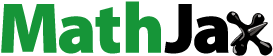
ABSTRACT
The aim of this study was to investigate the effects of pre-harvest gibberellic acid (GA3) and calcium chloride (CaCl2) and post-harvest modified atmosphere packaging (MAP) applications on fruit quality of sweet cherry during the cold storage. At the end of the cold storage, GA3+CaCl2 applications in MAP-applied fruit and CaCl2 in non-MAP-applied fruit significantly delayed weight loss compared to the control. During the cold storage, the decay of CaCl2-applied fruit has not been observed. At the end of cold storage, the a* and firmness values of the fruit treated with GA3 and CaCl2 were significantly higher than the control. The CaCl2-applied fruit had less soluble solids than the control, but they had significantly higher acidity compared to other applications. In MAP, GA3- and CaCl2-applied fruit had higher vitamin C and antioxidant activity values than the control fruit, but their total phenolics were higher. However, the total flavonoids of CaCl2-applied fruit were higher than the control. On fruit in non-MAP, with GA3 application, less anthocyanin was obtained compared to the control. As a result, GA3-treated fruit had a relatively lower bioactive compound content compared to the fruit of the CaCl2 and control applications both during the harvest and the cold storage. However, it has been revealed that GA3 and CaCl2 applications can be used as significant pre-harvest tools to delay the loss of the fruit firmness.
Introduction
Sweet cherry is one of the most-accepted fruit species by consumers due to its excellent quality. In sweet cherry, fruit size, appearance, taste, firmness and storability are very significant properties in the marketing of the fresh fruit (Aglar et al., Citation2019; Serrano et al., Citation2005). Sweet cherry fruit is more sensitive due to its physical properties, and its losses in the cold storage after harvest are high. Therefore, extending the shelf life of sweet cherry is the most valuable goal for the cherry industry (Aglar et al., Citation2017; Wani et al., Citation2014).
Fruit firmness in sweet cherry is one of the most significant factors affecting fruit quality and post-harvest life. Therefore, the main objective of extending the post-harvest life should be to increase the fruit firmness. Plant growth regulators (PGR) such as gibberellic acid (GA3) are widely used for this target (Correia et al., Citation2019; Ozturk et al., Citation2019a). GA3, which promotes cell division and elongation, are known as regulators of certain biological processes in the plant and fruit development stage (Choi et al., Citation2002; Pharis and King, Citation1985) and are therefore widely used to increase fruit size and quality (Fortes et al., Citation2015), and contribute to the maintenance of the fruit firmness due to its effect on the cell wall (Choi et al., Citation2002). In previous studies (Choi et al., Citation2002; Clayton et al., Citation2006), it was determined that with pre-harvest GA3 application in sweet cherry, the harder and larger, hence higher-quality fruits were obtained. Also, Ozkaya et al. (Citation2006) stated that sweet cherry fruit quality during cold storage could be maintained with pre-harvest gibberellic acid treatments (10, 20 and 30 mgL−1).
Calcium application in sweet cherry is one of the most effective methods to prolong the cold storage life by increasing the resistance mechanism and to prevent cracking, which is one of the most significant problems leading to the losses of the fruit quality. Calcium is a significant element in the structure of the cell wall and contributes to the mechanical properties of plant tissues (Correia et al., Citation2019). Calcium applications, either pre-harvest as a spray or post-harvest as a dip, are a common tool for maintaining or improving the post-harvest quality of sweet cherry fruit (Wang et al., Citation2014; Zoffoli et al., Citation2017). Pre-harvest calcium application has been shown to directly improve the fruit quality of sweet cherry (Dong et al., Citation2019). In some studies (Brown et al., Citation1995; Wermund et al., Citation2001), it has been found that pre-harvest calcium application increases fruit firmness and reduces fruit cracking in sweet cherry. In addition, the fruit with lower Ca levels are more susceptible to softening, pitting, and the decay processes that may follow damage to skin integrity (De Freitas and Mitcham, Citation2012). The pre-harvest bioregulators application is widely used to extend the post-harvest life of fruit species (Correia et al., Citation2019).
Recently, it has been reported that some post-harvest applications such as MAP in stone fruit have been effective in prolonging the cold storage time and minimizing the fruit quality losses in the cold storage. It has been revealed that MAP, which is applied to sweet cherry to extend the cold storage time and to minimize the fruit quality losses in the cold storage, can be used as a potential tool to maintain post-harvest fruit quality (Ozturk et al., Citation2019a; Petracek et al., Citation2002; Serrano et al., Citation2005).
Studies conducted on the effect of pre-harvest GA3 and calcium applications on reducing the cracking problem in sweet cherry and on the effect of post-harvest MAP application on maintaining fruit quality properties in the cold storage are limited. Therefore, the aim of this study was to determine the effects of combined GA3, CaCl2 and modified atmosphere packing (MAP) applications on the quality properties of sweet cherry fruit during cold storage.
Materials and methods
Plant material
The study was conducted in 2017 on sweet cherry trees planted in Tokat Gaziosmanpasa University Research and Application Center (591 m altitude and 40° 20’ 01.99”N latitude, 36° 28’ 35.62”E longitude). In the study, sweet cherry (Prunus avium) trees (9 years old) belonging to Regina cultivar grafted on MaxMa 14 rootstock were selected as plant material. The trees were planted with 4 × 4 m space and trained by the Vogel Central Leader system. Irrigation, fertilization, pruning and other cultural processes during the development of trees (weed, disease and pest control, etc.) were regularly conducted.
Experimental Design and Spray Treatments
The experiment was established as randomized complete block design with three replications and two trees per replication. For the study, four different applications were determined as control, gibberellic acid (GA3, ProGibb®, ValentBioScience, USA), calcium chloride (CaCl2, Sigma-Aldrich, Germany) and GA3+CaCl2. In the field studies of the experiment, eight trees with homogeneous product loads were used in each block, so 24 trees in total.
The anticipated harvest date (AHD) (20 June 2017) was determined to be 21 days after the period at the straw-yellow stage of fruit skin color. GA3 solution was sprayed on trees at a concentration of 30 mg L−1 (Ozkaya et al., Citation2006) 3 weeks before the AHD, while CaCl2 solution was sprayed on trees at 0.5% concentration 20 and 10 days before the AHD. Tween 20 (0.1%, Tween 20, Sigma-Aldrich, Germany) was used as the surfactant in all treatments. Prepared solutions were sprayed with a hand priming pump with low pressure in the morning of a windless and rain-free day until the trees were completely wet. All treatments were separated with two buffer trees to minimize the risk of drift problems.
On the AHD, enough fruit was harvested by hand from two trees belonging to each treatment on each block (replication) and immediately (30 min) transferred to the post-harvest physiology laboratory with a refrigerated vehicle [10°C and 90% relative humidity (RH)].
