Abstract
Apoptosis is a well-defined cellular process in which a cell dies, characterized by cell shrinkage and DNA fragmentation. In parasites like Leishmania, the process of apoptosis-like cell death has been described. Moreover upon infection, the apoptotic-like population is essential for disease development, in part by silencing host phagocytes. Nevertheless, the exact mechanism of how apoptosis in unicellular organisms may support infectivity remains unclear. Therefore we investigated the fate of apoptotic-like Leishmania parasites in human host macrophages. Our data showed—in contrast to viable parasites—that apoptotic-like parasites enter an LC3+, autophagy-like compartment. The compartment was found to consist of a single lipid bilayer, typical for LC3-associated phagocytosis (LAP). As LAP can provoke anti-inflammatory responses and autophagy modulates antigen presentation, we analyzed how the presence of apoptotic-like parasites affected the adaptive immune response. Macrophages infected with viable Leishmania induced proliferation of CD4+ T-cells, leading to a reduced intracellular parasite survival. Remarkably, the presence of apoptotic-like parasites in the inoculum significantly reduced T-cell proliferation. Chemical induction of autophagy in human monocyte-derived macrophage (hMDM), infected with viable parasites only, had an even stronger proliferation-reducing effect, indicating that host cell autophagy and not parasite viability limits the T-cell response and enhances parasite survival. Concluding, our data suggest that apoptotic-like Leishmania hijack the host cells´ autophagy machinery to reduce T-cell proliferation. Furthermore, the overall population survival is guaranteed, explaining the benefit of apoptosis-like cell death in a single-celled parasite and defining the host autophagy pathway as a potential therapeutic target in treating Leishmaniasis.
Abbreviations:
- ANXA5, annexin V
- CFSE, carboxyfluorescein succinimidyl ester
- CM, complete medium
- hMDM, human monocyte derived macrophage
- IF, immunofluorescence
- IL, interleukin
- LAP, LC3-associated phagocytosis
- MAP1LC3/LC3, microtubule-associated protein 1 light chain 3
- Lm, Leishmania
- log.ph, logarithmic phase
- MACS, magnetic-associated cell sorting
- MFI, mean fluorescence intensity
- MHC, major histocompatibility complex
- MOI, multiplicity of infection
- PBMCs, peripheral blood mononuclear cells
- PS, phosphatidylserine
- stat.ph, stationary phase
- TGFB, transforming growth factor
- β; TT, tetanus toxoid
Introduction
The protozoan parasites of the genus Leishmania are the causative agent of Leishmaniasis, leading to upwards of 30,000 deaths yearly. The infective inoculum of Leishmania consists of both viable and apoptotic-like parasites, the latter also being required for disease development.Citation1 The exact mechanism for how apoptosis in unicellular organisms may support infectivity remains elusive. Apoptosis-like programmed cell death has been described in various unicellular protists, including the protozoan parasites Plasmodium and Trypanosoma.Citation2 It has been speculated how apoptosis could serve single-celled organisms. As most protozoan parasites are vector transmitted, it may be necessary to restrict the population size in the vector, as shown for Trypanosoma brucei and Plasmodium.Citation3,4 From an overall population perspective, the induction of apoptosis could also be employed by the parasites to evade early immune responses. Evidence supporting the idea that apoptosis contributes to survival of the overall population was given by the fact that phagocytosis of apoptotic-like parasites induces production of anti-inflammatory mediators, likely to silence phagocytes enabling the intracellular survival of viable parasites.Citation5 In agreement, apoptotic cells exacerbate parasite growth in Trypanosoma cruzi infection and phosphatidylserine exposure by Toxoplasma gondii is fundamental for granting intracellular survival, in macrophages.Citation6,7 Macrophages also serve as host cell for Leishmania parasites and act as antigen-presenting cells, in which phagosomal and autophagosomal maturation are involved in major histocompatibility complex (MHC) loading. In addition to the well-known endogenous MHC loading pathway, recent efforts have shed light on the involvement of autophagy-related proteins in antigen presentation. A novel processing compartment is formed, having a profound effect on protein digestion, contributing to a more efficient antigen processing and MHC loading, hereby orchestrating the immune system.Citation8-11
Autophagy is a “double-edged sword” in the field of immunity. The autophagy-related proteins, like the mammalian orthologs and paralogs of yeast Atg8 (herein LC3), facilitate the elimination of invading pathogens.Citation12-14 Nevertheless, the autophagy machinery is also involved in suppressing oncogenesis and plays a role during homeostasis, by clearing apoptotic cells, in a process termed LC3-associated phagocytosis. The process of LAP leads to an efficient degradation, promoting tolerogenic pathways by means of IL10 (interleukin 10) and TGFB (transforming growth factor, β) induction and dampening production of proinflammatory IL1B and IL6.Citation15 Since autophagy helps in shaping an anti-inflammatory environment, autoimmune disorders are prevented, as defects may lead to inflammation, tissue damage, or even Parkinson disease.Citation16 Depending on which antigens are presented, autophagy may favor an inflammatory or immune-silencing response. Consequently, T-cell polarization to T-helper 1 and T-helper 2 may change, a balance which plays a pivotal role in Leishmania disease outcome.Citation17-20 In addition to autophagy as an immune-defense mechanism, pathogens have exploited the functions of the autophagy-related proteins hereby securing survival. Autophagy plays a positive role in the replication of Brucella abortus and may assist in HIV biogenesis.Citation21,22 Moreover, induction of autophagy correlates with an increased Leishmania parasite load.Citation23 Focusing on the latter one, we could already show that the virulent Leishmania inoculum consists partially of apoptotic-like parasites, through which disease development can occur.Citation1 In the present study we first characterized the apoptotic population and then determined the fate of apoptotic-like Leishmania parasites in human host macrophages and its significance for T-cell activation. We demonstrated apoptotic-like parasites to activate the macrophages´ autophagy machinery, hereby dampening T-cell responses and enhancing parasite survival. Finally, our data define a novel mechanism, explaining the benefit of apoptosis-like cell death in a single-cell parasite for the survival of the overall population.
Results
The infective Leishmania parasite inoculum contains SubG1 phase-positive, TUNEL-positive and annexin V binding promastigotes.
In order to characterize the apoptotic-like population in promastigote cultures in more detail, we focused on guidelines of the Nomenclature Committee on Cell Death used to define cell death.Citation24 We performed cell cycle analysis, TUNEL staining, combined with a phosphatidylserine (PS) specific antibody as well as ANXA5 (annexin V) stainings on different growth stages of Lm promastigotes. By cell cycle analysis we could demonstrate that only a minority (20.8% ± 1.1) of the Leishmania infectious inoculum, defined as stationary growth phase Lm (stat.ph. Lm) is in the G1 phase () whereas the majority (78.7% ± 3.5) of logarithmic growth phase Lm (log.ph. Lm) is in the G1 phase (). Moreover, the greater part (74.8% ± 1.2) of stat.ph. Lm is in the SubG1 phase, a marker for dying and apoptotic cells ().Citation24 We analyzed DNA fragmentation by means of a TUNEL assay and assessed ANXA5 binding. We could show stat.ph. Lm to contain a proportion of cells being TUNEL+ (42.4% ± 1.5) and ANXA5-binding+ (57.3% ± 5.6). In contrast, among log.ph. Lm significant less parasites are TUNEL+ (18.3% ± 2.3) and ANXA5-binding+ (11.1% ± 5.9) (). Using a PS-binding antibody in combination with a TUNEL assay, we could distinguish between parasites being solely PS+, (10.5% ± 3.3), or PS+TUNEL+ (16.4% ± 0.4), or only TUNEL+ (37.8% ± 0.8) (). Similar to ANXA5-binding promastigotes, PS+TUNEL+ Lm have a round-shaped body morphology and lack flagellular movement (). By magnetic separation (magnetic-associated cell sorting, MACS), using ANXA5 beads, we isolated viable from apoptotic-like parasites, the latter being in the SubG1 phase (93.0% ± 2.6), and TUNEL+ (71.6% ± 9.2) as well as ANXA5-binding+ (87.6% ± 3.9) (), consequently we termed these parasites apoptotic-like. Moreover, the purified non-ANXA5-binding Lm had only low levels of SubG1 phase (36.3% ± 7.7), TUNEL+ (30.4% ± 3.1) and ANXA5-binding+ (23.2% ± 0.5), similar as found for the log.ph. Lm and both these populations comprise a G2 phase (), indicating multiplying promastigotes. Therefore we termed these parasites viable. Subsequently, we induced apoptosis characteristics in promastigotes using either miltefosine or staurosporine.Citation5 Upon treatment of log.ph. Lm, a significant increase of parasites residing in the SubG1 phase was observed, whereas the population of G2 positive parasites was absent. Also ANXA5-binding and TUNEL positivity were significantly increased (Fig. S1). In the following experiments we used log.ph. Lm parasites as a model for ANXA5-depleted viable promastigotes.
