Abstract
Deficient autophagy causes a distinct phenotype in Dictyostelium discoideum, characterized by the formation of multitips at the mound stage. This led us to analyze autophagy in a number of multitipped mutants described previously (tipA−, tipB−, tipC−, and tipD−). We found a clear autophagic dysfunction in tipC− and tipD− while the others showed no defects. tipD codes for a homolog of Atg16, which confirms the role of this protein in Dictyostelium autophagy and validates our approach. The tipC-encoded protein is highly similar to human VPS13A (also known as chorein), whose mutations cause the chorea-acanthocytosis syndrome. No member of the VPS13 protein family has been previously related to autophagy despite the presence of a region of similarity to Atg2 at the C terminus. This region also contains the conserved domain of unknown function DUF1162. Of interest, the expression of the TipC C-terminal coding sequence containing these 2 motifs largely complemented the mutant phenotype. Dictyostelium cells lacking TipC displayed a reduced number of autophagosomes visualized with the markers GFP-Atg18 and GFP-Atg8 and an impaired autophagic degradation as determined by a proteolytic cleavage assay. Downregulation of human VPS13A in HeLa cells by RNA interference confirmed the participation of the human protein in autophagy. VPS13A-depleted cells showed accumulation of autophagic markers and impaired autophagic flux.
Abbreviations:
- ATG, autophagy related
- AX4, axenic strain 4
- DUF, domain of unknown function
- GFP, green fluorescent protein
- LC3, microtubule-associated protein 1 light chain 3
- PtdIns3K, phosphatidylinositol 3-kinase
- PtdIns3P, phosphatidylinositol 3-phosphate
- VPS13, vacuolar protein sorting 13 homolog (S. cerevisiae)
- WIPI, WD repeat domain, phosphoinoside interacting
Introduction
Dictyostelium discoideum is a social amoeba whose developmental process depends on macroautophagy (referred to as ‘autophagy’ hereafter for simplicity). This process is a highly conserved lysosomal degradation pathway and it is the main cellular mechanism for protein and organelle degradation in eukaryotes.Citation1,2 It is characterized by the formation of double-membrane vesicles, the autophagosomes, which engulf a variety of cargos including portions of the cytoplasm, protein aggregates or damaged organelles. The autophagosomes eventually fuse with lysosomes, where the cargo is degraded and simple biochemical compounds are released for recycling or energy production. At the molecular level, autophagy is controlled by different functional complexes, which are required for the origin, elongation, and maturation of the autophagosomes.Citation3 The hierarchical relationship among autophagic proteins allows a tight regulation of the process. At the initial step, the inductive complexes containing the serine/threonine kinase ULK1/Atg1 (unc-51 like autophagy activating kinase 1) and the class III phosphatidylinositol 3-kinase (PtdIns3K) PIK3C3/Vps34 (phosphatidylinositol 3-kinase, catalytic subunit type 3/vacuolar protein sorting 34) are recruited to specific sites of the endoplasmic reticulum and provide a platform for autophagosome biogenesis, the so-called omegasome.Citation4 The activity of PtdIns3K generates a PtdIns3P-enriched region to which the complex formed by Atg2 and WIPI/Atg18 (WD repeat domain, phosphoinoside interacting) is recruited. Later, the ATG12–ATG5-ATG16L1 complex and the lipidation of LC3/Atg8 (microtubule-associated protein 1 light chain 3) to the emerging membrane allow the elongation of the phagophore.Citation5
A number of studies in Dictyostelium have revealed the similarities, both morphological and molecular, of its autophagic process with that of animal cells.Citation6 In addition, this organism possesses several autophagic proteins that are conserved in mammals but absent in S. cerevisiae such as ATG101 and VMP1.Citation2,6,7 Dictyostelium mutant strains lacking Atg proteins display deficient autophagy and developmental abnormalities.Citation8-11 The most common phenotype is the block of development at the mound stage and the formation of multiple tips instead of normal fruiting bodies. This feature can be used as a screening parameter that might help to identify new genes involved in autophagy. Four multitipped mutants were described in the laboratory of William F. Loomis in 1999 and the proteins affected are named TipA, TipB, TipC, and TipD (DictyBase Gene IDs: DDB_G0281561, DDB_G0276333, DDB_G0267422, DDB_G0275323).Citation12,13 The 4 tip genes are expressed during vegetative growth and throughout development and have parallel but yet unknown functions during Dictyostelium development.Citation13 Sequence analysis reveals that tipC codes for a protein highly similar to the conserved VPS13 family. In humans, a member of this family, VPS13A, is the protein mutated in chorea-acanthocytosis.
Chorea-acanthocytosis (ChAc) (OMIM ID: 200150) is a rare autosomal recessive neurodegenerative disease. The most relevant symptoms are involuntary tensing of muscles (especially those in the face, mouth, and limbs), neurodegeneration, and erythrocyte acanthocytosis.Citation14-16 This disorder is caused by the loss of function of the 360-kDa protein VPS13A (also known as chorein), which is nearly absent in patients.Citation17-21 A mouse model of the disease has been reported.Citation22 It shows similarities to the human syndrome, such as brain pathology and red blood acanthocytosis, but displays a mild phenotype with late old-age onset.Citation22 In humans, VPS13A is expressed in a wide variety of tissues.Citation23 Defects in actin cytoskeleton regulation have been described in erythrocytes, platelets, and vascular endothelial cells in the absence of VPS13A.Citation24,25 In simpler organisms, null mutants in Saccharomyces cerevisiae and Tetrahymena thermophila suggest a role of Vps13 and VPS13A in membrane traffic and phagocytosis respectively but the precise VPS13 function is largely unknown.Citation26-28
Here we describe how the VPS13-related protein TipC is required for efficient autophagy in D. discoideum. We extended our observations to human VPS13A by analyzing autophagy in RNA-interfered HeLa cells. Our results confirmed the role of VPS13A in autophagy and suggest the possibility that autophagy is involved in the etiology of chorea-acanthocytosis as described previously for other neurodegenerative diseases such as Parkinson, Alzheimer, and Huntington diseases.Citation29 This is relevant because, at present, no effective therapy for chorea-acanthocytosis is available and there is a growing interest of medical research in modulating autophagy as a therapeutic treatment.Citation29-31
Results
Screening for autophagy defects in Dictyostelium multitipped mutants
Dictyostelium strains carrying gene disruptions in tipA, tipB, tipC, or tipD showed a similar phenotype in which large mounds split up to form multiple tips.Citation13 The similarity of this phenotype to the one observed in autophagy-deficient strainsCitation2 led us to analyze autophagy in these mutants. To this end, autophagic flux was measured by a proteolytic cleavage assay described previously.Citation32 A large decrease in autophagic flux was detected in tipC− and tipD− strains () while tipA− and tipB− mutants showed similar levels than those of the wild type ().