Firstly, homogeneous in size and color [at commercial maturity of color grade 4 according to the color scale developed by CTIFL (Center Technique Interprofessionnel des Fruit et Legumes, Paris, France), in which 1-light pink and 7-dark mahogany], undamaged, healthy and flawless fruits were selected. Then, 1 kg of fruit was taken from each application in each block for the AHD analysis (June 20, 2017), and analyses were performed. In addition, the fruits of each application harvested from each block to determine the quality characteristics during the cold storage period were divided into 18 groups, each containing approximately 1 kg of fruit. Half of them (nine pieces) were placed in the plastic boxes (39 × 29 × 21 cm, Plastas, Turkey) with modified atmosphere packaging [Xtend® (815-CH97/a, StePac, Tefen, Israel)], and the other half were also placed in the plastic boxes without MAP and the fruits were transferred to the cold storage immediately. The fruits were pre-cooled with cold air in the conditions of 24 h, 4.0 ± 0.5°C and 90 ± 5% RH, and the plastic boxes were closed with plastic clips. Finally, the fruits were kept at 0.0 ± 0.5°C and 90 ± 5% RH for 21 days. The measurements and analyses were made at the end of the 7th, 14th and 21st days of the enclosure. Three boxes of fruit were taken for each application during each measurement period. Each box represented a replication.
The Change of O2 and CO2 Gas Composition (%) in MAP
The O2 and CO2 gas composition (%) of MAP during the cold storage was determined by a gas analyzer (Abiss, model legend, France).
Weight loss
Firstly, at the beginning of the enclosure, the weights of the fruit (Wi) were determined by a digital scale with a precision of 0.01 g (Radwag, Poland). Then, on days 7, 14 and 21, the fruits (Wf) were weighed. The weight loss (WL) that occurs in fruit was based on the weight at the beginning of each measurement period and determined as a percentage through the equality given below (EquationEq.1)(Eq.1)
(Eq.1) .
Decay ratio
Before the cold storage, the fruits (about 1 kg of fruit) were counted in each replication, and the total number of fruit (TF) was determined. Then, during each measurement period, the decayed fruits (DF) in each replication were determined. If the development of mycelium on fruit surface occurred, the fruits were considered rotten. Finally, with the following equation (EquationEq.2(Eq.2)
(Eq.2) ), the decay rate (DR, %) was detected (Selcuk and Erkan, Citation2014)
L* and A* Color Characteristics
Color data of sweet cherry fruit were presented according to CIE system (Commission Internationale de l’Eclairage) with L* (lightness) and a* color feature (-a* = greenness and +a* = redness). Color measurement was made via a color meter (Konica Minolta, CR–400, Japan). Measurements were determined at two different points in the equatorial part of the fruit, and 20 fruits were used in each replication (McGuire, Citation1992).
Fruit firmness
Ten fruits from each replication (30 fruits for each treatment) were used for firmness measurements (Ozturk et al., Citation2019b). The measurements were made on two opposite sides of the equatorial part of the fruit through a portable digital durometer (nondestructive device, Agrosta® 100 Field, France) with a flat cylindrical surface and with a diameter of 10 mm. The tip of the durometer was slightly and longitudinally pressed to the outer skin of the fruit, and the Durofel units on the screen were recorded. If the value is close to 100, the fruit is considered very firm, and close to 0 indicates that the fruit is extremely soft.
Soluble Solids Content (SSC), titratable acidity and vitamin C
Twenty fruits taken from each replication were first washed with pure water, and their seeds were separated from the fruit flesh. The fruits were chopped with a stainless steel knife, cut into parts and homogenized by a blender (Model No. Promix HR2653 Philips, Turkey). Then, the homogenate was filtered through a cheesecloth, and the juice was obtained. Soluble solids content (SSC) was measured with a portable digital refractometer (Atago PAL-1, USA) and expressed as a percentage. For titratable acidity measurement, 10 mL juice was taken and 10 mL distilled water was added on. Then, 0.1 N NaOH (sodium hydroxide) was added until the pH of the solution reached 8.2. Based on the amount of NaOH consumed during titration, titratable acidity was determined and expressed as g malic acid 100 mL−1. For vitamin C measurement, 0.5 mL juice was taken, and 5 mL of 0.5% oxalic acid was added to it. The ascorbic acid test strip (Catalog no: 116981, Merck, Germany) was then taken from a collapsible sealed gas-tight tube. Reflectometer (Merck RQflex plus 10) was started. The test strip was plunged into the solution for 2 seconds and then removed from the solution. It was then held for 8 seconds, and reading was done at the end of the 15th second. Results presented as mg 100 mL−1.
Total Phenolics, Total Flavonoids, Total Monomeric Anthocyanin and Antioxidant Activity
During each measurement period, 20 fruits were taken from each replication of each application. The fruits were washed with distilled water, and their stones were removed by hand and sliced with a stainless steel knife. Later, the fruit pulp was crumbled by a blender (Model No. Promix HR2653, Philips, Turkey) and homogenized. About 30 mL of homogenate was taken and placed in a 50 ml falcon tube. The prepared tubes were kept at −20°C until analyses were carried out.
Before beginning the analyses, the frozen samples were dissolved below room temperature (21°C). Pulp and juice were separated from each other by a centrifuge at 12.000 × g at 4°C during 35 minutes. The resulting filtrate was used to determine the content of total phenolics, total flavonoids, total monomeric anthocyanin and antioxidant activity.
Spectrophotometric measurements for bioactive compounds were performed at the UV–Vis spectrophotometer (Shimadzu UV-1601PC, Kyoto, Japan). Total phenolics were measured according to the method of Singleton and Rossi (Citation1965) and were expressed as mg GAE (gallic acid equivalent) 100 g−1 fresh weight (fw). Total flavonoids were measured according to the method of Chang et al. (Citation2002) and were expressed as mg QE (quercetin equivalent) 100 g−1 fw. Total monomeric anthocyanin was determined by using pH (4.5 and 7.0) differential principle according to the method described by Giusti et al. (Citation1999). The obtained results were shown as mg cyanidin 3-glucoside (cy-3-glu) 100 g−1 on a fresh weight basis. The antioxidant activity of sweet cherry fruit was determined according to two different procedures of Trolox Equivalent Antioxidant Capacity (TEAC) (Ozgen et al., Citation2006) and Ferric Ions (Fe+3) Reducing Antioxidant Power (FRAP) (Benzie and Strain, Citation1999), and the results were expressed in µmol Trolox equivalent (TE) 100 g−1 fw.
Statistical analysis
Whether the data were normally distributed was checked by Kolmogorov-Smirnov Test. Homogeneity control of the group/subgroup variances was confirmed by Levene’s test. After the variance analysis of the data was done according to factorial design, Tukey’s multiple-comparison test was used to check whether there were significant differences between treatments. The statistical analyses were performed by using SAS software (SAS 9.1 version, USA).
