Abstract
Recent evidence has indicated that the lysosome is able to act as a signaling organelle that senses nutrient availability and generates an adaptive response that is important for cellular homeostasis. We recently discovered another example of lysosomal signaling where lysosomal calcium release activates the master autophagy regulator TFEB via the phosphatase calcineurin.
The lysosome plays a fundamental role in the adaptive response of the cell to environmental cues. Energy-demanding conditions, such as starvation and physical exercise, which involve a switch from anabolic to catabolic pathways, require an induction of the lysosomal-autophagic pathway. Such induction is mediated by both posttranslational and transcriptional mechanisms. TFEB (transcription factor EB) plays a major role in the induction of lysosomal biogenesis and autophagy by positively regulating the expression of a large subset of lysosomal and autophagic genes. During starvation TFEB translocates from the cytoplasm to the nucleus, where it exerts its function. TFEB subcellular localization is controlled by phosphorylation, which is mediated by the MTORC1 kinase complex and occurs on the lysosomal surface where MTORC1 is part of a nutrient-sensing machinery.
Phosphorylation of TFEB residue Ser211 promotes its interaction with the YWHAB/14-3-3 protein and results in a cytoplasmic localization. Conversely, conditions that lead to MTOR inhibition, such as starvation, promote TFEB nuclear translocation and transcriptional activation of lysosomal-autophagic genes. The discovery that the MTOR kinase resides on the lysosomal surface and controls the activity of a master transcriptional regulator, has revealed the presence of a lysosome-to-nucleus signaling mechanism.
In our study we identified another signaling pathway that originates from the lysosome and activates TFEB by dephosphorylation. To identify the phosphatase(s) that dephosphorylates TFEB we performed a siRNA screening based on a TFEB nuclear translocation assay. This screening revealed that inhibition of the calcium-activated serine/threonine phosphatase calcineurin prevents the nuclear translocation of TFEB in starved cells. This effect was mimicked by pharmacological inhibition of calcineurin. Conversely, overexpression of a constitutively active form of calcineurin promotes TFEB nuclear translocation and transcriptional activity in cells grown in nutrient-rich conditions. Similar experiments were conducted in skeletal muscle cells in vivo, suggesting that activation of calcineurin is sufficient to control TFEB nuclear translocation. In addition to starvation, another energy-demanding condition, such as physical exercise, is able to promote the nuclear translocation of TFEB in skeletal muscle cells, a response that is inhibited by the electroporation of the endogenous calcineurin inhibitor CAIN. We also demonstrated that calcineurin interacts with TFEB and dephosphorylates TFEB residues Ser211 and Ser142, 2 key sites involved in the subcellular localization of TFEB. Interestingly, calcineurin is physically associated with TFEB in both normal nutrient conditions and during starvation. This feature is typical of well-known substrates of calcineurin such as NFAT. We also found that the induction of TFEB nuclear translocation after Torin1-mediated MTOR inhibition is significantly suppressed in calcineurin-silenced cells, suggesting that the control of TFEB subcellular localization by calcineurin is independent from MTOR activity and that calcineurin acts downstream of MTOR in the regulation of TFEB.
Calcineurin is activated by calcium, and TFEB subcellular localization is modulated by calcineurin, therefore we investigated whether calcium regulates TFEB nuclear translocation. We found that calcium chelation significantly inhibits TFEB nuclear translocation in both starved cells and in cells treated with Torin 1, confirming the involvement of calcium in the regulation of TFEB. Conversely, experimental manipulations that increase intracellular calcium concentrations, such as thapsigargin or ionomycin treatment, enhance the nuclear translocation of TFEB in cells under nutrient-rich conditions, an effect that in thapsigargin-treated cells requires calcineurin. In an attempt to investigate the mechanism of starvation-mediated elevation of intracellular calcium, we found that starvation does not increase bulk cytosolic calcium concentrations, but does increase the activity of a genetically-encoded calcium reporter fused to the lysosomal calcium channel MCOLN1/mucolipin 1/TRPML1. This activity is sensitive to GPN, a lysosomotropic drug, but not to extracellular calcium chelation, suggesting the involvement of lysosomal calcium stores in the starvation response. In addition, we found that starvation-mediated TFEB nuclear translocation is strongly reduced by using the fast calcium chelator BAPTA-AM but not by using the slower chelator EGTA-AM. Together these results suggest that starvation triggers the release of microdomains of calcium starting at the lysosome.
Notably, the starvation-induced nuclear translocation of TFEB is reduced by knockdown of MCOLN1 or in fibroblasts from individuals with mucolipidosis type 4, a lysosomal storage disease caused by mutations in MCOLN1. Similarly, shRNA-mediated inhibition of MCOLN1 activity reduces the exercise-induced nuclear translocation of TFEB in skeletal muscle. Conversely, MCOLN1 overexpression or agonist-mediated activation of MCOLN1 induces TFEB nuclear translocation. Thus, starvation specifically induces lysosomal calcium release through MCOLN1 generating a lysosomal microdomain that is responsible for calcineurin activation and local TFEB dephosphorylation near the lysosomal membrane. Further studies are needed to identify the precise mechanism of MCOLN1 channel activation after starvation including posttranslational modification of the lysosomal channel or changes in the local levels of endogenous modulators of MCOLN1 such as the phosphoinositides.
As TFEB transcriptionally regulates lysosomal biogenesis and autophagy, we investigated whether the modulation of this newly identified lysosomal signaling pathway that involves MCOLN1 and calcineurin modulates these functions. Transcriptome analysis of starved MEFs derived from conditional KO of the gene encoding an essential regulatory subunit of calcineurin, Ppp3r1, results in the reduction, both in terms of number of genes and amplitude of response, of lysosomal/autophagic genes compared with the wild-type counterpart. Moreover, the silencing of calcineurin or of MCOLN1 inhibits starvation-mediated autophagy as measured by the number of autophagosomes and the accumulation of autophagy substrates. Thus, our results identify a novel, lysosome-based, signaling pathway that is triggered by calcium release via MCOLN1 and induces autophagy via calcineurin-mediated dephosphorylation of TFEB (). The coordinated modulation of oppositely acting pathways involving the MTOR kinase and the calcineurin phosphatase near the lysosome identifies this organelle as a central signaling hub modulating the adaptative response of the cell to nutrients and the switch between biosynthetic and catabolic pathways.
Figure 1. Model of TFEB regulation by lysosomal calcium. In nutrient-rich conditions TFEB is phosphorylated by MTOR in the lysosome and sequestered in the cytoplasm by interacting with YWHAB/14-3-3 proteins. During starvation or physical exercise, calcium is released from the lysosome via MCOLN1 leading to local calcineurin (CaN) activation and TFEB dephosphorylation. Dephosphorylated TFEB can shuttle to the nucleus where it transcriptionally activates the lysosomal/autophagic pathway.
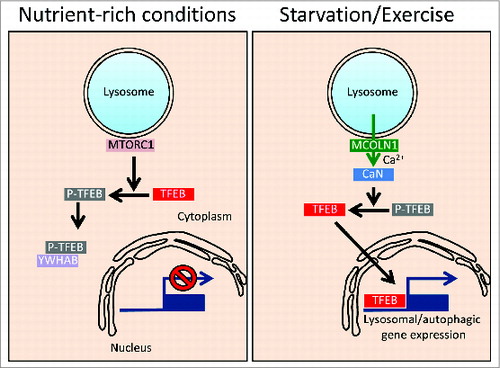
Disclosure of Potential Conflicts of Interest
No potential conflicts of interest were disclosed.