Figure 1. Autophagic flux is decreased in tipC− and tipD− cells. Proteolytic cleavage assay was performed in strains transfected with the marker GFP-Tkt-1. Protein extracts were analyzed by western blot using anti-GFP antibody. (A) The accumulation of cleaved GFP fragment (black arrows) in the presence of 100 mM NH4Cl is reduced in tipC− and tipD− mutants compared to the wild-type AX4 strain. (B) tipA− and tipB− levels of cleaved GFP are similar to those of AX4. The complete GFP-Tkt-1fusion protein is marked by white arrows and the stars indicate nonspecific immunoreactive bands.
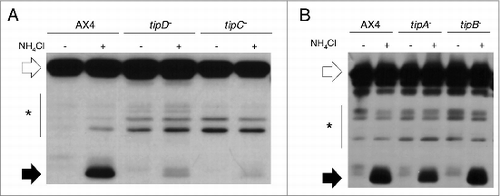
Analysis of the predicted protein sequences indicates that tipD (accession number EAL69727.1) codes for a protein similar to the yeast Atg16 and human ATG16L1. Of interest, the similarity of TipD to the homologous human protein is much higher (E-value: 2.2e-59) than it is to the yeast protein (E-value: 3.8e-4; E-values calculated with the LALIGN program from Biology WorkBench, http://workbench.sdsc.edu/) and the sequence also contains a large conserved WD-repeat region at the C terminus that is present in the human protein but absent in the yeast ortholog (Fig. S1). This domain might be involved in protein-protein interactions that have been conserved during evolution between Dictyostelium and mammalian cells. The identification of the Dictyostelium Atg16 ortholog and the confirmation of its role in autophagy validate our approach for the screening of new autophagic proteins using the mutitipped phenotypic feature.
tipC (EAL73163.1) encodes a large protein of 3848 amino acids highly similar to the VPS13 family of proteins (Fig. S2). The human VPS13 family has 4 members (VPS13A/B/C/D) and TipC shows the highest homology with VPS13A and VPS13C (Fig. S3). No previous connection with autophagy has been reported for any of these proteins. Since mutations in human VPS13A leads to chorea-acanthocytosis, a rare disease that causes neurodegeneration, we focused on the study of Dictyostelium TipC and human VPS13A.
The conserved C terminus of TipC is able to function independently to largely complement the mutant phenotype
TipC and VPS13A show 3 conserved regions at the N terminus and C terminus (). Interestingly, the C terminus contains a small sequence similar to the C-terminal region of Atg2, as detected by the NCBI Conserved Domain Search using the Pfam database v27.0 (). This motif is depicted as ATG_C-terminal domain and it is detected in both Dictyostelium TipC and human VPS13A with a significant E-value (2.42e-6 and 2.59e-6 respectively). The same region is detected in the yeast protein, but with a much less significant score (E-value: 0.29). The C-terminal region also contains a conserved domain of unknown function DUF1162. In order to assess the functionality of the Tip C-terminal region, we cloned and expressed the coding sequence fused to GFP in the tipC− mutant strain. The expression of the C-terminal polypeptide was confirmed by western blot using an anti-GFP antibody () and by confocal analysis of GFP fluorescence, which showed a cytoplasmic localization (). Remarkably, the expression of the fragment largely complemented the mutant developmental phenotype (). The complemented strain no longer displayed the characteristic multitipped phenotype. It succeeded to pass the mound stage and form normal-looking fruiting bodies. Calcofluor staining, which labels the cellulose walls of stalk and spore cells, showed that the few stalks present in the original mutant were abnormal. The complemented strain, however, showed fairly differentiated stalks (). Nevertheless, the recovery was not complete because some of the structures were smaller and had thick stalks. Spore differentiation was also complemented according to the ellipsoid morphology of spores that were stained with calcofluor () and the number and viability of the formed spores. Spore efficiency was quantified in comparison with the wild-type AX4 strain and it recovered from 1.70 ± 0.03% in the original mutant to 56.94 ± 0.20% in the complemented strain. All together, the full-length protein must be required for a total recovery, but the C-terminal region can function independently to some extent during development progression and stalk differentiation. Autophagy is required for these processes, and thus, the conserved C terminus of TipC probably has a critical function in autophagy.
Figure 2 (See previous page). The conserved C terminus of TipC largely complements the tipC− phenotype. (A) Scheme of the 3848-amino acid TipC protein and the conserved domains (colored boxes). The line under the scheme depicts the fragment expressed in the complementation experiments. Alignment of the N-terminal (B) and C-terminal (C) regions of D. discoideum TipC (EAL73163.1) and the human VPS13A (NP_150648.2) proteins using the ClustalW algorithm and shaded using the Boxshade tool at the SDSC Biology WorkBench server (http://workbench.sdsc.edu/). Identical residues are shaded black, and similar residues are shaded gray. Colored boxes frame the conserved domains. (D) Western blot using anti-GFP antibody confirmed the presence of a unique band of 150 kDa in the transformed strain with the C-terminal region of TipC (amino acids 2725 to 3848) fused to GFP. Lanes 1 and 3 correspond to protein extracts of AX4 and tipC− that do not express the fused proteins and were used as controls of antibody specificity. (E) The C-terminal TipC polypeptide fused to GFP is localized in the cytoplasm according to in vivo confocal microscopy visualization of transformed cells. (F) The complemented strain rescues fruiting body development. Scale bar: 10 μm. (G) Calcofluor staining shows details of stalk differentiation and spore shape of the different strains. For tipC− mainly cell debris could be visualized. Scale bar: 10 μm. Spore efficiency of each strain (sp. eff.) is indicated. (H) Phagocytosis rate of the wild-type, the mutant, and the complemented strain was measured through the internalized fluorescent signal of cells that were previously incubated with fluorescent beads during the indicated times.