Results and discussion
The Change of O2 and CO2 Gas Composition (%) in MAP
Data on the change of O2 and CO2 gas composition are presented in . The composition of O2 varied in the range of 15.20–13.43% in control, 14.83–16.11% in CaCl2, 15.97–16.80% in GA3 and 15.46–16.97% in GA3+CaCl2. The CO2 composition varied in the range of 1.40−5.70% in control, 1.13–4.43% in CaCl2, 0.80–4.90% in GA3 and 0.80–6.90% in GA3+CaCl2.
Table 1. The change in MAP gas composition of sweet cherry fruit treated with GA3 and CaCl2 during cold storage.
Weight Loss and Decay Ratio
During the cold storage, it was determined that the weight loss on the fruits of GA3+CaCl2 application stored in MAP was lower compared to both control and other applications. When the fruit without MAP were evaluated, the weight loss of the fruit treated with CaCl2 on the 7th day of the cold storage and GA3 and CaCl2 on day 14th of the cold storage were significantly lower than the control fruit. At the end of the cold storage, it was observed that only, the weight loss of the CaCl2–applied fruit was lower than the control ().
Figure 1. Effects of pre-harvest spray treatments (GA3 and CaCl2) on the weight loss and decay ratio of sweet cherry fruit throughout cold storage. The means indicated with the same letter vertically in periods, and above the bars were not significant according to Tukey’s test at P < .05.
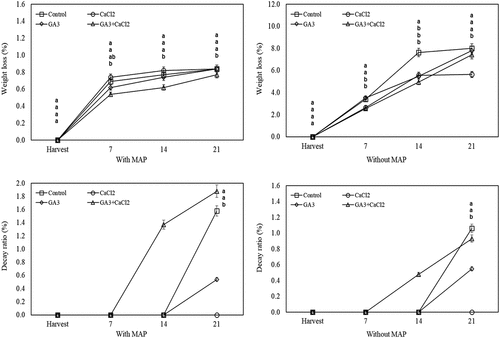
During the cold storage period, the decay was not observed on the fruit of CaCl2 application. However, at the end of the cold storage, in the fruit with MAP; GA3 and CaCl2 applications had a lower decay ratio compared to the control. In fruit without MAP, the fruit treated with GA3 had a lower decay ratio than the control fruit ().
Weight loss during the cold storage of fruit is a significant problem leading to greater economic losses (Sandhya, Citation2010). Sometimes, the weight loss can rise to 25–30%. The weight loss in fruit is related to the evaporation of water by transpiration, depending on the surface–volume ratio of the fruit and the relative humidity in the atmosphere surrounding the fresh product (Kader and Yahia, Citation2011). Technology such as MAP contributes to the extension of the cold storage time and reduction of weight loss during cold storage by reducing gas exchange and water loss in fruit (Valero et al., Citation2014). The positive effect of MAP on weight loss reduction has been reported by many researchers (Erkan and Eski, Citation2012). It has been reported that GA3 and CaCl2 applications in different fruit species have positive effects on delaying weight loss (Sharma and Pratima, Citation2018). The significant role of PGRs such as calcium (Zoffoli et al., Citation2017) and gibberellic acid (Fortes et al., Citation2015) in the formation and structure of the cuticle layer of the fruit may be the reason for the reduction in weight loss in the cold storage.
As in many fruits, in sweet cherry as well, in the cold storage, the decay occurs due to diseases such as Monilinia spp., Botrytis cinerea, Alternaria spp., Rhizopus stolonifer and Mucor piriformis caused by post-harvest damage and excessive moisture, and it causes significant product losses (Cappellini and Ceponis, Citation1977). It is possible to reduce the losses caused by the decay by increasing the fruit firmness with growth regulators such as CaCl2 and GA3 before harvest and by controlling the atmosphere of gas and moisture around the fruit with post-harvest MAP applications (Rodrigez and Zoffoli, Citation2016).
MAP successfully prolongs the post-harvest shelf-life of the fruit by reducing their respiration rate, minimizing metabolic activity, delaying enzymatic browning and retaining visual appearance (Waghmare and Annapure, Citation2013). MAP creates a suitable environment, which is a low level of oxygen and moderate level of carbon dioxide in the package, to maintain the quality characteristics of the packaged product (Mendoza et al., Citation2016). MAP is a widely used technique for maintaining the natural quality of fruit as well as for extending the cold storage time (Horev et al., Citation2012). The positive effect of MAP on atmospheric change during the cold storage maintains fruit firmness and reduces respiratory activity, ethylene production, enzymatic reactions and some physiological changes, thus it helps to maintain quality longer (Gorny, Citation1997). Rodrigez and Zoffoli (Citation2016) reported that the decay rate in the cold storage in “Brigitta” blueberry cultivar was reduced by CaCl2 and MAP applications. CaCl2 is used as a preservative to extend the post-harvest life of fruit and vegetables by increasing fruit firmness (Rico et al., Citation2007). This effect may be the result of forming a cross-linked polymer network by interacting calcium ions and pectic polymers. Kader (Citation2002) has reported that the high calcium content in fruit delays the fruit ripening, increases the fruit firmness and reduces physiological disorders and the fruit decay. Gibberellic acid applications, which thicken the cuticle layer in fruit and increase fruit firmness, are widely used to reduce the risk of product losses in sweet cherry.
Fruit Color Characteristics and Firmness
At harvest, it was determined that the a* value of the fruit treated with CaCl2 and GA3 was significantly lower than the control, but at the end of the cold storage, these fruits had higher a* value. At the harvest, the CaCl2-applied fruit, and on the 7th day of the cold storage, the fruit treated with CaCl2 and GA3 had higher L* than the control fruit. On the 7th day of the cold storage, the L* value of the fruit of CaCl2 application with MAP was higher than both the control and the other applications. However, on the 14th and 21st days of the cold storage, it was observed that in the fruit without MAP, the L* value of the fruit treated with the GA3+CaCl2 was higher than the control fruit ().
Figure 2. Effects of pre-harvest spray treatments (GA3 and CaCl2) on the L*, a* and firmness of sweet cherry fruit throughout cold storage. The means indicated with the same letter vertically in periods, and above the bars were not significant according to Tukey’s test at P < .05. * The scale ranges from 0 to 100 for very soft to very firm surfaces.
