Bellin, B. Ruperti, and J. Olejníčková. 2009. Pre-symptomatic detection of Plasmopara viticola infection in grapevine leaves using chlorophyll fluorescence imaging. Eur. J. Plant Pathol. 125(2):291–302. doi: 10.1007/s10658-009-9482-7.
- Dandan, X.H.T., L. Yanyan, J. Meng, W. Qi, and W.G. Ling. 2017. Epidemic regularity and two prediction models of Plasmopara viticola in Yanqing of Beijing. Chin. Agric. Sci. Bulletin 2017:13.
- Geng, Q., H. Xing, H. Zhai, Y. Du, and E. Jiang. 2019. Effects of different light intensity and temperature on psii photochemical activity in ‘cabernet sauvignon’grape leaves under ozone stress. Scientia Agricultura Sinica 52(7):1183–1191.
- Gessler, C., I. Pertot, and M. Perazzolli. 2011. Plasmopara viticola: A review of knowledge on downy mildew of grapevine and effective disease management. Phytopathol. Mediterr. 50(1):3–44.
- Ghorbanzadeh, P., S. Aliniaeifard, M. Esmaeili, M. Mashal, B. Azadegan, and M. Seif. 2020. Dependency of growth, water use efficiency, chlorophyll fluorescence, and stomatal characteristics of lettuce plants to light intensity. J. Plant Growth Regul. 40:1–17.
- Gilardi, G., M. Pugliese, W. Chitarra, I. Ramon, M.L. Gullino, and A. Garibaldi. 2016. Effect of elevated atmospheric CO 2 and temperature increases on the severity of basil downy mildew caused by Peronospora belbahrii under phytotron conditions. J. Phytopathol. 164(2):114–121. doi: 10.1111/jph.12437.
- Giuntoli, A., S. Orlandini. 2000. Effects of Downy Mildew on Photosynthesis of Grapevine Leaves, Acta Hort. 461–466.
- Greer, D.H., and M.M. Weedon. 2013. The impact of high temperatures on Vitis vinifera cv. Semillon grapevine performance and berry ripening. Front Plant Sci 4:491. doi:10.3389/fpls.2013.00491.
- Hermans, C., M. Smeyers, R.M. Rodriguez, M. Eyletters, R.J. Strasser, and J.P. Delhaye. 2003. Quality assessment of urban trees: A comparative study of physiological characterisation, airborne imaging and on site fluorescence monitoring by the OJIP-test. J. Plant Physiol. 160(1):81–90. doi: 10.1078/0176-1617-00917.
- Huang, G.J., Q.Q. Zhang, X.H. Wei, S.B. Peng, and Y. Li. 2017. Nitrogen can alleviate the inhibition of photosynthesis caused by high temperature stress under both steady-state and flecked irradiance. Front Plant Sci. 8: doi:10.3389/fpls.2017.00945.
- Islam, M.T. 2015. Effects of high temperature on photosynthesis and yield in mungbean. Bangladesh J. Bot. 44(3):451–454. doi: 10.3329/bjb.v44i3.38553.
- Johnson, G., A. Young, J. Scholes, and P. Horton. 1993. The dissipation of excess excitation energy in British plant species. Plant Cell Environ. 16(6):673–679. doi: 10.1111/j.1365-3040.1993.tb00485.x.
- Jones, G.V., M.A. White, O.R. Cooper, and K. Storchmann. 2005. Climate change and global wine quality. Clim. Change 73(3):319–343. doi: 10.1007/s10584-005-4704-2.
- Kennelly, M.M., D.M. Gadoury, W.F. Wilcox, P.A. Magarey, and R.C. Seem. 2007. Primary infection, lesion productivity, and survival of sporangia in the grapevine downy mildew pathogen Plasmopara viticola. Phytopathology 97(4):512–522. doi: 10.1094/PHYTO-97-4-0512.
- Li, P.M., H.Y. Gao, and R.J. Strasser. 2005. Application of the fast chlorophyll fluorescence induction dynamics analysis in photosynthesis study. J. Plant Physiol. Mol. Biol. Plants 31(6):559–566.