Figure 1. The Leishmania apoptotic-like population consists of TUNEL-positive, ANXA5-binding-positive and SubG1 phase-positive promastigotes. (A–D) Cell cycle analysis was performed using PI staining of stat.ph Lm. (A) MACS ANXA5+ separated apoptotic Lm (B), viable parasites (C) and log.ph. Lm (D). (E) DNA fragmentation of stat.ph. Lm, log.ph. Lm, MACS separated viable or apoptotic-like Lm was assessed using TUNEL assay (black bars) and ANXA5 binding (white bars), as analyzed by flow cytometry. (F and G) Stat.ph. Lm (gray) and log.ph. Lm (black) were double-stained by a TUNEL and PS antibody staining. Samples were analyzed by flow cytometry (F) and immunofluorescence microscopy (G) as depicted (arrow head, PS-positive Lm; thick arrow, TUNEL-positive Lm; thin arrow, double-positive Lm). Data are presented as mean ± SEM of at least 3 independent experiments. The IF and flow cytometry micrographs are representative of at least 3 independent experiments.
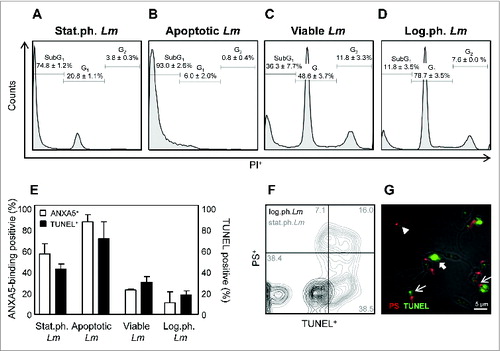
Apoptotic-like Leishmania induce LC3-associated-phagocytosis in primary human macrophages
Upon infection with stat.ph. Lm, consisting of viable and apoptotic parasites, we found 67.2% (± 2.1) of the parasites to reside in compartments, being positive for the autophagy marker LC3 (). Autophagy is known to be involved in the clearance of apoptotic cells.Citation25 Therefore we investigated whether the LC3+ phagosomes preferentially harbor the apoptotic promastigotes. Using ANXA5-MACS separated populations to infect hMDM we found that apoptotic-like parasites resided in a LC3+ compartment (92% ± 0.7) () whereas the majority of the viable parasites did not (7% ± 1) (). Furthermore, we could demonstrate that the rate of apoptotic-like parasites correlated with the amount of compartments harboring parasites, being LC3-positive (R = 0.98) (). In addition, we examined the autophagic activity using western blot analysis. Upon infection with log.ph. Lm (containing only a small fraction of apoptotic cells), already a significant increase in LC3-I to LC3-II was detected, which was even higher with increasing amounts of apoptotic-like Lm (stat.ph. Lm). In addition, we analyzed the expression of the ubiquitin-binding receptor SQSTM1, a marker of conventional autophagy of which the expression correlates inversely with LC3I-II conversion.Citation26 Upon infection with either of both Leishmania stages, no difference in SQSTM1 expression was observed (). In line with these data we analyzed by quantification of immunofluorescence stainings, that log.ph. Lm parasites induced only low levels of LC3+ compartments (34.7% ± 6.3) in hMDM, compared to infection with stat.ph. parasites (68.9% ± 3.8) (). A hallmark of autophagosomes is the formation of a multimembrane compartment.Citation27 To examine the ultrastructure of parasite-containing compartments, we preserved the samples by high-pressure freezing, followed by electron microscopy analysis. Upon internalization of ANXA5-binding apoptotic-like parasites by hMDM, we found 96.1% (± 1.8) of all apoptotic-like parasites (n = 134) to reside in compartments consisting of a single lipid bilayer (). Taken together, these data suggest LAP to play a role in the biogenesis of phagosomes containing apoptotic-like Lm parasites.
Figure 2. Apoptotic-like Leishmania parasites enter a LC3+ single-membrane compartment. (A–C) hMDM were infected with stat.ph. Lm (A), MACS separated apoptotic-like (B) or viable (C) parasites (MOI = 10). After 3 h, samples were fixed and antibody staining was performed to detect Lm parasites (green) and LC3 (red), counterstained with DAPI (blue) (arrow head, LC3- and Lm-positive; thick arrow, Lm-positive). (D) The apoptotic rate of the stat.ph. Lm, log.ph. Lm, MACS separated viable and apoptotic-like parasites was assessed by ANXA5-binding positivity in flow cytometry. Infection of hMDM with these Lm populations was performed for 3 h and subsequently samples were fixed, stained for Lm parasites and LC3 using antibodies, after which Lm-LC3 double-positive compartments were quantified by immunofluorescence analysis. As depicted, the apoptotic rate of the Lm population was plotted against the percentage of Lm-LC3 double-positive compartments, showing a correlation R. (E and F) hMDM were infected with stat.ph. or log.ph. Lm (MOI = 10). After 3 h, lysates were made after which LC3 conversion (black bars) and SQSTM1 level (white bars) were assessed by western blot and densitometry analysis. SQSTM1 level was normalized to ACTB expression (E). A representative immunoblot is depicted (F). (G) hMDM were infected with stat.ph. Lm (MOI = 10). After 3 h, samples were prepared for electron microscopy (EM) analysis, as described in Materials and Methods. The EM micrograph shows the membrane of an apoptotic Lm (white arrow) residing in a single membrane compartment (black arrow). Data are presented as mean ± SEM and immunoblots/IF/EM images are representative of at least 3 independent experiments.
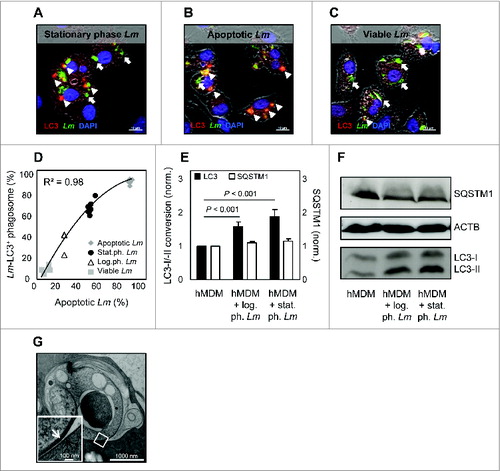
The presence of apoptotic-like parasites reduces Leishmania-induced T-cell proliferation
To investigate the consequence of Lm infection on a T-cell level, a carboxyfluorescein succinimidyl ester (CFSE)-based lymphocyte proliferation assay was established. As most donors in Germany are vaccinated for tetanus toxoid (TT), this peptide was used as a positive control for which we expected a T-cell response. Upon TT treatment of hMDM for 24 h following coincubation of autologous CFSE-labeled peripheral blood mononuclear cells (PBMCs), a proliferating, CFSElow T-cell subset (19.1% ± 1.7) could be detected after 6 d (). To analyze whether Leishmania modulate TT-induced proliferation, hMDM were treated with TT in combination with log.ph. Lm. Remarkably, after cocultivation of autologous CFSE labeled PBMCs, an even higher proliferating response (47.2% ± 3.0) was observed compared to proliferation induced by TT alone. Analyzing proliferation upon treating hMDM with TT in combination with apoptotic Lm (stat.ph. Lm), a significant reduction of proliferation could be demonstrated (33.9% ± 2.5) (). Interestingly, this significant discrepancy could also be observed in the absence of TT; hMDM infected with log.ph. Lm only induced a strong proliferation (31.1% ± 2.1), which was significantly reduced in the presence of apoptotic-like parasites (19.3% ± 1.8). To strengthen the finding that the presence of apoptotic-like parasites leads to a reduced proliferation, apoptosis was chemically induced in parasites. Log.ph. or stat.ph. Lm were treated with apoptosis-inducing drugs, staurosporine (25 μM) or miltefosine (25 μM), for 48 h (Fig. S1). Proliferation, induced by infected hMDM, was significantly reduced upon induction of apoptosis in log.ph. (4.4% ± 1.1 ; 4.9% ± 3.1) or stat.ph. Lm (6.4% ± 1.8; 3.8% ± 1.0), by staurosporine or miltefosine treatment, compared to untreated Lm (28.8% ± 1.9 ; 18.9% ± 1.7) (). By applying an analysis algorithm we were able to calculate the frequency of precursor cells after TT stimulation (0.010% ± 0.002), comprising antigen-specific cells and cells that proliferated due to bystander activation. In comparison we found a similar frequency (0.018% ± 0.005) after Lm stimulation ().