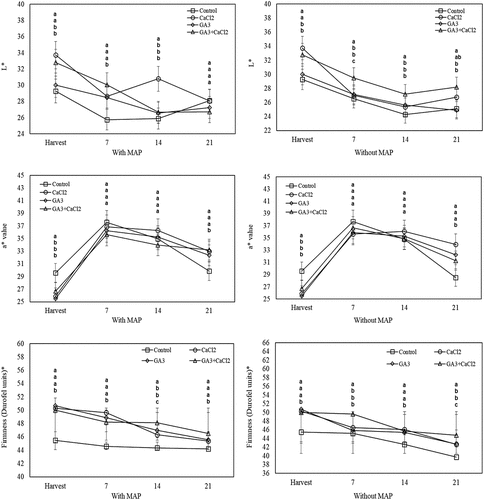
At harvest, the fruit firmness of the CaCl2 and GA3 applied fruit was significantly higher than the control. In the last two measurements of cold storage, the fruit softening was slower in CaCl2 and GA3 treated fruit both with and without MAP. At the end of the cold storage, in the fruit without MAP, the firmness loss of the CaCl2 and GA3-applied fruit was higher compared to the fruit of the GA3+CaCl2 application ().
Fruit color, which is a significant criterion in terms of consumer preferences, varies depending on the maturity of the fruit. Patino et al. (Citation2018) reported that there was an increase in red index a* during the cold storage, but the increase was lower with MAP application. Chemical applications such as calcium extend shelf life and reduce discoloration in apples (Rocha et al., Citation1998). Again, Dong et al. (Citation2019) have determined that GA3 application delays fruit color change during the cold storage in sweet cherry.
Fruit firmness, an important quality attribute, is directly related to enhancing the storability potential and to induce greater resistance to mechanical damage and fruit decay (Esti et al., Citation2002). The main target is the maintenance of fruit firmness during the cold storage to extend the storage life. It is possible to obtain more resistant fruit by using growth regulators such as pre-harvest calcium (Rico et al., Citation2007) and GA3 (Einhorn et al., Citation2013) and to maintain the post-harvest fruit quality by using post-harvest MAP packaging techniques. MAP application contributes to the maintenance of fruit firmness by reducing respiratory activity, ethylene production, enzymatic reactions and some physiological changes during the cold storage and thus helps to maintain quality longer (Gorny, Citation1997).
CaCl2, which increased the fruit firmness, is used to prolong the post-harvest life of fruits and vegetables (Rico et al., Citation2007). High calcium content in the fruit also delays ripening and increases fruit firmness (Kader, Citation2002). In addition, the application of calcium pre- or post-harvest is considered by some to be beneficial (Wang et al., Citation2014; Zoffoli et al., Citation2017). The effect of pre-harvest CaCl2 sprays on sweet cherry fruit firmness is inconsistent (Winkler and Knoche, Citation2019). This effect can be explained by the interaction of calcium ions and pectic polymers and delaying the onset of climacterial elevation in fruit (Ben-Arie et al., Citation1995).
Growth regulators are widely used to increase fruit size and quality (Fortes et al., Citation2015), contributing to the preservation of the storage quality due to its effect on the cell wall (Eccher and Hajnajari, Citation2006). Studies (Choi et al., Citation2002; Clayton et al., Citation2006; Correia et al., Citation2019) have shown that GA3 applications increase fruit size and fruit firmness in sweet cherry. Again, Cline and Trought (Citation2007), in sweet cherry, GA3 treatments increased fruit firmness by 5% compared with untreated control fruit.
SSC, Titratable Acidity and Vitamin C
At the harvest, the highest SSC, titratable acidity and vitamin C were obtained from the fruit belonging to the GA3 application, whereas the lowest SSC, titratable acidity and vitamin C were obtained from GA3+CaCl2 applied fruit. The highest titratable acidity and vitamin C were recorded in the fruit of the CaCl2 application. At the end of the cold storage, in the applications both with and without MAP, the fruit of the CaCl2 application had significantly lower SSC and higher titratable acidity compared to the control. The fruits treated with GA3 and stored without MAP were found to have significantly lower acidity than the control. When the fruits stored in MAP were evaluated, it was determined that the vitamin C content of the fruit treated with GA3 and CaCl2 was significantly lower compared the control. However, in the fruit stored without MAP, it was observed that the vitamin C content in the fruit of only the GA3+CaCl2 application was significantly lower than the control ().
Figure 3. Effects of pre-harvest spray treatments (GA3 and CaCl2) on the SSC, titratable acidity and vitamin C of sweet cherry fruit throughout cold storage. The means indicated with the same letter vertically in periods, and above the bars were not significant according to Tukey’s test at P < .05.
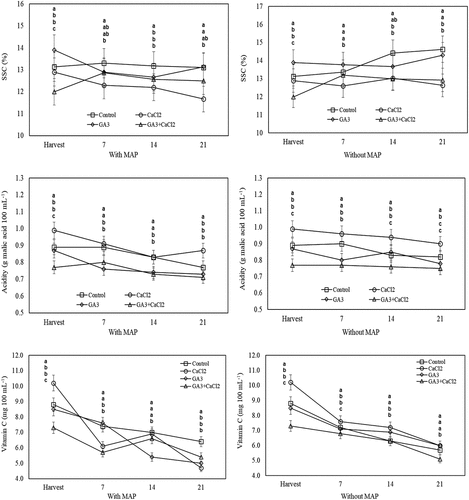
SSC is directly related to the taste of the fruits. The majority of SSC consists of sugars. SCC increases, and titratable acidity and vitamin C decrease due to maturation progresses during the storage (Abd El-Gawad et al., Citation2019). Similarly, it was reported that during the cold storage in sweet cherry, SSC rate increased and titratable acidity (Gonçalves et al., Citation2004) and vitamin C content (Tian et al., Citation2004) decreased. The effect of MAP application at the cold storage on the chemical composition can be explained by the change in physiological phenomena such as ethylene production, respiration and transpiration due to MAP application (Domínguez et al., Citation2016). It has been reported that MAP applications have caused the decreasing of SSC ratio by slowing down the ripening during the cold storage in kiwifruit (Zhang et al., Citation2003) and sweet cherry (Diaz-Mula et al., Citation2012). However, Aglar et al. (Citation2017) determined that MAP treatment does not cause much change in the SSC value of sweet cherry. Gonçalves et al. (Citation2004) reported that MAP treatment increased SSC rate and decreased titratable acidity rate of sweet cherry fruits throughout the storage. Mohammadi and Hanafi (Citation2014) reported significantly delayed losses in vitamin C content of strawberry fruits treated with MAP treatments.
Beigi et al. (Citation2019) reported that CaCl2 treatment had a positive impact on the fruit chemical composition, and simultaneous application of CaCl2 and GA3 resulted in the maximum content of pH and SSC and the minimum acidity and ascorbic acid. In this study, ripening-retarding effect of GA3 was clearly observed in SSC. In a study by Alrashdi et al. (Citation2017), which was carried out on table grapes, it was determined that SSC content was not affected by the GA3 treatments. In addition, Winkler and Knoche (Citation2019) reported that pre-harvest Ca application reduced losses in soluble solids during storage. However, Correia et al. (Citation2019) determined that CaCl2 application did not have an effect in soluble solids.