- Li, W. 2019. Methods of predicting wine grape downy mildew in the eastern foot of helan mountain. Ning Xia University, Ning Xia.
- Liu, M., and Y. Fang. 2020. Effects of heat stress on physiological indexes and ultrastructure of grapevines. Scientia Agricultura Sinica 53(7):15.
- Lu, C.M., and J.H. Zhang. 2000. Photosystem II photochemistry and its sensitivity to heat stress in maize plants as affected by nitrogen deficiency. J. Plant Physiol. 157(1):124–130. English doi:10.1016/S0176-1617(00)80145-5.
- Lu, L. 2013. Grapevine carbernet sauvignon’s changes of physiology and biochemistry after mildew downy infection hebei province. Hebei Normal University of Science and Technology, China.
- Luo, H., L. Ma, W. Duan, S. Li, and L. Wang. 2010. Influence of heat stress on photosynthesis in Vitis vinifera L. cv. Cabernet Sauvignon. Scientia Agricultura Sinica 43(13):2744–2750.
- Machino, S., S. Nagano, and K. Hikosaka. 2021. The latitudinal and altitudinal variations in the biochemical mechanisms of temperature dependence of photosynthesis within Fallopia japonica. Environ. Exp. Bot. 181:104248. doi:10.1016/j.envexpbot.2020.104248.
- Makino, A., and R.F. Sage. 2007. Temperature response of photosynthesis in transgenic rice transformed with ‘sense’or ‘antisense’rbc S. Plant Cell Physiol. 48(10):1472–1483. doi: 10.1093/pcp/pcm118.
- Mathur, S., S.I. Allakhverdiev, and A. Jajoo. 2011. Analysis of high temperature stress on the dynamics of antenna size and reducing side heterogeneity of Photosystem II in wheat leaves (Triticum aestivum) [Article]. Biochimica Et Biophysica Acta-Bioenergetics 1807(1):22–29. English doi:10.1016/j.bbabio.2010.09.001.
- Maxwell, K., and G.N. Johnson. 2000. Chlorophyll fluorescence—a practical guide. J. Exp. Bot. 51(345):659–668. doi: 10.1093/jexbot/51.345.659.
- Moosavi-Nezhad, M., R. Salehi, S. Aliniaeifard, G. Tsaniklidis, E.J. Woltering, D. Fanourakis, K. Żuk-Gołaszewska, and H.M. Kalaji. 2021. Blue light improves photosynthetic performance during healing and acclimatization of grafted watermelon seedlings. Int J Mol Sci 22:8043. doi:10.3390/ijms22158043.
- Moriondo, M., S. Orlandini, A. Giuntoli, and M. Bindi. 2005. The effect of downy and powdery mildew on grapevine (Vitis vinifera L.) leaf gas exchange. J. Phytopathol. 153(6):350–357. doi: 10.1111/j.1439-0434.2005.00984.x.
- Munekage, Y., M. Hashimoto, C. Miyake, K.I. Tomizawa, T. Endo, M. Tasaka, and T. Shikanai. 2004. Cyclic electron flow around photosystem I is essential for photosynthesis. Nature 429(6991):579–582. doi: 10.1038/nature02598.
- Nazar, R., N. Iqbal, S. Syeed, and N.A. Khan. 2011. Salicylic acid alleviates decreases in photosynthesis under salt stress by enhancing nitrogen and sulfur assimilation and antioxidant metabolism differentially in two mungbean cultivars. J. Plant Physiol. 168(8):807–815. doi: 10.1016/j.jplph.2010.11.001.
- Pan, X. 2010. Study on the heat wave and sultry weather in summer of eastern China area for past 30 years. Shanghai Normal University, Shang Hai.
- Park, E.W., R.C. Seem, D.M. Gadoury, and R.C. Pearson. 1997. DMCAST: A prediction model for grape downy mildew development. Vitic Enol Sci 52:182–189.
- Saito N, Inomata M, Tomita A, Tezuka, T, Murakami, Y, Hagihara, E, Udo, Y, Global warming tendency in recent years and influence forecasts to fruit production in Yamanashi in Proceedings of the Annual Meeting of the Society of Agricultural Meteorology of Japan. 2008: 81.