Figure 3. The presence of apoptotic-like parasites reduces T-cell proliferation upon Lm infection. (A) hMDM were coincubated with tetanus toxoid (TT; 10 μM) alone or in combination with stat.ph. Lm or log.ph. Lm (MOI = 10). After 24 h hMDM were washed and cocultured with autologous CFSE labeled PBMCs (ratio 1:5). After 6 d the CFSElow, proliferating cells were quantified by flow cytometry (n = 4 to 73). (B) hMDM were infected with log.ph. and stat.ph. Lm (MOI = 10) which were untreated or treated with apoptosis inducing drugs, 25 μM miltefosine (gray bars) or 25 μM staurosporine (white bars), for 48 h prior to infection. After 24 h of infection, hMDM were washed and cocultured with autologous CFSE labeled PBMCs. After 6 d the CFSElow, proliferating cells were quantified by flow cytometry (n = 6). (C) Precursor frequency of proliferating cells in response to Leishmania or TT was calculated, as described in Materials and Methods (n= 6 to 11). (D) Proliferation could be inhibited dose dependently by anti-MHCII antibodies (gray bars), not by anti-MHCI antibodies (white bars), as described in Materials and Methods. (E) hMDM were infected with log.ph. Lm and stat.ph Lm (MO = I10) for 24 h in the presence of 30 nM bafilomycin A1 (BAFA1). After 24 h of infection, hMDM were washed and cocultured with autologous CFSE labeled PBMCs. After 6 d the CFSElow, proliferating cells were quantified by flow cytometry (n = 6). Data, presented as mean ± SEM from at least 3 independent experiments.
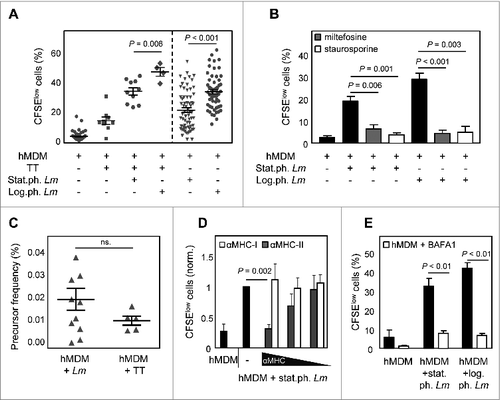
Upon phenotyping the proliferation subset, upon either infection with stat.ph. or log.ph. Lm we gated using the FSC/SSC on the lymphocyte proliferation. Subsequently the proliferating CFSElow PBMCs was found to be CD3+, being T lymphocytes, the majority of which were CD4+ positive (Fig. S2C). Activation of CD4+ T-cells requires antigen presentation by MHCII molecules. To test the MHC restriction in our human in vitro system we treated Lm infected hMDM with blocking antibodies specific for human MHCI or MHCII. After 6 d of cocultivation with autologous PBMCs, T-cell proliferation was analyzed by flow cytometry. No blocking effect of MHCI antibodies was detectable (). By contrast, when exposing hMDM to increasing amounts of MHCII specific antibodies, a dose-dependent reduction of CFSElow PBMCs was observed. Proliferation was significantly reduced (0.3 ± 0.01 fold change) compared to the control, when treating hMDM with anti-MHCII antibodies at the highest tested dose. In addition, inhibition of lysosomal acidification by bafilomycin A1, hereby preventing MHCII antigen processing, also reduced proliferation significantly during infection of hMDM with log.ph. Lm (42.1% ± 2.9 to 6.7% ± 1.2) and stat.ph. Lm (32.6% ± 4.1 to 8.0% ± 1.3) ().
Different hypotheses about the reduction in T-cell proliferation caused by the apoptotic-like parasites can be raised. A first parameter influencing proliferation may be antigen availability. During the process of apoptosis various proteins are targeted for degradation. To exclude the possibility that the reduced protein amount in apoptotic-like parasites could be responsible for the significant lower proliferation, protein levels were normalized. By applying the micro-Kjeldahl method the protein concentration of the parasites in both growth stages was determined.Citation28 Next, hMDM were infected with log.ph. (multiplicity of infection [MOI] = 10]) and stat.ph. Lm parasites (MOI = 17), normalized for protein content. However, hMDM infected with stat.ph. Lm parasites (MOI = 17) still induced a significant lower proliferation (18.9% ± 3.6), compared to proliferation induced by hMDM harboring viable parasites (27.2% ± 2.8) (Fig. S2A). Second, the importance of parasite integrity was assessed. Stimulating hMDM with soluble Leishmania antigen led to a significant reduction of proliferation, compared to proliferation induced upon infection with intact parasites (Fig. S2A). Third, parasites like trypanosomes and Leishmania are able to secrete and excrete antigens themselves which might be able to induce proliferation early after exposure to immune cells.Citation29,30 To determine whether the observed T-cell response is elicited by secreted antigens and thus dependent on the viability of the parasites, hMDM were incubated with the supernatant fraction, collected from log.ph. Lm and stat.ph. Lm parasite cultures and subsequently cocultivated with autologous PBMCs. After 6 d, no significant higher proliferation could be observed compared to proliferation induced by the untreated hMDM (data not shown). Moreover, analyzing proliferation over time showed proliferation to start at d 3 to 4 after PBMCs were cocultured (Fig. S2B). A fourth explanation lies in the fact that parasites are able to directly interact with PBMCs as described for natural killer cells.Citation31 To address this question, we directly cocultured parasites with PBMCs, containing T-cells, as well as monocytes and dendritic cells. However, only a minor proliferation of CD4+ cells (6.2% ± 1.7) could be observed (data not shown). In all, these data demonstrate human primary macrophages, upon Leishmania infection, to induce an antigen-specific, MHCII-dependent T-cell response, which is dampened in the presence of apoptotic-like parasites.
T-cell proliferation contributes to a reduced Lm infection
Activated T lymphocytes are known to be important in clearing intracellular pathogens. To investigate the consequences of T-cell proliferation on parasite infection rate and parasite load we used transgenic Lm parasites, of which the viable parasites express the dsRed protein.Citation32 This offered us the ability to use flow cytometry to analyze parasite survival by means of infection rate (dsRed+ hMDM) and parasite load (mean fluorescent intensity, MFI). Next, the hMDM were infected with log.ph. or stat.ph. transgenic Lm parasites for 24 h, following coculturing with or without PBMCs for an additional 5 to 6 d. The presence of proliferating PBMCs significantly reduced the infection rate () (stat.ph. Lm: 1.7 fold; log.ph. Lm: 2.2 fold) and diminished the parasite load () (stat.ph. Lm: 1.4 fold; log.ph. Lm: 1.4 fold), suggesting a role for T lymphocytes in reducing intracellular parasite survival. Remarkably, in the presence of apoptotic-like parasites, a significant higher overall parasite survival was achieved, which manifests itself in an increased infection rate and parasite load. In conclusion, since the stat.ph. Lm inoculum contains apoptotic-like promastigotes these data demonstrate the importance of the apoptotic-like parasites to secure survival of the overall population.
Figure 4. Proliferation leads to a reduced parasite survival. (A and B) hMDM were infected with log.ph. or stat.ph. transgenic dsRed expressing Lm (MOI = 10). After 24 h hMDM were washed following coculturing with (w/, black bars) or without (w/o, white bars) autologous CFSE labeled PBMCs (ratio 1:5). After 6 d, infection rates of hMDM were analyzed by flow cytometry, as dsRed (PE+) positive hMDM (A). The mean fluorescence intensity (MFI) of Lm dsRed infected (PE+) hMDM were analyzed to determine the parasite load (B). Data were normalized (n = 9 to 13). (C) Representative DiffQuick© pictures of hMDM infected with log.ph. or stat.ph. Lm in the presence or absence of autologous PBMCs, are depicted. Data, presented as mean ± SEM, are of 6 independent experiments.
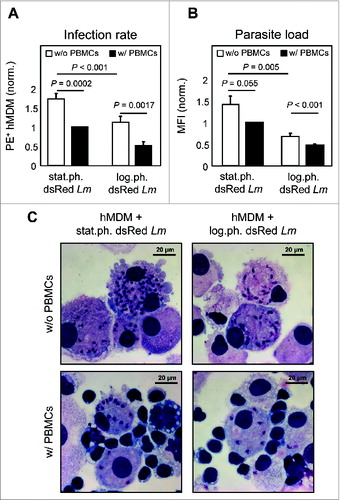
In the presence of apoptotic-like parasites, production of proinflammatory cytokines is reduced
Next we examined the cytokine profile of hMDM infected with log. or stat.ph Lm 24 h post infection and 6 d after PBMC cocultivation. Upon infection of hMDM with log.ph. Lm an increased production of the proinflammatory IL1B, IFNG, IL6 and TNF, was observed, of which the latter 2 were significantly lower in the presence of apoptotic-like parasites (stat.ph. Lm) (). The anti-inflammatory IL10 was produced in significantly greater amounts upon infection of hMDM with log.ph. Lm (). After PBMC cocultivation we could again demonstrate a strong proinflammatory response, primarily by the production of IFNG upon of infection with log.ph. Lm. In the presence of apoptotic-like parasites, the production of IFNG was significantly reduced ().