Total Phenolics, Total Flavonoids, Total Monomeric Anthocyanin and Antioxidant Activity
At the harvest, the total phenolics content of GA3-applied fruit was significantly lower than the control. However, the total flavonoids content of the GA3 and CaCl2 applications and the total anthocyanin of the fruit treated with CaCl2 were significantly higher than the control. According to the FRAP assay, the antioxidant activity of only the CaCl2 application was significantly higher than the control. At the end of the cold storage, the total phenolics of the fruit treated with GA3 and CaCl2 and stored in MAP were significantly higher compared to the control. However, during the cold storage, the total phenolics and total anthocyanin of the GA3-applied fruit and kept without MAP were significantly lower than the control. In the last two measurements of the cold storage, the total flavonoids of CaCl2-applied fruit and stored in MAP were significantly higher than the control. During the cold storage, according to both FRAP and DPPH tests, the antioxidant activity of the fruit treated with GA3+CaCl2-treated and stored in MAP was significantly lower than the control ().
Figure 4. Effects of pre-harvest spray treatments (GA3 and CaCl2) on the total phenolics, total flavonoids and total anthocyanin of sweet cherry fruit throughout cold storage. The means indicated with the same letter vertically in periods, and above the bars were not significant according to Tukey’s test at P < .05.
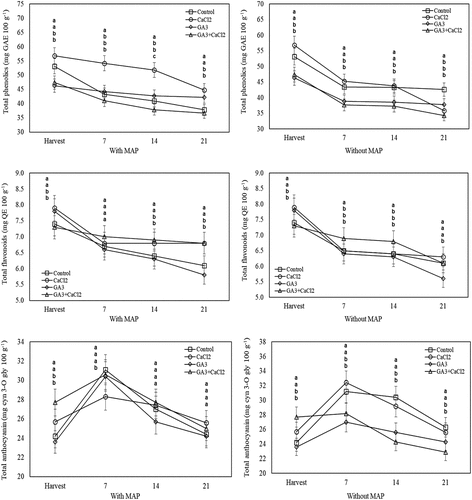
Figure 5. Effects of pre-harvest spray treatments (GA3 and CaCl2) on the antioxidant activity (according to FRAP and TEAC assays) of sweet cherry fruit throughout cold storage. The means indicated with the same letter vertically in periods, and above the bars were not significant according to Tukey’s test at P < .05.
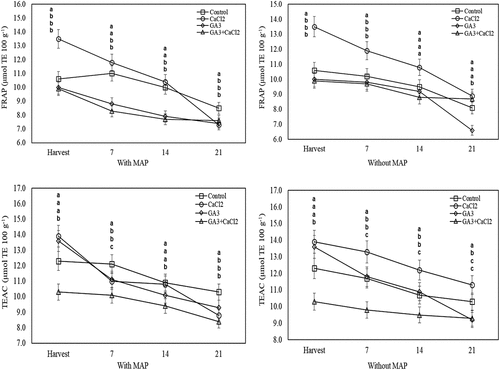
Sweet cherry is a rich source of dietary phenolics with antioxidant properties that are associated with a wide range of health benefits (Mulabagal et al., Citation2009). Anthocyanins and flavonoids are important phenolic compounds in sweet cherry (Aghdama et al., Citation2013). The composition and concentration of these compounds may vary depending on genetic and environmental factors and fruit ripening stage (Serra et al., Citation2011). In sweet cherry, there is a correlation between fruit ripening level and bioactive content of fruit, and phenolic substances, anthocyanins and antioxidants have the highest value at optimal maturity level (Mahmood et al., Citation2013).
Aglar et al. (Citation2017) determined that an increase in bioactive compound content from the beginning till the end of the cold storage occurred. Ratanachinakorn et al. (Citation1997) and Maul et al. (Citation2000) determined that MAP treatment can delay aroma deterioration and biochemical changes in the post-harvest period in sweet cherry. Artes-Hernandez et al. (Citation2006) determined that the MAP treatment delayed carotenoid and anthocyanin formation at cold storage. Guan and Dou (Citation2010) have reported that MAP-treated plum fruits have lower antioxidant capacity. Again, Aglar et al. (Citation2017) reported that MAP treatment caused a difference in the bioactive compound content during cold storage. The reason for this might be due to the effect of MAP treatments on gas composition around the fruit.
Michailidis et al. (Citation2017) have reported that CaCl2 sprays increased cherries phenol content and antioxidant activity at harvest and post-harvest periods and decreased in post-harvest respiration rate, ascorbic acid and flavonoid loss. Wang et al. (Citation2014) reported that CaCl2 applications (0.2%, 0.5%) increased total anthocyanin, total flavonoids, total phenolics and total antioxidant capacity after 4 weeks of the cold storage. Aghdama et al. (Citation2013) have shown that post-harvest CaCl2 application increases the antioxidant capacity by effectively preserving higher total flavonoids, total phenolics and anthocyanin content of the cranberry fruit.
Biosynthesis of phenolics such as anthocyanins and flavonoids in plants is carried out via the shikimate-phenylpropanoid-flavonoid pathways, in which phenylalanine ammonia-lyase (PAL) serves as a key enzyme (Kays and Paull, Citation2004). It has been postulated that an enhancement of TP and antioxidant activity in fruit by CaCl2 treatment is due to stimulating the phenylpropanoid pathway by increasing the PAL activity (Aghdama et al., Citation2013). Moreover, these results can be explained with the effect of the calcium on several key physiological processes connected to ripening-related phenomena, including those associated with the structure and functionality of cell walls and membranes, or with the activity of particular enzymes (Lara, Citation2013). In the study, it was determined that GA3-treated fruit had a relatively lower bioactive compound content compared to fruit of calcium and control applications both during harvest and cold storage. The effect of GA3 application had also been effected on the values of CaCl2+GA3 combination application. In accordance with the study results, Diaz-Mula et al. (Citation2009) and Ozkan et al. (Citation2016) reported that GA3 treatments significantly decreased total phenolics, antioxidant capacity and total anthocyanin content of sweet cherry.
Conclusion
At the end of the cold storage, with MAP; the weight loss was lower on the fruit treated with GA3+CaCl2 and on the CaCl2 applied fruit without MAP. During the cold storage, the decay was delayed by CaCl2 application. At the end of the cold storage, the fruit treated with GA3 and CaCl2 had higher a* and firmness values. The SSC in the fruit of the CaCl2 application was lower than the control application. However, significantly higher acidity was determined from CaCl2 application compared to other applications. In fruit stored in MAP, vitamin C content and antioxidant activity of fruit treated with GA3 and CaCl2 were significantly lower. But total phenolics were higher. Also, the total flavonoids of the CaCl2-applied fruit were higher compared to the control. The anthocyanin content of the fruit treated with GA3 and stored without MAP was lower compared to the control.