- Salinari, F., S. Giosue, F.N. Tubiello, A. Rettori, V. Rossi, F. Spanna, C. Rosenzweig, and M.L. Gullino. 2006. Downy mildew (Plasmopara viticola) epidemics on grapevine under climate change. Global Change Biol. 12(7):1299–1307. English doi:10.1111/j.1365-2486.2006.01175.x.
- Seif, M., S. Aliniaeifard, M. Arab, Z. Mehrjerdi, A. Shomali, D. Fanourakis, T. Li, and E. Woltering. 2021. Monochromatic red light during plant growth decreases the size and improves the functionality of stomata in chrysanthemum. Funct. Plant Biol. 48:515–528. doi:10.1071/FP20280.
- Shahbazi, M., M. Tohidfar, S. Aliniaeifard, F. Yazdanpanah, and M. Bosacchi. 2021. Transgenic tobacco co-expressing flavodoxin and betaine aldehyde dehydrogenase confers cadmium tolerance through boosting antioxidant capacity. Protoplasm 258(04): 1–15.
- Shtienberg, D. 1992. Effects of foliar diseases on gas-exchange processes - a comparative-study. Phytopathology 82(7):760–765. English doi:10.1094/Phyto-82-760.
- Strasser, B.J. 1997. Donor side capacity of photosystem II probed by chlorophyll a fluorescence transients. Photosyn. Res. 52(2):147–155. doi: 10.1023/A:1005896029778.
- Sun, Y.J., Y.D. Fu, Y.P. Du, and H. Zhai. 2013. Effects of different temperature and light treatments on photosynthetic system II in vitis vinifera L. cv. Cabernet Sauvignon [J]. Scientia Agricultura Sinica 46(06):1191–1200 .
- Tan, W., Q. Li, Y. Luo, W. Wang, and X. Yang. 2009. Alleviative effects of exogenous CaCl2 on the inhibition of photosynthesis induced by heat stress in tobacco. Scientia Agricultura Sinica 42(11):3871–3879.
- Tiwari, Y.K., and S.K. Yadav. 2019. High temperature stress tolerance in maize (Zea mays L.): physiological and molecular mechanisms. J. Plant Biol. 62(2):93–102. doi: 10.1007/s12374-018-0350-x.
- Wise, R., A. Olson, S. Schrader, and T. Sharkey. 2004. Electron transport is the functional limitation of photosynthesis in field‐grown Pima cotton plants at high temperature. Plant Cell Environ. 27(6):717–724. doi: 10.1111/j.1365-3040.2004.01171.x.
- Xiao, F., Z. Yang, and K. Lee. 2017. Photosynthetic and physiological responses to high temperature in grapevine (Vitis vinifera L.) leaves during the seedling stage. J. Hortic. Sci. Biotechnol. 92(1):2–10. doi: 10.1080/14620316.2016.1211493.
- Yu, S., C. Liang, L. Liu, C. Liu, and J. Fu. 2016a. Correlation among grape downy mildew epidemic rate, airborne sporangium density of Plasmopara viticola and environmental factors in Shenyang. Acta Phytophylacica Sinica 43(3):434–441.
- Yu, S., C. Liu, H. Wang, L. Liu, and T. Guan. 2016b. Effect of rain-shelter cultivation on temporal and spatial dynamics of airborne sporangia of plasmopara viticola. Sci. Agric. Sin. 49:1892–1902.
- Yun J. Y, D. Yang, and H.L. Zhao. 2006. Research progress in the mechanism for drought and high temperature to affect plant photosynthesis. Acta Botanica Boreali-Occidentalia Sinica 26(3): 641–648.
- Zhang, K., B.H. Chen, H. Yan, Y. Rui, and Y.A. Wand. 2018. Effects of short-term heat stress on PSII and subsequent recovery for senescent leaves of Vitis vinifera L. cv. Red Globe. J. Integr. Agr. 17(12):2683–2693. doi: 10.1016/S2095-3119(18)62143-4.
- Zhao K H. 2004. A Color Atlas of Grape Diseases and Pests. Beijing: China Agriculture Press