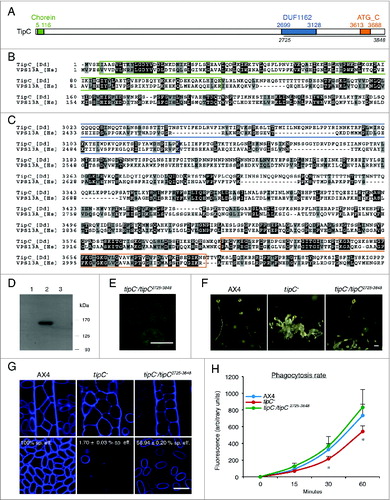
Since autophagy and phagocytosis have been described as connected Citation33,34 and Tetrahymena thermophila VPS13A is required for efficient phagocytosis,Citation27 we have also analyzed this process in the AX4, tipC− and the complemented strains. The results indicated a modest defect in phagocytosis in the mutant that was clearly recovered in the complemented strain ().
TipC and TipD regulate autophagy at different stages of autophagosome formation
To further characterize the role of TipC and TipD in autophagy and to determine which stage of autophagosome formation is affected, we expressed the autophagic markers GFP-Atg8 and GFP-Atg18 in tipC− and tipD− strains and visualized them by confocal microscopy. The number and the pattern of GFP-Atg8 puncta were altered in both strains. tipC− cells showed a reduced number of puncta and also some abnormally large structures absent in the wild-type strain. In the case of tipD− cells, they showed a much higher number of large structures, which appeared to be protein aggregates (). GFP-Atg8 is an aggregate-prone marker and previous studies in Dictyostelium have shown a similar pattern in mutants of the autophagic proteins Atg1, Atg7, and Vmp1,Citation7,10 with the presence of large ubiquitinated protein aggregates containing GFP-Atg8. Therefore, we analyzed the presence of ubiquitin-positive aggregates by immunofluorescence. Both mutant strains contained ubiquitinated aggregates although they were larger in tipD− cells, where the colocalization with GFP-Atg8 was also more evident and demonstrates that these large structures are not real autophagosomes (). These abnormal patterns and the presence of ubiquitinated aggregates are consistent with the observed block in autophagy flux.
Figure 3. The pattern of GFP-Atg8 is altered in tipC− and tipD− cells. (A) In vivo confocal analysis of cells expressing GFP-Atg8 in growing and starvation conditions. Puncta formation is inhibited in starved tipC− cells compared with AX4. In contrast, large aggregates of the autophagosome marker are evident in tipD− cells in both growing conditions. (B) Immunofluorescence of ubiquitin in the cells expressing GFP-Atg8 demonstrates the presence of ubiquitin-positive protein aggregates in both mutants. The ubiquitin structures are smaller in tipC− cells and do not always colocalize with GFP-Atg8, while the autophagosome marker is clearly contained in the large tipD− ubiquitinated aggregates. Scale bar: 10 μm.
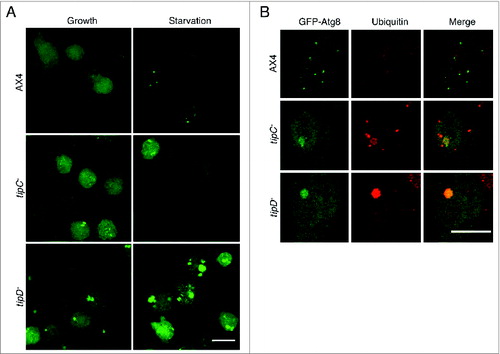
Due to the GFP-Atg8 tendency to aggregate, we used another marker, GFP-Atg18, for quantitative analysis of puncta (). GFP-Atg18 labels the initial stages of autophagosome formation and the puncta pattern is thinner than that of the GFP-Atg8. tipC−, and tipD− cells showed a distinct GFP-Atg18 pattern. Puncta were accumulated in tipD− cells under growth and starvation conditions compared with the wild type. Since GFP-Atg18 is recruited to PtdIns3P-enriched endoplasmic reticulum-derived omegasomes,Citation35 this accumulation suggests that autophagosome formation is impaired at a stage posterior to the PdtIns3P signaling. This is consistent with the described role of Atg16 in the stage of autophagosome membrane elongation, downstream of Atg1 and PtdIns3K in the functional hierarchy.Citation5 Conversely, tipC− cells showed a significantly reduced number of GFP-Atg18 puncta. This is in agreement with an early blockade in autophagy, as observed in other Dictyostelium mutants lacking components that participate at the initial inductive signaling, such as the null mutant in Atg1.Citation7
Figure 4. GFP-Atg18 puncta signaling is decreased in tipC− cells and accumulates in tipD− cells. (A) Cells expressing GFP-Atg18 were analyzed in vivo by confocal microscopy. Puncta formation is induced under starvation conditions in AX4 cells. However, most of tipC− cells do not display any puncta. Conversely, many puncta are observed tipD− cells regardless of the nutritional conditions. Scale bar: 10 μm. (B) Quantification of GFP-Atg18 puncta of at least 100 cells in blinded images from 3 independent experiments. Results are shown as mean values with standard deviations of the percentage of cells showing the indicated number of puncta. Asterisks indicate the level of significance of the Student t test (* < 0.05; ** < 0.01).