As a result, it was observed that GA3 treatment retarded fruit ripening and maintained firmness which is a significant quality parameter for sweet cherry. It can be said that pre-harvest GA3 and CaCl2 applications have increased the fruit quality of sweet cherry and have a significant effect on extending the post-harvest life of sweet cherry and pre-harvest GA3 and CaCl2 and post-harvest MAP applications are effective and usable applications to reduce storage losses and maintain fruit quality during the cold storage.
Authors’ Contributions
Burhan Ozturk, Erdal Aglar, Sefa Gun and Orhan Karakaya. Planning, design, analysis of fruit and data analysis of experiment and writing of the manuscript.
Compliance With Ethical Standards
The authors declare that they have no conflict of interest
Acknowledgments
This study was supported by the Scientific Research Unit (Project number AR-1658) of Ordu University.
Disclosure Statement
No potential conflict of interest was reported by the author(s).
References
- Abd El-Gawad, M.G., Z.A. Zaki, and Z.A. Ekbal. 2019. Effect of some postharvest treatments on quality of “Alphonse” Mango fruits during cold storage. Middle East J 8,1067-1079.
- Aghdama, M.S., A.Y. Dokhanieh, H. Hassanpour, and J.R. Fard. 2013. Enhancement of antioxidant capacity of cornelian cherry (Cornus mas) fruit by postharvest calcium treatment. Sci. Hortic. 161:160–164. doi: 10.1016/j.scienta.2013.07.006.
- Aglar, E., B. Ozturk, S.K. Guler, O. Karakaya, S. Uzun, and O. Saracoglu. 2017. Effect of modified atmosphere packaging and ‘Parka’ treatments on fruit quality characteristics of sweet cherry fruits (Prunus avium L. ‘0900 Ziraat’) during cold storage and shelf life. Sci. Hortic. 222:162–168. doi: 10.1016/j.scienta.2017.05.024.
- Aglar, E., O. Saracoglu, O. Karakaya, B. Ozturk, and S. Gun. 2019. The relationship between fruit color and fruit quality of sweet cherry (Prunus avium L. cv.‘0900 Ziraat’). Turk J. Food Agric. Sci. 1(1):1–5.
- Alrashdi, A.M.A., A.D. Al-Qurashi, M.A. Awad, S.A. Mohamed, and A.A. Al-rashdi. 2017. Quality, antioxidant compounds, antioxidant capacity and enzymes activity of ‘El-Bayadi’ table grapes at harvest as affected by preharvest salicylic acid and gibberellic acid spray. Sci. Hortic. 220:243–249. doi: 10.1016/j.scienta.2017.04.005.
- Artes-Hernandez, F., F.A. Tomas-Barberan, and F. Artes. 2006. Modified atmosphere packaging preserves quality of SO2-free ‘Superior seedless’ table grapes. Postharvest Biol. Technol. 39(2):146–154. doi: 10.1016/j.postharvbio.2005.10.006.
- Beigi, M.H., A. Zarei, M. Rostaminia, and J.E. Moghadam. 2019. Positive effects of foliar application of Ca, B and GA3 on the qualitative and quantitative traits of pomegranate (Punica granatum L.) cv. ‘Malase-Torshe-Saveh. Sci. Hortic. 254:40–47. doi: 10.1016/j.scienta.2019.04.081.
- Ben-Arie, R., I. Mignani, L.C. Greve, M. Huysamer, and J.M. Labavitch. 1995. Regulation of the ripening of tomato pericarp discs by GA3 and divalent cations. Physiol. Plant 93(1):99–107. doi: 10.1034/j.1399-3054.1995.930115.x.
- Benzie, I.F., and J.J. Strain. 1999. Ferric reducing/antioxidant power assay: Direct measure of total antioxidant activity of biological fluids and modified version for simultaneous measurement of total antioxidant power and ascorbic acid concentration. Meth. Enzymol. 299:15–27.
- Brown, G., S. Wilson, W. Boucher, B. Graham, and B. McGlasson. 1995. Effects of copper-calcium sprays on fruit cracking in sweet cherry (Prunus avium). Sci. Hortic 62(1–2):75–80. doi: 10.1016/0304-4238(94)00746-3.
- Cappellini, R.A., and M.J. Ceponis. 1977. Vulnerability of stem-end scars of blueberry fruits to postharvest decays. Phytopathol 67(1):118–119. doi: 10.1094/Phyto-67-118.
- Chang, C.C., M.H. Yang, H.M. Wen, and J.C. Chern. 2002. Estimation of total flavonoid content in propolis by two complementary colorimetric methods. J. Food Drug Anal. 10(3):178–182.
- Choi, C., P.A. Wiersma, P. Toivonen, and F. Kappel. 2002. Fruit growth, firmness and cell wall hydrolytic enzyme activity during development of sweet cherry fruit treated with gibberellic acid (GA3). J. Hortic. Sci. Biotech. 77(5):615–621. doi: 10.1080/14620316.2002.11511547.
- Clayton, M., W.V. Biasi, I.T. Agar, S.M. Southwick, and E.J. Mitcham. 2006. Sensory quality of bing’sweet cherries following preharvest treatment with hydrogen cyanamide, calcium ammonium nitrate, or gibberellic acid. Hort Science 41(3):745–748. doi: 10.21273/HORTSCI.41.3.745.
- Cline, J.A., and M. Trought. 2007. Effect of gibberellic acid on fruit cracking and quality of Bing and Sam sweet cherries. Can. J. Plant Sci. 87(3):545–550. doi: 10.4141/P06-132.
- Correia, S., F. Queirós, C. Ribeiro, A. Vilela, A. Aires, A.I. Barrosa, R. Schouten, A.P. Ana Silva, and B. Gonçalves. 2019. Effects of calcium and growth regulators on sweet cherry (Prunus avium L.) quality and sensory attributes at harvest. Sci. Hortic. 248:231–240. doi: 10.1016/j.scienta.2019.01.024.
- De Freitas, S.T., and E.J. Mitcham. 2012. Factors involved in fruit calcium deficiency disorders. Hortic. Rev. 40:107–146.
- Diaz-Mula, H.M., S. Castillo, D. Martinez-Romero, D. Valero, P.J. Zapata, F. Guillen, and M. Serrano. 2009. Sensory, nutritive and functional properties of sweet cherryas affected by cultivar and ripening stage. Food Sci. Technol. Int. 15(6):535–543. doi: 10.1177/1082013209351868.
- Diaz-Mula, F.H.M., M. Serrano, and D. Valero. 2012. Alginate coatings preserve fruit quality and bioactive compounds during storage of sweet cherry. Food Bioprocess Technol. 5(8):2990–2997. doi: 10.1007/s11947-011-0599-2.