Figure 5. In the presence of apoptotic-like parasites, production of proinflammatory cytokines is reduced. (A–E) hMDM (gray bars) were infected with log.ph. (black bars) or stat.ph. Lm (white bars) (MOI = 10). After 24 h, infected and control hMDM were washed and the supernatant fraction was collected. Next, autologous CFSE labeled PBMCs were cocultured (ratio 1:5). After 6 d, also supernatant fraction was collected. The supernatant fractions, 24 h post infection (w/o PBMCs) and 6 d after coculturing (w/ PBMCs), were tested for the presence of TNF (A), IL6 (B), IL1B (C), IFNG (D) and IL10 (E) by flow cytometry using a BD Cytometric Bead Array (n = 4 to 8). Data are presented as mean ± SEM, of 3 independent experiments; ND not determinable.
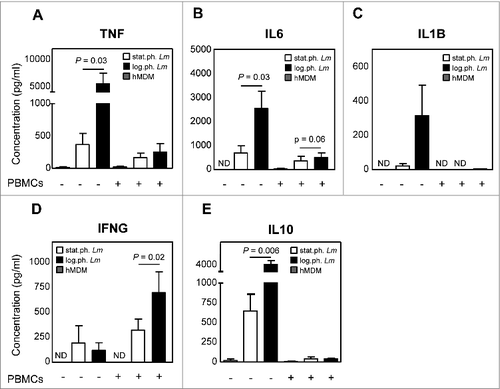
The autophagy machinery, as a negative regulator of T-cell proliferation
The uptake of apoptotic-like cells by macrophages is known to activate the autophagy machinery, leading to efficient degradation and an anti-inflammatory environment.Citation15 To mimic the role of the autophagy machinery and apoptotic-like promastigotes, we chemically modulated autophagy in human primary macrophages. As described, PI-103, rapamycin and AZD-8055 are known to interact with the mechanistic target of rapamycin (MTOR), a master regulator of autophagy.Citation33,34 Upon autophagy induction (30 min) in hMDM, autophagy activity was elevated upon treatment with rapamycin (1.6 ± 0.3 fold change) (Fig. S3A), as also for AZD-8055 and PI-103 (data not shown). The increase in autophagy activity upon rapamycin treatment, was accompanied by an increased autophagy flux (3.2 ± 0.8 fold change), measured as LC3 accumulation, upon bafilomycin A1 treatment (Fig. S3A and D).Citation35
In addition to LC3, we assessed protein expression of SQSTM1 by western blot analysis. At early time points after autophagy induction (30 min) using rapamycin, no difference in SQSTM1 levels could be observed compared to untreated hMDM (data not shown). However, after 2 h of rapamycin treatment of hMDM, a strong LC3I-II conversion was detected, as also a decreased amount of SQSTM1 protein (Fig. S3B and E). In a next step we used wortmannin and spautin-1 as negative modulators of autophagy. As wortmannin impaired uptake of Leishmania by hMDM (data not shown), we used spautin-1 and found upon treatment a decreased LC3I-II conversion and an increase in SQSTM1 protein level (Fig. S3C and E). Subsequently, we analyzed the effect of autophagy modulation on T-cell proliferation. To this end we induced autophagy in hMDM, followed by infection with viable parasites only (log.ph. Lm). After 6 d of coculturing with autologous PBMCs, a significant lower proliferation was induced by infected hMDM, treated with rapamycin (5.4% ± 1.6) (), PI-103 (15.1% ± 3.7) and AZD-8055 (19.3% ± 6.5) (data not shown), as compared to the control (42.5% ± 1.2). The reduction of proliferation was more pronounced compared to proliferation induced by hMDM in which apoptotic parasites reside (25.8% ± 2.1). We reasoned that autophagy induction might compromise uptake of Lm parasites leading to the reduced T-cell activation. However after 24 h, no reduction in infection rate was detected regardless whether macrophages were treated with autophagy modulators or not. Only with PI-103 treatment a slight reduction was observed (data not shown). By modulating autophagy negatively using spautin-1, we could observe an elevated proliferation (31.8% ± 5.8), which did not differ significantly from proliferation induced by log.ph. Lm (). Finally we assessed the effect of autophagy induction on parasite survival during PBMC cocultivation. In the presence of T-cells, the induction of autophagy secured intracellular Lm survival. Lm infection rate increased up to 3.3 (± 0.2) fold upon treatment with rapamycin (), 1.3 (± 0.1) fold using PI-103, 2.1 (± 0.1) fold using AZD-8055 or (data not shown). By modulating autophagy negatively, no difference was observed in parasite survival (data not shown). These data suggest that the activation of the autophagy machinery, induced chemically or by apoptotic-like parasites, leads to an increased overall parasite survival.
Figure 6. Autophagy induction leads to a reduced T-cell proliferation and an enhanced parasite survival. (A) hMDM were treated with rapamycin (1 μM) or spautin-1 (10 μM) 30 min and 24 h, respectively, prior to infection with log.ph. (black bars) or stat.ph. Lm parasites (gray bars) (MOI = 10). After 24 h hMDM were washed and cocultured with autologous CFSE labeled PBMCs (ratio 1:5). After 6 d the CFSElow proliferating cells were quantified by flow cytometry (n = 7 to 24). (B) hMDM were treated with rapamycin (1 μM) or spautin-1 (10 μM) 30 min or 24 h prior to infection with log.ph. (black bars) or stat.ph. (gray bars) transgenic dsRed parasites (MO = I10). After 24 h hMDM were washed and cocultured with autologous PBMCs (ratio 1:5). After 6 d, Lm infection rates (PE+) of hMDM were analyzed by flow cytometry (n = 5 to 14). Data are presented as mean ± SEM and are representative of at least 3 independent experiments.
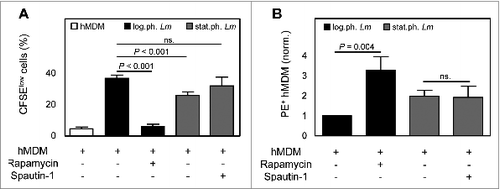
Discussion
An optimal T-cell response is of great importance for intracellular pathogen clearance. Our data indicate that in the presence of proliferating T-cells, the Leishmania infection rate and parasite load in hMDM is reduced. The observed T-cell activation was depending on antigen processing and MHCII restricted, which is in agreement with reports describing a proliferation of naive CD4+ T-cells upon stimulation with membrane derived antigens from Leishmania infantum.Citation36 We assessed the precursor frequencies after Leishmania infection based on CFSE dilutions. We found them to be higher compared to antigen-specific T-cell frequencies obtained by tetramer staining. This is in line with reports of Danke et al. and Novak et al. showing only a minority of the CFSElow proliferating cells to be antigen specific. A majority (80% to 96%) of tetramer-negative proliferating cells was found to proliferate as a result of bystander activation.Citation37,38
To circumvent identification and elimination through the immune system and secure survival, intracellular pathogens have evolved strategies to silence their host cells. The bacterium M. tuberculosis as well as the protozoa Toxoplasma try to escape from the phagosome upon phagocytosis into the cytoplasm, whereas C. burnetti delays the autophagolysosomal maturation to benefit its own survival.Citation39-41 Similarly, Leishmania donovani delays phagolysosomal maturation to transform into the disease-propagating amastigote form.Citation42 A recent study in mice using L. donovani demonstrated a reduced T-cell proliferation caused by the inhibition of Leishmania antigens cross-presented on MHC class I molecules.Citation43 In agreement with these data we could observe Leishmania major parasites to indirectly reduce T-cell proliferation and this inhibitory effect was depending on the presence of apoptotic-like Leishmania parasites.