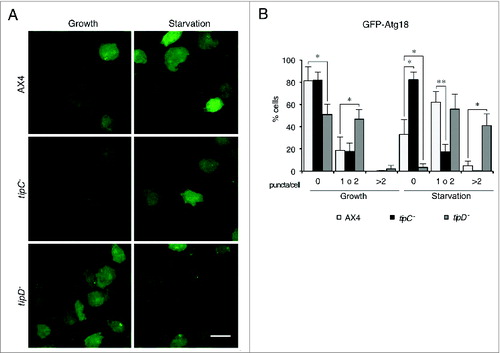
VPS13A depletion in human HeLa cells alters the pattern of autophagosome markers
We next studied the consequences of VPS13A depletion in human HeLa cells in order to determine if the role in autophagy is conserved. Cells were transfected with control or VPS13A siRNAs and the GFP-LC3 and GFP-WIPI1 pattern were observed in growth and starvation. Inhibition of VPS13A expression was determined by qRT-PCR. The VPS13A mRNA levels were reduced by 89.5 ± 2.9% compared to the levels in control-transfected cells. Depletion of VPS13A protein was also determined by western blot (). In HeLa cells stably expressing GFP-LC3, a high proportion of VPS13A-depleted cells showed an accumulation of puncta at the perinuclear region regardless of the nutritional conditions. In starvation, besides the perinuclear accumulation, the VPS13A siRNA-treated cells showed less translocation of the marker from the nucleus to the cytoplasm as compared with the control-transfected cells (). Puncta quantification shows that VPS13A-silenced cells had an increase of puncta in growth and this number did not increase during starvation as did occur in the control cells (). In HeLa VPS13A-depleted cells transiently transfected with the GFP-WIPI1 marker, there were more GFP-WIPI1 puncta than in control cells in both conditions, and there was not a significant increase following starvation (). These results suggest that the accumulation of puncta might be the result of a deficiency in autophagic flux.
Figure 5. VPS13A downregulation causes GFP-LC3 and GFP-WIPI1 accumulation. (A) Western blot of control and VPS13A siRNA-transfected cells. A band of the VPS13A-predicted molecular weight decreases in the VPS13A-silenced cells. The asterisks indicate nonspecific immunoreactive bands. (B and C) HeLa cells stably expressing GFP-LC3 were transfected with control or VPS13A siRNAs and the pattern observed by confocal microscopy. VPS13A-depleted cells show accumulated puncta and less translocation from the nucleus to the cytoplasm during starvation. (D and E) HeLa cells were transfected with GFP-WIPI1 and the pattern observed by confocal microscopy. The number of puncta is higher in VPS13A siRNA-treated cells and does not significantly increase following starvation. The graphs show the mean and the standard deviation of puncta quantification of more than 40 cells from 3 independent experiments. Asterisks indicate the level of significance of the Student t test (* < 0.05; ** < 0.01). Scale bar: 10 μm.
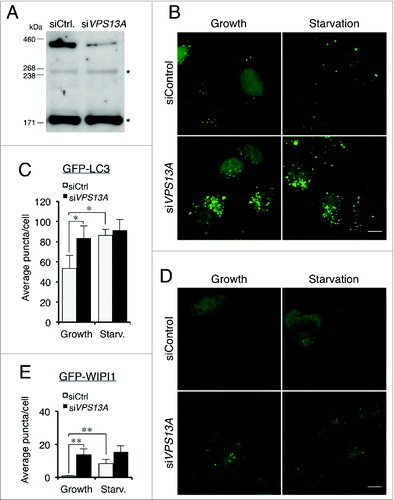
VPS13A is required for autophagic flux
In order to analyze autophagic flux, siRNA-treated HeLa cells stably expressing GFP-LC3 were incubated in complete or starvation medium in the presence or absence of the lysosome inhibitor chloroquine. Protein extracts of at least 3 independent experiments were analyzed by western blot to detect GFP-LC3, cleaved GFP, and endogenous LC3-I/-II levels (). Since GFP-LC3 is degraded by autophagy, the level of GFP-LC3 has been previously shown to decrease during starvation.Citation36-38 As expected, this was the case for the control cells; however, high levels of the marker were present in the VPS13A siRNA-treated cells irrespective of the nutritional conditions (). Another marker of autophagic flux is the cleaved GFP band, which is generated in part by autophagic degradation, as demonstrated by the GFP accumulation in chloroquine-treated cells. Once more, cleaved GFP accumulation was evident, together with a smaller increase in the presence of chloroquine in starved VPS13A-depleted cells (). These experiments indicate a lower autophagic flux during starvation.
Figure 6. A reduced autophagic flux is observed in VPS13A siRNA-treated cells. (A) GFP-LC3, free GFP, and endogenous LC3-I/-II were analyzed by western blot from control or VPS13A siRNA-treated cells under growth or starvation conditions with or without 5 μM chloroquine (CQ). (B–E) Densitometric quantification showing the mean and the standard deviations of at least 3 independent experiments. The data was normalized against GAPDH. Asterisks indicate the level of significance of the Student t test (* < 0.05; ** < 0.01; *** < 0.001).
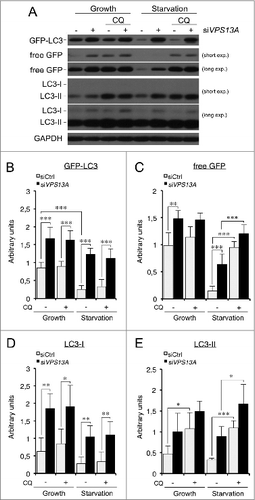
The levels and turnover of endogenous LC3-I/-II were also quantified. Of note, the amount of LC3-I (the cytosolic form) in VPS13A-depleted cells was higher than in control cells, suggesting that conversion of LC3-I to LC3-II was affected (). LC3 turnover was also consistent with a partial block of autophagic flux as the difference in the LC3-II levels between cells treated with or without chloroquine was reduced in VPS13A-silenced cells in both conditions (). All together, these results indicate that VPS13A is required for efficient autophagy at basal and starvation conditions in human HeLa cells.