- Domínguez, I., M.T. Lafuente, P. Hernández-Muñoz, and R. Gavara. 2016. Influence of modified atmosphere and ethylene levels on quality attributes of fresh tomatoes (Lycopersicon esculentum Mill.). Food Chem. 209:211–219. doi: 10.1016/j.foodchem.2016.04.049.
- Dong, Y., H. Zhi, and Y. Wang. 2019. Cooperative effects of pre-harvest calcium and gibberellic acid on tissue calcium content, quality attributes, and in relation to postharvest disorders of late-maturing sweet cherry. Sci. Hortic. 246:123–128. doi: 10.1016/j.scienta.2018.10.067.
- Eccher, T., and H. Hajnajari. 2006. Fluctuations of endogenous gibberellin A4 and A7 content in apple fruits with different sensitivity to russet. Acta Hortic. 727(727):537–544. doi: 10.17660/ActaHortic.2006.727.66.
- Einhorn, T.C., Y. Wang, and J. Turner. 2013. Sweet cherry fruit firmness and postharvest quality of late-maturing cultivars are improved with low-rate, single applications of gibberellic acid. Hort Science 48(8):1010–1017. doi: 10.21273/HORTSCI.48.8.1010.
- Erkan, M., and H. Eski. 2012. Combined treatment of modifi ed atmosphere packaging and 1-methylcyclopropene improves postharvest quality of Japanese plums. Turk. J. Agric. For. 36:563–575.
- Esti, M., L. Cinquanta, F. Sinesio, E. Moneta, and M. Di Matteo. 2002. Physicochemical and sensory fruit characteristics of two sweet cherry cultivars after cool storage. Food Chem. 76(4):399–405. doi: 10.1016/S0308-8146(01)00231-X.
- Fortes, A.M., R.T. Teixeira, and P. Agudelo-Romero. 2015. Complex interplay of hormonal signals during grape berry ripening. Molecules 20(5):9326–9343. doi: 10.3390/molecules20059326.
- Giusti, M.M., L.E. Rodríguez-Saona, D. Griffin, and R.E. Wrolstad. 1999. Electrospray and tandem mass spectroscopy as tools for anthocyanin characterization. J. Agric. Food Chem. 47(11):4657–4664.
- Gonçalves, B., A.K. Landbo, D. Knudsen, A.P. Silva, J. Moutinho-Pereira, and E. Rosa. 2004. Effect of ripeness and postharvest storage on the phenolic profiles of cherries (Prunus avium L.), p. . J. Agric. Food Chem. 52, 523-530.
- Gorny, J.R. 1997. A summary of CA and MA requirements and recommendations for the storage of fresh-cut (minimally processed) fruits and vegetables. Acta Hortic. 600:609–614.
- Guan, J.F., and S.J. Dou. 2010. The effect of MAP on quality and browning of cold stored plum fruits. J. Food Agric. Environ. 8:113–116.
- Horev, B., S. Sela, Y. Vinokur, E. Gorbatsevich, R. Pinto, and V. Rodov. 2012. The effects of active and passive modified atmosphere packaging on the survival of Salmonella enterica serotype Typhimurium on washed romaine lettuce leaves. Food Res. Int. 45(2):1129–1132. doi: 10.1016/j.foodres.2011.05.037.
- Kader, A.A. 2002. Quality parameters of fresh-cut fruit and vegetable products. In: O. Lamikanra (ed.). Fresh-cut fruits and vegetables: science, technology, and market. CRC Press , Roca Baton, FL. 1-50.
- Kader, A.A., and E.M. Yahia. 2011. Postharvest biology of tropical and subtropical fruits, p. 79–111. In: E.M. Yahia ed. Postharvest biology and technology of tropical and subtropical fruits. Vol. 1. Abington: Woodhead Publishing.
- Kays, S.J., and R.E. Paull. 2004. Movement and exchange of gases, solvents and solutes within harvested products and their exchange between the product and its external environment. Postharvest Biology. Exon Press, Athens, GA. 415–458.
- Lara, I. 2013. Preharvest sprays and their effects on the postharvest quality of fruit. Stewart Postharvest Rev. 9:3–12.
- Mahmood, Z., A. Ishtiag, M.U.Q. Saeed, and M.A. Sheikh. 2013. Investigation of physico-chemical composition and antimicrobial activity of essential oil extracted from lignin-containing Cupressus sempervirens. Bio Resources 8(2):1625–1633. doi: 10.15376/biores.8.2.1625-1633.
- Maul, F., S.A. Sargent, C.A. Sims, E.A. Baldwin, M.O. Balaban, and D.J. Huber. 2000. Tomato flavor and aroma quality as affected by storage temperature. J. Food Sci. 65(7):1228–1237. doi: 10.1111/j.1365-2621.2000.tb10270.x.
- McGuire, R.G. 1992. Reporting of objective colour measurement. Hort Science 27(12):1254–1255. doi: 10.21273/HORTSCI.27.12.1254.
- Mendoza, R., D.A. Castellanos, J.C. García, J.C. Vargas, and A.O. Herrera. 2016. Ethylene production, respiration and gas exchange modelling in modified atmosphere packaging for banana fruits. Inter. J. Food Sci. Technol. 51(3):777–788. doi: 10.1111/ijfs.13037.
- Michailidis, M., E. Karagiannis, G. Tanou, K. Karamanoli, A. Lazaridou, T. Matsi, and A. Molassiotis. 2017. Metabolomic and physico-chemical approach unravel dynamic regulation of calcium in sweet cherry fruit physiology. Plant Physiol. Biochem. 116:68–79. doi: 10.1016/j.plaphy.2017.05.005.
- Mohammadi, H., and Q. Hanafi. 2014. Effect of different atmospheres on quality changes of Kurdistan strawberry. J. Food Chem. Nutr. 2:61–69.
- Mulabagal, V., G.A. Lang, D.L. Dewitt, S.S. Dalavoy, and M.G. Nair. 2009. Anthocyanin content, lipid peroxidation and cyclooxygenase enzyme inhibitory activities of sweet and sour cherries. J. Agric. Food Chem. 57(4):1239–1246. doi: 10.1021/jf8032039.
- Ozgen, M., R.N. Reese, A.Z. Tulio, A.R. Miller, and J.C. Scheerens. 2006. Modified 2,2-azino-bis-3-ethylbenzothiazoline-6-sulfonic acid (ABTS) method to measure antioxidant capacity of selected small fruits and comparison to ferric reducing antioxidant power (FRAP) and 2,2-diphenyl-1-picrylhydrazyl (DPPH) methods. J. Agric. Food Chem. 54(4):1151–1157. doi: 10.1021/jf051960d.
- Ozkan, Y., M. Ucar, K. Yildiz, and B. Ozturk. 2016. Pre-harvest gibberellic acid (GA3) treatments play an important role on bioactive compounds and fruit quality of sweet cherry cultivars. Sci. Hortic. 211:358–362. doi: 10.1016/j.scienta.2016.09.019.