Apoptosis among parasites is still extensively debated. Various reports state that parasites use “apoptotic mimicry” in order to silence phagocytes enabling survival.Citation44,45 By applying unified criteria of the Nomenclature Committee on Cell Death, we could define a subpopulation of the Leishmania infectious inoculum to show characteristics of apoptosis. Interestingly, viable parasites, residing in the G2 phase, also were shown to be positive for TUNEL staining, a finding which could be misinterpreted as apoptotic mimicry. Taking a closer look at these viable parasites, negative for ANXA5-binding, we observed only the kinetoplast, comprising maxi- and minicircles DNA, to stain TUNEL-positive. In line with previous observations, this phenomenon is explained by the fact that prior to Leishmania divisions, the progeny minicircles replicate and contain nicks or gaps.Citation46,47 These nicks and gaps are repaired upon division, however our data suggest that these nicks are detectable by TUNEL staining in stages where the parasites is preparing for division.Citation48
While apoptosis-like cell death plays a crucial role for population survival, also for several other protozoan parasites, it is still a matter of debate how cell death could be beneficial for a single-cell organism. As Leishmania parasites are transferred by the sandfly, apoptosis-like cell death could serve to restrict population growth in the vector, hereby increasing the life span of the vector and increasing the transmission rate, as shown for other protozoan parasites.Citation3,4 From another point of view it is also tempted to speculate that the presence of apoptotic-like parasites has an immune-silencing effect, as has been shown upon apoptotic cell clearance during homeostasis. Santos et al. already showed that phosphatidylserine exposure by Toxoplasma gondii is fundamental for granting survival of the parasite in murine macrophages.Citation7 In concordance, phagocytosis of apoptotic cells by macrophages plays a role in Trypanosoma cruzi persistence.Citation6 Indeed, we have also found that during Leishmania infection, disease development relies on the presence of apoptotic-like promastigotes as infection of mice with only viable Leishmania promastigotes does not result in disease development.Citation1 In this study employing human primary cells, we could confirm apoptotic-like parasites to be crucial for an enhanced parasite survival. To clarify how the apoptotic-like population secures survival of the viable ones, we analyzed their intracellular fate. Upon phagocytosis, apoptotic-like parasites were found to reside in a compartment decorated with the autophagy marker LC3. This finding is in agreement with murine data showing that Leishmania parasites engage PI3K-AKT signaling, which is an upstream event able to initiate autophagy.Citation49 In contrast to conventional autophagy, only a single lipid bilayer was found to surround the parasite, indicating that processing of apoptotic-like parasites occurred in a compartment formed by LC3-associated phagocytosis.Citation13,50,51 Moreover, we found the protein SQSTM1/p62 not to be involved in the process of LAP induced by apoptotic-like parasites, as described for Listeria as well.Citation52 As described, autophagy is a key player in maintaining homeostasis, enabling phagocytes to clear apoptotic and necrotic cells efficiently and silently to prevent inflammation and development of autoimmune disorders, like systemic lupus erythematous.Citation23 Also in the presence of apoptotic parasites, immune responses were dampened, as infected hMDM induced a lower T-cell proliferation. Clearance of dying cells or even apoptotic-like Lm is known to create a more anti-inflammatory environment that is dominated by cytokines such as IL10 and TGFB and suppression of TNF, IL6 and IL1B.Citation1,15 Although uptake of apoptotic-like parasites led to a reduced IL6 and IL1B production, IL10 was strongly produced in response to viable parasites. This is in agreement with the fact that a strong proinflammatory response may be dampened by anti-inflammatory mediators.Citation53 Tiemessen et al. showed that TGFB has a pronounced inhibitory effect on proliferation of antigen-specific CD4+ T cells, a mechanism that could also be functional in our context.Citation54 Alternatively, the activation of the autophagy machinery could also lead to an increased presentation of innocuous self-antigens, which contributes to tolerance-inducing mechanisms also dampening adaptive immune responses.Citation55,8 As a consequence of the reduced T-cell response, the parasite´s survival was enhanced, leading to the assumption that activation of the autophagy machinery is beneficial for the parasite. To underscore this statement, we could demonstrate that induction of autophagy by rapamycin or by other means, hereby simulating the role of apoptotic-like parasites, even led to a higher infection rate, which was previously observed upon infection with the related protozoa T.cruzi.Citation56 In addition, Roberta O. Pinheiro et al. have found that autophagy increases the parasitic replication of Leishmania amazonensis inside macrophages.Citation23 In contrast, our data did not indicate autophagy to influence replication. However our data suggest that an elevated autophagy activity prevents the elimination of promastigotes. The increased amount of viable promastigotes is then able to transform into the amastigote form, presumably being the source of a higher parasite load. Upon modulating autophagy negatively, we observed T-cell proliferation to be slightly increased, without having a profound effect on parasite survival. We used spautin-1, which targets BECN1/Beclin 1 for degradation, which fit the data of Martinez et al. stating that LAP depends on BECN1.Citation57,59 In addition, LAP is induced by several stimuli-like ROS, TLR ligands, and PS receptors like TIM4.Citation60,50 In our model, inhibiting one of these potential LAP-inducing signals might strongly influence LAP with a consequence for Leishmania parasites survival.
In conclusion, cell death among Leishmania parasites is necessary to guarantee an overall parasite population survival. Apoptosis-like cell death is used as a “death-deceiving” immune evasion mechanism to silence phagocytes, involving the host-cell autophagy machinery, resulting in a significantly reduced T-cell proliferation. This promotes overall parasite survival in the host, thereby defining a novel mechanism, which explains the benefit of apoptosis-like cell death in a single-cell parasite. Additionally, our data define the host autophagy pathway as a potential therapeutic target in treating Leishmaniasis.Citation58
Materials and Methods
Leishmania major parasites
Leishmania major (MHOM/IL/81/FEBNI) parasites, wild-type, and transgenic parasites, were cultured as described.Citation61,62 Apoptotic and viable promastigotes were purified from stationary phase promastigotes by magnetic separation, as described previously.Citation1
Assessing apoptosis
To assess apoptosis-like cell death, log.ph. Lm were treated with 25 μM staurosporine (Sigma, S4400) or 25 μM miltefosine (Calbiochem, 475841) for 48 h. DNA fragmentation was assessed by flow cytometry or immunofluorescence imaging using an in situ cell-death detection kit, based on terminal deoxynucleotidyl transferase dUTP nick end labeling (TUNEL) (Roche, 11684795910), as described by the manufacturer. PS exposure was analyzed by ANXA5/annexin V (Invitrogen, A-23204) binding or PS antibody (Upstate, 05-719) positivity, using flow cytometry. Cell cycle analysis was performed using propidium iodide staining, as described.Citation66
Human peripheral mononuclear cells
Human peripheral mononuclear cells (PBMCs) were isolated from buffy coats (DRK-Blutspendedienst Hessen GmbH, 506838) by passage over a Leukocyte Separation Medium (PAA, J15-004) gradient. The donors were healthy German adults without known exposure to Leishmania parasites. After separation, PBMCs were washed and resuspended in complete medium (CM), consisting out of RPMI 1640 (R0883) supplemented with 10% heat inactivated fetal calf serum (F7524), 50 μM β-mercaptoethanol (M6250) (all from Sigma Aldrich), 2 mM L-glutamine (K 0282), 100 U/ml penicillin and 100 μg/ml streptomycin (A 2213), 10 mM HEPES (L 1613) (all from Biochrom). Monocytes were isolated by exploiting their ability to adhere to plastic, or by CD14 positive selection (Miltenyi, 130-050-201). Nonadherent or CD14-negative cells were collected and frozen in CM containing 40% fetal calf serum and 10% DMSO for lymphocyte proliferation assays. Monocytes were differentiated into human monocyte derived macrophages by addition of 10 ng/ml recombinant human macrophages colony stimulating factor (M-CSF) (R&D, 216-MC), for a period of 5 to 7 d at 37°C, 5% CO2.
Infection of human primary macrophages
Adherent hMDM were detached, counted and subsequently seeded in 96-well plates, chamber slides or 1.5 ml microcentrifuge tubes. For infection, Lm promastigotes (logarithmic/stationary phase/apoptotic/viable) were added at an MOI of 1:10. After 3 or 24 h of incubation at 37°C, 5% CO2, extracellular parasites were removed by (i) washing 96-well plates and chamber slides with CM or (ii) centrifugation of microcentrifuge tubes. Freshly prewarmed CM was added to the infected hMDM and the samples were further incubated at 37°C until the desired time point of interest was reached. As a readout to assess infection rate and parasite load, FACS analysis of hMDM infected with transgenic parasites, or histological staining using Diff Quick (Medion Diagnostics AG, 130834, 130835, 130833) of hMDM infected with wild-type parasites, were performed.
During infection experiments, autophagy was induced by preincubation of hMDM with 10 μM of PI-103 (S1038), 10 μM of AZD-8055 (S1555) or 1 μM of rapamycin (S1039) (all from Selleckchem) for 30 min prior to infection, following washing. To investigate the autophagy flux, hMDM were treated with 30 nM of bafilomycin A1 (Sigma, B1793) for 1 h, following autophagy induction or Lm infection. To negative modulate autophagy, hMDM were pretreated with 20 μM spautin-1 (Sigma, SML0440) for 24 h. After the given time points, lysates were made or infection experiments were performed.