Discussion
We have characterized the role of TipC and TipD in Dictyostelium autophagy. TipD encodes a homolog of the S. cerevisiae Atg16 but shows a higher similarity to the human ATG16L1 because it contains a large C-terminal region that is not present in the yeast model. This is another example of the close similarity of the autophagy machinery between Dictyostelium and animal cells.Citation2
Dictyostelium TipC and human VPS13A belong to the VPS13 family. To our knowledge, this is the first description of the role of this protein family in autophagy. Dictyostelium genome encodes other 5 Vps13 proteins, which have not been characterized yet. Similarly, the human genome also codes for several members of this family (VPS13A/B/C/D). Two of them, VPS13A and VPS13B, are responsible for the diseases chorea-acanthocytosis and Cohen syndrome, respectively. These diseases differ in many phenotypic and pathological aspects so it is likely that these proteins have diverged in their function. In addition, the analysis of amino acid identity shows that VPS13A and VPS13C are more similar, while VPS13B is more distantly related. The Dictyostelium TipC shows higher similarity to human VPS13A and VPS13C than to VPS13B. Interestingly, the expression of the C-terminal region of TipC allows the strain to progress in development, pass the finger stage, and form differentiated spores and stalks, a process dependent on autophagy. These results suggest that the C-terminal region is important for autophagy regulation. This region contains 2 conserved domains also present in the human VPS13 proteins (DUF1162, and ATG_C). The first one is a domain of unknown function, and the second one is a short region of similarity with Atg2, a protein that is recruited together with WIPI1/Atg18 to the sites of autophagosome formation.
The function of VPS13 proteins has been addressed in other model systems like the yeast Saccharomyces cerevisiae and the protozoa Tetrahymena thermophila. Interestingly, the Tetrahymena VPS13A localizes to the phagosome membrane and it is required for efficient phagocytosis.Citation27 A relationship between autophagy and phagocytosis pathways has been described in mammalian phagocytes and this connection seems to be required for efficient killing and digestion of extracellular pathogens.Citation34 The phagocytosis defect observed in Dictyostelium tipC− is not profound and could be due to a side effect of the autophagy blockade.
Yeast has only one Vps13 protein, which has been involved in membrane morphogenesis during sporulation but no defects in autophagy have been reported in the mutant strain so far. More specifically, the yeast vps13Δ cells show a depletion of PtdIns4P levels in prospore membranes.Citation26,39 Distinct PtdIns-phosphates play essential roles in autophagy at different levels. For example, PtdIns(3,4,5)P3 is essential for the signaling pathway that regulates TORC1 (TOR complex 1), and PtdIns(4,5)P2 is required for the formation of autophagosomes originated from the plasma membrane.Citation40 Another important PtdIns molecule is PtdIns3P, which is formed at the ER-derived omegasome by the action of the class III PtdIns3K/Vps34. This signaling lipid is required for the recruitment of Atg18 and Atg2 to the phagophore site. Our results in Dictyostelium show a lower recruitment of the marker GFP-Atg18 in cells lacking TipC, which might indicate a defect at the level of PdIns3P signaling. However, VPS13A-depleted HeLa cells, although impaired in autophagy flux, show the accumulation of autophagic markers, including WIPI1, the counterpart of Atg18. These results suggest that Dictyostelium TipC and human VPS13A regulate autophagy at different stages. Due to the large number of members of the VPS13 family in Dictyostelium and humans, we cannot be certain if the Dictyostelium TipC is the precise functional counterpart of human VPS13A. It is likely that the different members could regulate autophagy at different levels or even distinct but related processes, an issue that will require the complete characterization of the different proteins of the family in both species.
Previous studies in human cells report that silencing VPS13A in K562-erythrocytic cells and vascular endothelial cells leads to changes in cell shape and cytoskeletal architecture.Citation24,25 It has been suggested that VPS13A-depleted cells have lower activity of class I PI3K at the plasma membrane and this affects the control of actin cytoskeleton.Citation25 Class I PI3K inhibits autophagy through activation of TORC1, thus it is possible that VPS13A-depleted cells have a higher activation of the inductive stages of autophagy. However, even if this occurs, the concomitant accumulation of autophagic markers and the reduction in autophagic flux indicate that autophagosome maturation is impaired in VPS13A-silenced cells.
Malfunction of autophagy is associated with a variety of human diseases, including the most prominent neurodegenerative diseases,Citation41 and therefore, an increasing number of autophagy-modulating compounds are being tested in clinical trials.Citation29 The role of TipC and VPS13A in autophagy opens a new perspective in the functional studies of this protein family and warrants further explorations on the involvement of autophagy in VPS13-related diseases, and more specifically, in chorea-acanthocytosis.
Materials and Methods
Dictyostelium cell culture and genetic manipulations
Dictyostelium Tip mutants were obtained from Dicty-stock center (kindly deposited by William F. Loomis). The parent strain, AX4, was used as wild type. Cells were grown axenically in complete medium (HL-5 or SIH medium; Formedium, HLB0102; SIH0102), or in association with Klebsiella planticola on SM-agar plates. Transformations were carried out by electroporation as described previously.Citation42 For synchronous development, axenically grown cells were washed from culture media by centrifugation, resuspended in PDF buffer (20 mM KCl, 9 mM K2HPO4, 13 mM KH2PO4, 1 mM CaCl2, 1 mM MgSO4, pH 6.4) and deposited on nitrocellulose filters (Millipore, HABP04700). The C-terminal region of TipC was amplified by PCR from genomic DNA and cloned into the pCGFPCTAP vector (fused to GFP at the C terminus).
Calcofluor staining, sporulation, and phagocytosis assays
Calcofluor (Sigma, F3543) staining and sporulation assays were performed as described previously.Citation43 Sporulation efficiency was calculated by taking into account the spore production and the viability of the formed spores and given as a percentage related to the result of wild-type AX4 strain. For the phagocytosis assay, vegetative-grown cells were incubated with fluorescent latex beads (Fluotesbrite 1.0 Micron Microspheres; Polysciences, 18860) and treated as indicated previously.Citation44 The amount of internalized fluorescence was measured in a fluorimeter and corrected by protein concentration in each sample.