- Ozkaya, O., O. Dundar, and A. Kuden. 2006. Effect of preharvest gibberellic acid treatments on postharvest quality of sweet cherry. J. Food Agric. Environ. 4(1):189–191.
- Ozturk, B., E. Aglar, O. Karakaya, O. Saracoglu, and S. Gun. 2019a. Effects of preharvest GA3, CaCl2 and modified atmosphere packaging treatments on specific phenolic compounds of sweet cherry. Turk J. Food Agric. Sci. 1(2):44–56.
- Ozturk, A., K. Yildiz, B. Ozturk, O. Karakaya, S. Gun, S. Uzun, and M. Gundogdu. 2019b. Maintaining postharvest quality of medlar (Mespilus germanica) fruit using modified atmosphere packaging and methyl jasmonate. LWT, Food Sci. Technol. 111:117–124. doi: 10.1016/j.lwt.2019.05.033.
- Patino, L.S., D.A. Castellanos, and A.O. Herrera. 2018. Influence of 1-MCP and modified atmosphere packaging in the quality and preservation of fresh basil. Postharvest Biol. Technol. 136:57–65.
- Petracek, P.D., D.W. Joles, A. Shirazi, and A.C. Cameron. 2002. Modified atmosphere packaging of sweet cherry (Prunus avium L. cv ‘Sams’) fruit: Metabolic responses to oxygen, carbon dioxide, and temperature. Postharvest Biol. Technol. 24(3):259–270.
- Pharis, R.P., and R.W. King. 1985. Gibberellins and reproductive development in seed plants. Ann. Rev. Plant Physiol. 36(1):517–568. doi: 10.1146/annurev.pp.36.060185.002505.
- Ratanachinakorn, B., A. Klieber, and D.H. Simons. 1997. Effect of short-term controlled atmospheres and maturity on ripening and eating quality of tomatoes. Postharvest Biol. Technol. 11(3):149–154. doi: 10.1016/S0925-5214(97)00021-5.
- Rico, D., A.B. Martin-Diana, J.M. Barat, and C. Barry-Ryan. 2007. Extending and measuring the quality of fresh-cut fruit and vegetables: A review. Trends Food Sci. Tech. 18(7):373–386. doi: 10.1016/j.tifs.2007.03.011.
- Rocha, A.M.C.N., C.M. Brochado, and A.M.M.B. Morais. 1998. Influence of chemical treatment on quality of cut apple (cv. Journal of Food Quality 21(1):13–28. doi: 10.1111/j.1745-4557.1998.tb00500.x.
- Rodriguez, J., and J.P. Zoffoli. 2016. Effect of sulfur dioxide and modified atmosphere packaging on blueberry postharvest quality. Postharvest Biol. Technol. 117:230–238. doi: 10.1016/j.postharvbio.2016.03.008.
- Sandhya, K.V.K. 2010. Modified atmosphere packaging of fresh produce: Current status and future needs. LWT - Food Sci. Technol. 43(3):381–392. doi: 10.1016/j.lwt.2009.05.018.
- Selcuk, N., and M. Erkan. 2014. Changes in antioxidant activity and postharvest quality of sweet pomegranates cv. Hicrannar under modified atmosphere packaging. Postharvest Biol. Technol. 92:29–36. doi: 10.1016/j.postharvbio.2014.01.007.
- Serra, A.T., R.O. Duarte, M.R. Bronze, and C.M.M. Duarte. 2011. Identification of bioactive response in traditional cherries from Portugal. Food Chem. 125(2):318–325. doi: 10.1016/j.foodchem.2010.07.088.
- Serrano, M., D. Martinez-Romero, S. Castillo, F. Guillén, and D. Valero. 2005. The use of natural antifungal compounds improves the beneficial effect of MAP in sweet cherry storage. Innov. Food Sci. Emerg Technol. 6(1):115–123. doi: 10.1016/j.ifset.2004.09.001.
- Sharma, S.K., and P. Pratima. 2018. Effect of pre-harvest gibberellic acid and calcium application on post-harvest behaviour of subtropical peaches. Current Agri. Res. J. 6(1):78–84. doi: 10.12944/CARJ.6.1.10.
- Singleton, V.L., and J.A. Rossi. 1965. Colorimetry of total phenolics with phosphomolybdic-phosphotungstic acid reagents. Am. J. Enol. Viticult. 16(3):144–158.
- Tian, S.P., A.L. Jiang, Y. Xu, and Y.S. Wang. 2004. Responses of physiology and quality of sweet cherry fruit to different atmosphere in storage. Food Chem. 87(1):43–49. doi: 10.1016/j.foodchem.2003.10.014.
- Valero, D., S.H. Mirdehghan, M. Sayyari, and M. Serrano. 2014. Vapor treatments, chilling, storage, and antioxidants in pomegranates, p. 189–196. In: V.R. Preedy (ed.). Processing and Impact on Active Components in Food. Academic Press, London.
- Waghmare, R.B., and U.S. Annapure. 2013. Combined effect of chemical treatment and/or modified atmosphere packaging (MAP) on quality of fresh-cut papaya. Postharvest Biol. Technol. 85:147–153.
- Wang, Y., X. Xie, and L.E. Long. 2014. The effect of postharvest calcium application in hydrocooling water on tissue calcium content, biochemical changes, and quality attributes of sweet cherry fruit. Food Chem. 160:22–30. doi: 10.1016/j.foodchem.2014.03.073.
- Wani, A.A., P. Singh, K. Gul, M.H. Wani, and H.C. Langowski. 2014. Sweet cherry (Prunus avium): Critical factors affecting the composition and shelf life. Food Packag. Shelf Life 1(1):86–99. doi: 10.1016/j.fpsl.2014.01.005.
- Wermund, U., A. Holland, and S. Reardon. 2001. Cracking susceptibility of sweet cherries in the United Kingdom in relation to calcium application and cover systems. Acta Hortic. 667:475–482.
- Winkler, A., and M. Knoche. 2019. Calcium and the physiology of sweet cherries: A review. Sci. Hortic. 245:107–115. doi: 10.1016/j.scienta.2018.10.012.
- Zhang, Y., K. Chen, S. Zhang, and I. Ferguson. 2003. The role of salicylic acid in postharvest ripening of kiwifruit. Postharvest Biol. Technol. 28(1):67–74. doi: 10.1016/S0925-5214(02)00172-2.
- Zoffoli, J.P., P. Toivonen, and Y. Wang.2017. Postharvest biologoy and handling for fresh market. p. 460–484. In: eds. J. Quero-García, A. Iezzoni, J. Puławska, and G. Lang. Cherries: botany, production and uses. Vol. Chapter 19, CABI, Oxfordshire, Wallingford, UK.