Immunofluorescence analysis
For immunofluorescence (IF) staining, hMDM containing chamber slides were fixed in 4% paraformaldehyde. Cells were permeabilized by saponin (Sigma, S7900) and stained with DAPI, a polyclonal rabbit anti-human LC3 Ab (Cell Signaling Technology, 2775S), and an anti-Lm polyclonal Ab obtained from Lm infected Balb/c mice.Citation62 For detection, chicken anti-mouse Alexa 488 (A21200) and goat anti-rabbit Alexa Fluor 568 (A11036) were used (Molecular Probes/Invitrogen). Chamber slides were analyzed using a Zeiss Observer, Zeiss LSM7 Live and Axiovision/ZEN 2009 Software (Carl Zeiss, Göttingen, Germany). Quantification of LC3+ compartments was assessed by counting > 20 Lm infected cells.
Transmission electron microscopy
The hMDM were seeded on carbon-coated sapphire discs (3 mm in diameter) in 6-well cell culture plates and infected with Lm promastigotes (MOI = 10). After coincubation for 3 h extracellular parasites were removed by washing. Subsequently the viable cells were directly frozen by high-pressure freezing with an HPF 01 apparatus. Samples were freeze substituted in acetone containing 0.1% (w/v) uranyl acetate, 0.2% (w/v) osmium tetroxide and 5% (v/v) water and embedded in epon as described previously.Citation63,64 The samples were imaged with a Zeiss EM109 transmission electron microscope (Carl Zeiss, Oberkochen, Germany) at an acceleration voltage of 80 kV.
Immunoblotting
Microcentrifuge tubes, containing hMDM (0.5 × 10Citation6), were centrifuged and washed with cold phosphate-buffered saline (distilled water containing 137 mM NaCl, 2.7 mM KCL, 8.1 Na2hPO4, 1.5 mM KH2PO4, pH 7.1, in house facility, Langen). Pellets were lysed with Laemmli at 95°C for 10 min. Samples were separated on 15% polyacrylamide SDS-PAGE gels and transferred onto nitrocellulose membranes. The membrane was blocked with TBST (PBS containing 0.05% Tween 20, Sigma, 93773) (+ 5% milk powder) following overnight incubation at 4 °C with a polyclonal rabbit anti-human LC3 Ab (Cell Signaling Technology, 2775S), anti-SQSTM1/p62 (Santa Cruz Biotechnology, sc-28359) or ACTB/β-actin (Cell Signaling Technology, 8H10D10) diluted in TBST (+ 5% Albumin Fraction V, PanReac AppliChem, A1391). The membrane was incubated with HRP-conjugated secondary antibodies (Thermo Scientific Pierce, 31430 and Santa Cruz Biotechnology, sc-2004/sc-2005) in TBST (+ 5% Albumin Fraction V) for 1 h at room temperature, following visualization with chemiluminescence protein detection reagents (GE Healthcare, RPN2109). Protein quantification in western blots was performed using ImageJ.
CFSE-based proliferation assay
The hMDM were stained, using CFSE (Sigma, C1157), modified from protocols described previously.Citation65 Briefly hMDM were incubated with CFSE (1 to 5 μM) followed by incubation at 37°C for 10 min. After washing with CM, cells were resuspended and seeded in 1.5 ml eppendorf tubes at 0.4 × 10Citation6/ml. The hMDM were infected with Lm parasites (MOI = 10). After 24 h incubation, 37°C, 5%CO2, extracellular parasites were removed by washing and hMDM were put in new 1.5 ml microcentrifuge tubes or in 96-well, round bottom plates. Autologous PBMCs were thawed and similarly labeled with CFSE. The PBMCs were coincubated with the (un)infected hMDM, at a ratio of 1:5. After 6 d of culture at 37°C, 5% CO2, cells were collected and proliferation (CFSElow cells) was analyzed by flow cytometry.
Ag processing and presentation assays
MHC blocking antibodies (MHCII L243 and MHCI W6/32) were produced in a hybridoma cell line. The supernatant fraction was added to hMDM in various dilutions following Leishmania infection (MOI = 10). To assess antigen processing, 30 nM bafilomycin A1 (Sigma, B1793) was added to the hMDM during infection. After 24 h of infection, autologous CFSE labeled PBMCs were coincubated (ratio 1:5). After 6 d, proliferation (CFSElow population) was assessed by flow cytometry.
Cytometric bead array
To profile cytokine production, hMDM were infected with Lm (MOI = 10) for 24 h after which the supernatant fraction was collected. Infected hMDM were additionally cocultured with autologous PBMCs for 6 d, after which the supernatant fraction was also collected. Both samples were tested for the presence of IL6, IL10, TNF, IFNG and IL1B using the BD CBA Flex Set System, according to the manufacturer's instruction (BD Bioscience, 558276, 558274, 560112, 560111, 558279).
Flow cytometry
FACS analysis of Leishmania promastigotes was performed as described.Citation62 To determine the apoptotic rate of the promastigotes populations, parasites were stained using ANXA5 Alexa Fluor 647 (Molecular Probes/Invitrogen, A23204). For FACS analysis of human cells, 0.3 × 10Citation6 cells were labeled with mouse anti-human CD3-PE (556612), CD4-PE (555347), or CD8-pacific blue (558207), all purchased from BD Bioscience and the additional isotype controls as defined by the manufacturer. Upon analyzing, at least 10,000 events (human cells) or 20,000 (parasites) were recorded using a BD LSR II flow cytometer (BD Bioscience, Heidelberg, Germany). Data were analyzed by BD FACS Diva or FlowJo software (Treestar). Precursor frequencies of T-cells were calculated using FlowJo software (Treestar), based on an algorithm as described.Citation67
Statistical analysis
Data were assessed for normality using a D’Agostino and Pearson omnibus normality test. Subsequently statistical analysis was determined by a paired Student t test using Microsoft Excel and Graph-Pad Prism version 4. A value of P < 0.05 was considered statistically significant.
Disclosure of Potential Conflicts of Interest
No potential conflicts of interest were disclosed.
Supplemental Material
Supplemental data for this article can be accessed on the pub-lisher's website.
SupplementalFigures.zip
Download Zip (2.2 MB)Acknowledgments
We thank U. Ritter for carefully reading and commenting on this manuscript. We thank Vanessa Stuppi and Bianca Walber for the technical support.