HeLa cell culture and small interfering (siRNA) experiments
HeLa cells stably expressing GFP-LC3 were kindly provided by Aviva M. Tolkovsky (John Van Geest Center for Brain Repair, Cambridge, UK) and described previously.Citation45 Cells were maintained in complete medium (DMEM, Dulbecco's modified Eagle's medium). Knockdown was performed by transfection of VPS13A siRNA (Ambion, s23340) or control siRNA (Ambion, control#2) with Lipofectamine RNAiMAX (Invitrogen, 13778-150), according to the manufacturer's instructions. Cells were transfected again with the same siRNAs after 2 d from the first transfection and the assays were carried out 3 d later. The level of depletion of VPS13A mRNA was measured by TaqMan PCR (Applied Biosystems, Hs00362891_m1) and found to be 89.5 ± 2.9% for all the experiments shown. GFP-WIPI-1 transfections were performed using Lipofectamine 2000 (Invitrogen, 11668-019) according to the manufacturer's instructions and the plasmid pMX-IP GFP-WIPI-1 (Addgene, 38272).
Autophagy detection in Dictyostelium
In vivo confocal analysis of autophagic markers GFP-Atg8 and GFP-Atg18, immunofluorescence of ubiquitin aggregates, and proteolytic cleavage assay were performed as described previously.Citation10,32,46 For starvation assays, complete medium was replaced with PDF buffer or SIH medium without arginine and lysine (Formedium, SIH1001) 1 h prior to visualization.
Autophagy detection in HeLa cells
For confocal visualization, cells were incubated in complete medium (DMEM, Dulbecco's modified Eagle's medium) or starvation medium (EBSS, Earle's balanced salt solution) for 2 h, fixed with 4% paraformaldehyde and mount with ProLongGold (Invitrogen, P36934) for visualization. For western blot analysis, total cell lysates were isolated using RIPA buffer from cells incubated in complete or starvation medium for 4 h in the presence or absence of 5 μM chloroquine.
Western blot and confocal microscopy
Immunoblots were performed using anti-GFP (Sigma-Aldrich, G1544), anti-LC3B (Cell Signaling Technology, 2775), anti-GAPDH (Enzo LifeSciences, ADI-CSA-335) and anti-VPS13A (Santa Cruz Biotechnology, sc-109138) antibodies and the appropriate HRP-conjugated secondary antibody (Santa Cruz Biotechnology, sc-2004; sc2005). VPS13A detection was performed as previously described.Citation21 The western blot bands were quantified by densitometry (ImageJ software). Confocal images were acquired using an inverted Zeiss LSM 710 laser-scanning microscope (Carl-Zeiss-Strasse 22, 73447 Oberkochen, Germany). Puncta were counted using ImageJ software.
Statistical analysis
Data were shown as mean with SD (standard deviation) and analyzed using the Student t test. A P value < 0.05, P < 0.01 or P < 0.001 was denoted by *, **, or ***, respectively.
Supplemental data
Supplemental data for this article can be accessed on the publisher's website.
1034413_Supplemental_files.zip
Download Zip (3.6 MB)Acknowledgments
Sequence data for Dictyostelium were obtained from the Genome Sequencing Centers of the University of Cologne, Germany; the Institute of Molecular Biotechnology, Department of Genome Analysis, Jena; the Baylor College of Medicine in Houston, Texas, USA; and the Sanger Center in Hinxton, Cambridge, UK. Tip-deficient strains were obtained from Dicty Stock Center and deposited by William F. Loomis. We thank Patricia Boya and Aviva Tolkovsky for providing the HeLa cells stably expressing GFP-LC3 and M. J. Obregón for providing the quantitative PCR instrument.
Funding
This work was supported by grants BFU2009–09050 and BFU2012–32536 from the Spanish Ministerio de Ciencia e Innovación. We also thank the help of Obra Social Caja de Burgos para Jóvenes Excelentes. SMB is recipient of a predoctoral fellowship from Universidad Autónoma de Madrid.
References
- Yang Z, Klionsky DJ. Mammalian autophagy: core molecular machinery and signaling regulation. Curr Opin Cell Biol 2010; 22: 124-31; PMID:20034776; http://dx.doi.org/10.1016/j.ceb.2009.11.014
- Calvo-Garrido J, Carilla-Latorre S, Kubohara Y, Santos-Rodrigo N, Mesquita A, Soldati T, Golstein P, Escalante R. Autophagy in Dictyostelium: genes and pathways, cell death and infection. Autophagy 2010; 6: 686-701; PMID:20603609; http://dx.doi.org/10.4161/auto.6.6.12513
- Mizushima N, Yoshimori T, Ohsumi Y. The role of Atg proteins in autophagosome formation. Annu Rev Cell Dev Biol 2011; 27:107-32; PMID:21801009; http://dx.doi.org/10.1146/annurev-cellbio-092910-154005
- Axe EL, Walker SA, Manifava M, Chandra P, Roderick HL, Habermann A, Griffiths G, Ktistakis NT. Autophagosome formation from membrane compartments enriched in phosphatidylinositol 3-phosphate and dynamically connected to the endoplasmic reticulum. J Cell Biol 2008; 182:685-701; PMID:18725538; http://dx.doi.org/10.1083/jcb.200803137