References
- van Zandbergen G, Bollinger A, Wenzel A, Kamhawi S, Voll R, Klinger M, Müller A, Hölscher C, Herrmann M, Sacks D, et al. Leishmania disease development depends on the presence of apoptotic promastigotes in the virulent inoculum. Proc Natl Acad Sci USA 2006; 103:13837-42; PMID:16945916; http://dx.doi.org/10.1073/pnas.0600843103
- Kaczanowski S, Sajid M, Reece SE. Evolution of apoptosis-like programmed cell death in unicellular protozoan parasites. Parasit Vectors 2011; 4:44; PMID:21439063; http://dx.doi.org/10.1186/1756-3305-4-44
- Barcinski MA, DosReis GA. Apoptosis in parasites and parasite-induced apoptosis in the host immune system: a new approach to parasitic diseases. Braz J Med Biol Res 1999; 32:395-401; PMID:10347800
- Al-Olayan EM, Williams GT, Hurd H. Apoptosis in the malaria protozoan, Plasmodium berghei: a possible mechanism for limiting intensity of infection in the mosquito. Int J Parasitol 2002; 32:1133-43; PMID:12117496
- Lüder CG, Campos-Salinas J, Gonzalez-Rey E, van Zandbergen G. Impact of protozoan cell death on parasite-host interactions and pathogenesis. Parasit Vectors 2010; 3:116; PMID:21126352; http://dx.doi.org/10.1186/1756-3305-3-116
- Freire-de-Lima CG, Nascimento DO, Soares MB, Bozza PT, Castro-Faria-Neto HC, de Mello, F G, DosReis GA, Lopes MF. Uptake of apoptotic cells drives the growth of a pathogenic trypanosome in macrophages. Nature 2000; 403:199-203; PMID:10646605; http://dx.doi.org/10.1038/35003208
- Santos, Thiago Alves Teixeira dos, Portes, Juliana de Araújo, Damasceno-Sá JC, Caldas LA, Souza Wd, Damatta RA, et al. Phosphatidylserine exposure by Toxoplasma gondii is fundamental to balance the immune response granting survival of the parasite and of the host. PLoS ONE 2011; 6:e27867; PMID:22140476; http://dx.doi.org/10.1371/journal.pone.0027867
- Dengjel J, Schoor O, Fischer R, Reich M, Kraus M, Müller M, Kreymborg K, Altenberend F, Brandenburg J, Kalbacher H, et al. Autophagy promotes MHC class II presentation of peptides from intracellular source proteins. Proc Natl Acad Sci USA 2005; 102:7922-7; PMID:15894616; http://dx.doi.org/10.1073/pnas.0501190102
- Liu G, Bi Y, Wang R, Wang X. Self-eating and self-defense: autophagy controls innate immunity and adaptive immunity. J Leukoc Biol 2013; 93:511-9; PMID:23271703; http://dx.doi.org/10.1189/jlb.0812389
- Patterson NL, Mintern JD. Intersection of autophagy with pathways of antigen presentation. Protein Cell 2012; 3:911-20; PMID:23136066; http://dx.doi.org/10.1007/s13238-012-2097-3
- Levine B, Mizushima N, Virgin HW. Autophagy in immunity and inflammation. Nature 2011; 469:323-35; PMID:21248839; http://dx.doi.org/10.1038/nature09782
- Deretic V, Saitoh T, Akira S. Autophagy in infection, inflammation and immunity. Nat Rev Immunol 2013; 13:722-37; PMID:24064518; http://dx.doi.org/10.1038/nri3532
- Randow F, Münz C. Autophagy in the regulation of pathogen replication and adaptive immunity. Trends Immunol 2012; 33:475-87; PMID:22796170; http://dx.doi.org/10.1016/j.it.2012.06.003
- Gutierrez MG, Master SS, Singh SB, Taylor GA, Colombo MI, Deretic V. Autophagy is a defense mechanism inhibiting BCG and Mycobacterium tuberculosis survival in infected macrophages. Cell 2004; 119:753-66; PMID:15607973; http://dx.doi.org/10.1016/j.cell.2004.11.038
- Martinez J, Almendinger J, Oberst A, Ness R, Dillon CP, Fitzgerald P, Hengartner MO, Green DR. Microtubule-associated protein 1 light chain 3 alpha (LC3)-associated phagocytosis is required for the efficient clearance of dead cells. Proc Natl Acade Sci 2011; 108:17396-401; http://dx.doi.org/10.1073/pnas.1113421108
- Shintani T. Autophagy in health and disease: a double-edged sword. Science 2004; 306:990-5; PMID:15528435; http://dx.doi.org/10.1126/science.1099993
- Bourreau E, Prévot G, Gardon J, Pradinaud R, Hasagewa H, Milon G, Launois P. LACK-specific CD4(+) T cells that induce gamma interferon production in patients with localized cutaneous leishmaniasis during an early stage of infection. Infect Immun 2002; 70:3122-9; PMID:12011006
- Kariminia A, Bourreau E, Pascalis H, Couppié P, Sainte-Marie D, Tacchini-Cottier F, Launois P. Transforming growth factor beta 1 production by CD4+ CD25+ regulatory T cells in peripheral blood mononuclear cells from healthy subjects stimulated with Leishmania guyanensis. Infect Immun 2005; 73:5908-14; PMID:16113310; http://dx.doi.org/10.1128/IAI.73.9.5908-5914.2005
- Kurtzhals JA, Kemp M, Poulsen LK, Hansen MB, Kharazmi A, Theander TG. Interleukin-4 and interferon-gamma production by Leishmania stimulated peripheral blood mononuclear cells from nonexposed individuals. Scand J Immunol 1995; 41:343-9; PMID:7899822
- Sacks D, Noben-Trauth N. The immunology of susceptibility and resistance to leishmania major in mice. Nat Rev Immunol 2002; 2:845-58; http://dx.doi.org/10.1038/nri933
- Kirkegaard K, Taylor MP, Jackson WT. Cellular autophagy: surrender, avoidance and subversion by microorganisms. Nat Rev Micro 2004; 2:301-14; http://dx.doi.org/10.1038/nrmicro865
- Kyei GB, Dinkins C, Davis AS, Roberts E, Singh SB, Dong C, Wu L, Kominami E, Ueno T, Yamamoto A, et al. Autophagy pathway intersects with HIV-1 biosynthesis and regulates viral yields in macrophages. J Cell Biol 2009; 186:255-68; PMID:19635843; http://dx.doi.org/10.1083/jcb.200903070
- Pinheiro RO, Nunes MP, Pinheiro CS, D'Avila H, Bozza PT, Takiya CM, Côrte-Real S, Freire-de-Lima CG, DosReis GA, et al. Induction of autophagy correlates with increased parasite load of Leishmania amazonensis in BALB/c but not C57BL/6 macrophages. Microbes and Infection 2009; 11:181-90; PMID:19070676; http://dx.doi.org/10.1016/j.micinf.2008.11.006
- Kroemer G, Galluzzi L, Vandenabeele P, Abrams J, Alnemri ES, Baehrecke EH, Blagosklonny MV, El-Deiry WS, Golstein P, Green DR, et al. Classification of cell death: recommendations of the Nomenclature Committee on Cell Death 2009. Cell Death Differ 2009; 16:3-11; PMID:18846107; http://dx.doi.org/10.1038/cdd.2008.150
- Zou W, Wang X, Vale RD, Ou G. Autophagy genes promote apoptotic cell corpse clearance. Autophagy 2012; 8:1267-8; PMID:22653037; http://dx.doi.org/10.4161/auto.20786
- Mizushima N, Yoshimori T. How to interpret LC3 immunoblotting. Autophagy 2007; 3:542-5; PMID:17611390
- Bernard A, Klionsky DJ. Autophagosome formation: tracing the source. Dev Cell 2013; 25:116-7; PMID:23639440; http://dx.doi.org/10.1016/j.devcel.2013.04.004
- Röder M, Kleiner K, Sachs A, Keil N, Holzhauser T. Detectability of lupine seeds by ELISA and PCR may be strongly influenced by potential differences between cultivars. J Agric Food Chem 2013; 61:5936-45; PMID:23758099; http://dx.doi.org/10.1021/jf400508a
- Berrizbeitia M, Ndao M, Bubis J, Gottschalk M, Aché A, Lacouture S, Medina M, Ward BJ. Purified excreted-secreted antigens from Trypanosoma cruzi trypomastigotes as tools for diagnosis of Chagas' disease. J Clin Microbiol 2006; 44:291-6; PMID:16455872; http://dx.doi.org/10.1128/JCM.44.2.291-296.2006
- Santarém N, Silvestre R, Tavares J, Silva M, Cabral S, Maciel J, Cordeiro-da-Silva A. Immune response regulation by leishmania secreted and nonsecreted antigens. J Biomed Biotechnol 2007; 2007:85154; PMID:17710243; http://dx.doi.org/10.1155/2007/85154
- Lieke T, Nyln S, Eidsmo L, McMaster WR, Mohammadi AM, Khamesipour A, Berg L, Akuffo H. Leishmania surface protein gp63 binds directly to human natural killer cells and inhibits proliferation. Clin Exp Immunol 2008; 153:221-30; PMID:18713141; http://dx.doi.org/10.1111/j.1365-2249.2008.03687.x
- Stenger S, van Zandbergen G. Measuring the killing of intracellular pathogens: Leishmania. Curr Protoc Immunol 2011; Chapter 14:Unit14.23; PMID:21462165; http://dx.doi.org/10.1002/0471142735.im1423s93
- Enzenmüller S, Gonzalez P, Debatin K, Fulda S. Chloroquine overcomes resistance of lung carcinoma cells to the dual PI3K/mTOR inhibitor PI103 by lysosome-mediated apoptosis. Anticancer Drugs 2013; 24:14-9; PMID:23111416; http://dx.doi.org/10.1097/CAD.0b013e32835a36db