- Itakura E, Mizushima N. Characterization of autophagosome formation site by a hierarchical analysis of mammalian Atg proteins. Autophagy 2010; 6:764-76; PMID:20639694; http://dx.doi.org/10.4161/auto.6.6.12709
- King JS. Autophagy across the eukaryotes: Is S. cerevisiae the odd one out? Autophagy 2012; 8:1159-62; PMID:22722653; http://dx.doi.org/10.4161/auto.20527
- King JS, Veltman DM, Insall RH. The induction of autophagy by mechanical stress. Autophagy 2011; 7:1490-9; PMID:22024750; http://dx.doi.org/10.4161/auto.7.12.17924
- Otto GP, Wu MY, Kazgan N, Anderson OR, Kessin RH. Macroautophagy is required for multicellular development of the social amoeba Dictyostelium discoideum. J Biol Chem 2003; 278:17636-45; PMID:12626495; http://dx.doi.org/10.1074/jbc.M212467200
- Otto GP, Wu MY, Kazgan N, Anderson OR, Kessin RH. Dictyostelium macroautophagy mutants vary in the severity of their developmental defects. J Biol Chem 2004; 279:15621-9; PMID:14736886; http://dx.doi.org/10.1074/jbc.M311139200
- Calvo-Garrido J, Escalante R. Autophagy dysfunction and ubiquitin-positive protein aggregates in Dictyostelium cells lacking Vmp1. Autophagy 2010; 6:100-9; PMID:20009561; http://dx.doi.org/10.4161/auto.6.1.10697
- Tung SM, Unal C, Ley A, Pena C, Tunggal B, Noegel AA, Krut O, Steinert M, Eichinger L. Loss of Dictyostelium ATG9 results in a pleiotropic phenotype affecting growth, development, phagocytosis and clearance and replication of Legionella pneumophila. Cell Microbiol 2010; 12:765-80; PMID:20070309; http://dx.doi.org/10.1111/j.1462-5822.2010.01432.x
- Stege JT, Shaulsky G, Loomis WF. Sorting of the initial cell types in Dictyostelium is dependent on the tipA gene. Dev Biol 1997; 185:34-41; PMID:9169048; http://dx.doi.org/10.1006/dbio.1997.8538
- Stege JT, Laub MT, Loomis WF. tip genes act in parallel pathways of early Dictyostelium development. Dev Genet 1999; 25:64-77; PMID:10402673; http://dx.doi.org/10.1002/(SICI)1520-6408(1999)25:1%3c64::AID-DVG7%3e3.0.CO;2-1
- Dobson-Stone C, Rampoldi L, Bader B, Velayos Baeza A, Walker RH, Danek A, Monaco AP. Chorea-acanthocytosis. In: Pagon RA, Bird TD, Dolan CR, Stephens K, Adam MP, eds. GeneReviews. University of Washington: Seattle, WA, 1993.
- Walterfang M, Looi JC, Styner M, Walker RH, Danek A, Niethammer M, Evans A, Kotschet K, Rodrigues GR, Hughes A, et al. Shape alterations in the striatum in chorea-acanthocytosis. Psychiatry Res 2011; 192:29-36; PMID:21377843; http://dx.doi.org/10.1016/j.pscychresns.2010.10.006
- Neutel D, Miltenberger-Miltenyi G, Silva I, de Carvalho M. Chorea-acanthocytosis presenting as motor neuron disease. Muscle Nerve 2012; 45:293-5; PMID:22246890; http://dx.doi.org/10.1002/mus.22269
- Ueno S, Maruki Y, Nakamura M, Tomemori Y, Kamae K, Tanabe H, Yamashita Y, Matsuda S, Kaneko S, Sano A. The gene encoding a newly discovered protein, chorein, is mutated in chorea-acanthocytosis. Nat Genet 2001; 28:121-2; PMID:11381254; http://dx.doi.org/10.1038/88825
- Dobson-Stone C, Danek A, Rampoldi L, Hardie RJ, Chalmers RM, Wood NW, Bohlega S, Dotti MT, Federico A, Shizuka M, et al. Mutational spectrum of the CHAC gene in patients with chorea-acanthocytosis. Eur J Hum Genet: EJHG 2002; 10:773-81; PMID:12404112; http://dx.doi.org/10.1038/sj.ejhg.5200866
- Velayos-Baeza A, Vettori A, Copley RR, Dobson-Stone C, Monaco AP. Analysis of the human VPS13 gene family. Genomics 2004; 84:536-49; PMID:15498460; http://dx.doi.org/10.1016/j.ygeno.2004.04.012
- Tomiyasu A, Nakamura M, Ichiba M, Ueno S, Saiki S, Morimoto M, Kobal J, Kageyama Y, Inui T, Wakabayashi K, et al. Novel pathogenic mutations and copy number variations in the VPS13A gene in patients with chorea-acanthocytosis. Am J Med Genet B Neuropsychiatr Genet 2011; 156B:620-31; PMID:21598378; http://dx.doi.org/10.1002/ajmg.b.31206
- Dobson-Stone C, Velayos-Baeza A, Filippone LA, Westbury S, Storch A, Erdmann T, Wroe SJ, Leenders KL, Lang AE, Dotti MT, et al. Chorein detection for the diagnosis of chorea-acanthocytosis. Ann Neurol 2004; 56:299-302; PMID:15293285; http://dx.doi.org/10.1002/ana.20200
- Tomemori Y, Ichiba M, Kusumoto A, Mizuno E, Sato D, Muroya S, Nakamura M, Kawaguchi H, Yoshida H, Ueno S, et al. A gene-targeted mouse model for chorea-acanthocytosis. J Neurochem 2005; 92:759-66; PMID:15686477; http://dx.doi.org/10.1111/j.1471-4159.2004.02924.x
- Kurano Y, Nakamura M, Ichiba M, Matsuda M, Mizuno E, Kato M, Agemura A, Izumo S, Sano A. In vivo distribution and localization of chorein. Biochem Biophys Res Commun 2007; 353:431-5; PMID:17188237; http://dx.doi.org/10.1016/j.bbrc.2006.12.059
- Alesutan I, Seifert J, Pakladok T, Rheinlaender J, Lebedeva A, Towhid ST, Stournaras C, Voelkl J, Schäffer TE, Lang F. Chorein sensitivity of actin polymerization, cell shape and mechanical stiffness of vascular endothelial cells. Cell Physiol Biochem 2013; 32:728-42; PMID:24080826; http://dx.doi.org/10.1159/000354475
- Foller M, Hermann A, Gu S, Alesutan I, Qadri SM, Borst O, Schmidt EM, Schiele F, vom Hagen JM, Saft C, et al. Chorein-sensitive polymerization of cortical actin and suicidal cell death in chorea-acanthocytosis. FASEB J: Off Pub Federat Am Soc Exp Biol 2012; 26:1526-34; PMID:22227296; http://dx.doi.org/10.1096/fj.11-198317
- Park JS, Neiman AM. VPS13 regulates membrane morphogenesis during sporulation in Saccharomyces cerevisiae. J Cell Sci 2012; 125:3004-11; PMID:22442115; http://dx.doi.org/10.1242/jcs.105114
- Samaranayake HS, Cowan AE, Klobutcher LA. Vacuolar protein sorting protein 13A, TtVPS13A, localizes to the tetrahymena thermophila phagosome membrane and is required for efficient phagocytosis. Eukar Cell 2011; 10:1207-18; PMID:21764909; http://dx.doi.org/10.1128/EC.05089-11
- Brickner JH, Fuller RS. SOI1 encodes a novel, conserved protein that promotes TGN-endosomal cycling of Kex2p and other membrane proteins by modulating the function of two TGN localization signals. J Cell Biol 1997; 139:23-36; PMID:9314526; http://dx.doi.org/10.1083/jcb.139.1.23
- Jiang P, Mizushima N. Autophagy and human diseases. Cell Res 2014; 24:69-79; PMID:24323045; http://dx.doi.org/10.1038/cr.2013.161
- Yang ZJ, Chee CE, Huang S, Sinicrope F. Autophagy modulation for cancer therapy. Cancer Biol Ther 2011; 11:169-76; PMID:21263212; http://dx.doi.org/10.4161/cbt.11.2.14663
- Xie M, Morales CR, Lavandero S, Hill JA. Tuning flux: autophagy as a target of heart disease therapy. Curr Opin Cardiol 2011; 26:216-22; PMID:21415729; http://dx.doi.org/10.1097/HCO.0b013e328345980a
- Calvo-Garrido J, Carilla-Latorre S, Mesquita A, Escalante R. A proteolytic cleavage assay to monitor autophagy in Dictyostelium discoideum. Autophagy 2011; 7:1063-8; PMID:21876387; http://dx.doi.org/10.4161/auto.7.9.16629
- Shui W, Sheu L, Liu J, Smart B, Petzold CJ, Hsieh TY, Pitcher A, Keasling JD, Bertozzi CR. Membrane proteomics of phagosomes suggests a connection to autophagy. Proc Natl Acad Sci U S A 2008; 105:16952-7; PMID:18971338; http://dx.doi.org/10.1073/pnas.0809218105
- Mehta P, Henault J, Kolbeck R, Sanjuan MA. Noncanonical autophagy: one small step for LC3, one giant leap for immunity. Curr Opin Immunol 2014; 26:69-75; PMID:24556403; http://dx.doi.org/10.1016/j.coi.2013.10.012
- Polson HE, de Lartigue J, Rigden DJ, Reedijk M, Urbe S, Clague MJ, Tooze SA. Mammalian Atg18 (WIPI2) localizes to omegasome-anchored phagophores and positively regulates LC3 lipidation. Autophagy 2010; 6:506-22; PMID:20505359; http://dx.doi.org/10.4161/auto.6.4.11863
- Dorsey FC, Steeves MA, Prater SM, Schroter T, Cleveland JL. Monitoring the autophagy pathway in cancer. Methods Enzymol 2009; 453:251-71; PMID:19216910; http://dx.doi.org/10.1016/S0076-6879(08)04012-3
- Klionsky DJ, Abdalla FC, Abeliovich H, Abraham RT, Acevedo-Arozena A, Adeli K, Agholme L, Agnello M, Agostinis P, Aguirre-Ghiso JA, et al. Guidelines for the use and interpretation of assays for monitoring autophagy. Autophagy 2012; 8:445-544; PMID:22966490; http://dx.doi.org/10.4161/auto.19496
- Ni HM, Bockus A, Wozniak AL, Jones K, Weinman S, Yin XM, Ding WX. Dissecting the dynamic turnover of GFP-LC3 in the autolysosome. Autophagy 2011; 7:188-204; PMID:21107021; http://dx.doi.org/10.4161/auto.7.2.14181
- Park JS, Okumura Y, Tachikawa H, Neiman AM. SPO71 encodes a developmental stage-specific partner for VPS13 in Saccharomcyes cerevisiae. Eukar Cell 2013; 12:1530-7; PMID:24036347; http://dx.doi.org/10.1128/EC.00239-13
- Dall'armi C, Devereaux KA, Di Paolo G. The role of lipids in the control of autophagy. Curr Biol: CB 2013; 23:R33-45; PMID:23305670; http://dx.doi.org/10.1016/j.cub.2012.10.041
- Schneider JL, Cuervo AM. Autophagy and human disease: emerging themes. Curr Opin Genet Dev 2014; 26C:16-23; ; http://dx.doi.org/10.1016/j.gde.2014.04.003
- Pang KM, Lynes MA, Knecht DA. Variables controlling the expression level of exogenous genes in Dictyostelium. Plasmid 1999; 41:187-97; PMID:10366524; http://dx.doi.org/10.1006/plas.1999.1391
- Escalante R, Sastre L. A serum response factor homolog is required for spore differentiation in Dictyostelium. Development 1998; 125:3801-8; PMID:9729488
- Rivero F, Maniak M. Quantitative and microscopic methods for studying the endocytic pathway. Methods Mol Biol (Clifton, NJ) 2006; 346:423-38; PMID:16957305
- Bampton ET, Goemans CG, Niranjan D, Mizushima N, Tolkovsky AM. The dynamics of autophagy visualized in live cells: from autophagosome formation to fusion with endolysosomes. Autophagy 2005; 1:23-36; PMID:16874023; http://dx.doi.org/10.4161/auto.1.1.1495
- Mesquita A, Calvo-Garrido J, Carilla-Latorre S, Escalante R. Monitoring autophagy in Dictyostelium. Methods Mol Biol 2013; 983:461 70; PMID:23494324; http://dx.doi.org/10.1007/978-1-62703-302-2_26