- Huang J, Canadien V, Lam GY, Steinberg BE, Dinauer MC, Magalhaes MA, Glogauer M, Grinstein S, Brumell JH. Activation of antibacterial autophagy by NADPH oxidases. Proc Natl Acad Sci USA 2009; 106:6226-31; PMID:19339495; http://dx.doi.org/10.1073/pnas.0811045106
- Klionsky DJ, Abdalla FC, Abeliovich H, Abraham RT, Acevedo-Arozena A, Adeli K, Agholme L, Agnello M, Agostinis P, Aguirre-Ghiso JA, et al. Guidelines for the use and interpretation of assays for monitoring autophagy. Autophagy 2012; 8:445-544; PMID:22966490
- Sassi A, Larguèche-Darwaz B, Collette A, Six A, Laouini D, Cazenave PA, Dellagi K. Mechanisms of the natural reactivity of lymphocytes from noninfected individuals to membrane-associated Leishmania infantum antigens. J Immunol. 2005; 174:3598-607; PMID:15749897
- Danke NA, Kwok WW. HLA class II-restricted CD4+ T cell responses directed against influenza viral antigens postinfluenza vaccination. The Journal of Immunology2003; 171:3163-9; http://dx.doi.org/10.4049/jimmunol.171.6.3163
- Novak EJ, Masewicz SA, Liu AW, Lernmark A, Kwok WW, Nepom GT. Activated human epitope-specific T cells identified by class II tetramers reside within a CD4high, proliferating subset. Int. Immunol. 2001; 13:799-806; PMID:11369708
- Sauer J, Shannon JG, Howe D, Hayes SF, Swanson MS, Heinzen RA. Specificity of Legionella pneumophila and Coxiella burnetii vacuoles and versatility of Legionella pneumophila revealed by coinfection. Infect Immun 2005; 73:4494-504; PMID:16040960; http://dx.doi.org/10.1128/IAI.73.8.4494-4504.2005
- Simeone R, Bobard A, Lippmann J, Bitter W, Majlessi L, Brosch R, Enninga J. Phagosomal rupture by Mycobacterium tuberculosis results in toxicity and host cell death. PLoS Pathog. 2012; 8:e1002507; PMID:22319448; http://dx.doi.org/10.1371/journal.ppat.1002507
- Gutierrez MG, Colombo MI. Autophagosomes: a fast-food joint for unexpected guests. Autophagy 2005; 1:179-81; PMID:16874034
- Lodge R, Descoteaux A. Phagocytosis of Leishmania donovani amastigotes is Rac1 dependent and occurs in the absence of NADPH oxidase activation. Eur J Immunol 2006; 36:2735-44; PMID:16955522; http://dx.doi.org/10.1002/eji.200636089
- Matheoud D, Moradin N, Bellemare-Pelletier A, Shio MT, Hong WJ, Olivier M, Gagnon E, Desjardins M, Descoteaux A. Leishmania evades host immunity by inhibiting antigen crosspresentation through direct cleavage of the SNARE VAMP8. Cell Host & Microbe 2013; 14:15-25; http://dx.doi.org/10.1016/j.chom.2013.06.003
- Wanderley J L, Barcinski MA. Apoptosis and apoptotic mimicry: the Leishmania connection. Cell Mol Life Sci 2010; 67:1653-9; PMID:20140747; http://dx.doi.org/10.1007/s00018-010-0291-0
- El-Hani CN, Borges VM, Wanderley JL, Barcinski MA. Apoptosis and apoptotic mimicry in Leishmania: an evolutionary perspective. Front Cell Infect Microbiol 2012; 2:96; PMID:22912937; http://dx.doi.org/10.3389/fcimb.2012.00096
- Zangger H, Mottram JC, Fasel N. Cell death in Leishmania induced by stress and differentiation: programmed cell death or necrosis? Cell Death Differ 2002; 9:1126-39; PMID:12232801; http://dx.doi.org/10.1038/sj.cdd.4401071
- Kessler RL, Soares MJ, Probst CM, Krieger MA. Trypanosoma cruzi response to sterol biosynthesis inhibitors: morphophysiological alterations leading to cell death. PLoS One 2013; 8:e55497; PMID:23383204; http://dx.doi.org/10.1371/journal.pone.0055497
- Shapiro TA, Englund PT. The structure and replication of kinetoplast DNA. Annu Rev Microbiol 1995; 49:117-43; PMID:8561456; http://dx.doi.org/10.1146/annurev.mi.49.100195.001001
- Ruhland A, Leal N, Kima PE. Leishmania promastigotes activate PI3K/Akt signalling to confer host cell resistance to apoptosis. Cell Microbiol 2007; 9:84-96; PMID:16889626; http://dx.doi.org/10.1111/j.1462-5822.2006.00769.x
- Sanjuan MA, Dillon CP, Tait SW, Moshiach S, Dorsey F, Connell S, Komatsu M, Tanaka K, Cleveland JL, Withoff S, Green DR. Toll-like receptor signalling in macrophages links the autophagy pathway to phagocytosis. Nature 2007; 450:1253-7; http://dx.doi.org/10.1038/nature06421
- Mintern JD, Villadangos JA. Autophagy and mechanisms of effective immunity. Front Immun 2012; 3:60; http://dx.doi.org/10.3389/fimmu.2012.00060
- Lam GY, Cemma M, Muise AM, Higgins DE, Brumell JH. Host and bacterial factors that regulate LC3 recruitment to Listeria monocytogenes during the early stages of macrophage infection. Autophagy 2013; 9:985-95; PMID:23584039; http://dx.doi.org/10.4161/auto.24406
- Hocès de la Guardia A, Staedel C, Kaafarany I, Clément A, Roubaud Baudron C, Mégraud F, Lehours P. Inflammatory cytokine and microRNA responses of primary human dendritic cells cultured with Helicobacter pylori strains. Front Microbiol 2013; 4:236; PMID:23970881; http://dx.doi.org/10.3389/fmicb.2013.00236
- Tiemessen MM, Kunzmann S, Schmidt-Weber CB, Garssen J, Bruijnzeel-Koomen, Carla A, Knol EF, van Hoffen E. Transforming growth factor-beta inhibits human antigen-specific CD4+ T cell proliferation without modulating the cytokine response. Int Immunol 2003; 15:1495-504; PMID:14645158
- Klein L, Münz C, Lünemann JD. Autophagy-mediated antigen processing in CD4(+) T cell tolerance and immunity. FEBS Lett 2010; 584:1405-10; PMID:20074571; http://dx.doi.org/10.1016/j.febslet.2010.01.008
- Romano PS, Arboit MA, Vázquez CL, Colombo MI. The autophagic pathway is a key component in the lysosomal dependent entry of Trypanosoma cruzi into the host cell. Autophagy 2009; 5:6-18; PMID:19115481
- Liu J, Xia H, Kim M, Xu L, Li Y, Zhang L, Cai Y, Norberg HV, Zhang T, Furuya T, et al. Beclin1 controls the levels of p53 by regulating the deubiquitination activity of USP10 and USP13. Cell 2011; 147:223-34; PMID:21962518; http://dx.doi.org/10.1016/j.cell.2011.08.037
- Rubinsztein DC, Codogno P, Levine B. Autophagy modulation as a potential therapeutic target for diverse diseases. Nat Rev Drug Discov 2012; 11:709-30; PMID:22935804; http://dx.doi.org/10.1038/nrd3802
- Martinez J, Almendinger J, Oberst A, Ness R, Dillon CP, Fitzgerald P, Hengartner MO, Green DR. Microtubule-associated protein 1 light chain 3 alpha (LC3)-associated phagocytosis is required for the efficient clearance of dead cells. Proc Natl Acad Sci USA 2011; 108:17396-401; http://dx.doi.org/10.1073/pnas.1113421108
- Vernon PJ, Tang D. Eat-me: autophagy, phagocytosis, and reactive oxygen species signaling. Antioxid. Redox Signal. 2013; 18:677-91; http://dx.doi.org/10.1089/ars.2012.4810
- Neu C, Sedlag A, Bayer C, Förster S, Crauwels P, Niess J, van Zandbergen G, Frascaroli G, Riedel CU. CD14-dependent monocyte isolation enhances phagocytosis of listeria monocytogenes by proinflammatory, GM-CSF-derived macrophages. PLoS ONE 2013; 8:e66898; PMID:23776701; http://dx.doi.org/10.1371/journal.pone.0066898
- Wenzel UA, Bank E, Florian C, Förster S, Zimara N, Steinacker J, Klinger M, Reiling N, Ritter U, van Zandbergen G. Leishmania major parasite stage-dependent host cell invasion and immune evasion. FASEB J. 2012; 26:29-39; PMID:21908716; http://dx.doi.org/10.1096/fj.11-184895
- Buser C, Walther P. Freeze-substitution: the addition of water to polar solvents enhances the retention of structure and acts at temperatures around -60 degrees C. J Microsc 2008; 230:268-77; PMID:18445157; http://dx.doi.org/10.1111/j.1365-2818.2008.01984.x
- Walther P, Ziegler A. Freeze substitution of high-pressure frozen samples: the visibility of biological membranes is improved when the substitution medium contains water. J Microsc 2002; 208:3-10; PMID:12366592
- Bastian M, Braun T, Bruns H, Röllinghoff M, Stenger S. Mycobacterial lipopeptides elicit CD4+ CTLs in Mycobacterium tuberculosis-infected humans. J Immunol. 2008; 180:3436-46; PMID:18292570
- Wheeler RJ, Gluenz E, Gull K. The cell cycle of Leishmania: morphogenetic events and their implications for parasite biology. Mol Microbiol 2011; 79:647-62; http://dx.doi.org/10.1111/j.1365-2958.2010.07479.x
- Roederer M. Interpretation of cellular proliferation data: avoid the panglossian. Cytometry A 2011; 79:95-101; PMID:21265003; http://dx.doi.org/10.1002/cyto.a.21010