Abstract
The transcription-regulating activity of TFEB is dependent on its phosphorylation modification, but the phosphatase(s) involved in TFEB dephosphorylation have remained elusive. It has now become clear that lysosomal calcium signaling activates calcineurin, an endogenous serine/threonine phosphatase, which dephosphorylate TFEB leading to upregulation of autophagy.
Autophagy is a conserved cellular process critical for maintaining cellular homeostasis through the targeting of cytosolic proteins and damaged organelles to the lysosomes for degradation. As a major cellular catabolic system, the autophagic process is tightly controlled. Normally, autophagy occurs constitutively at basal levels but is dramatically stimulated by various extra- and intracellular stresses, such as nutrient or growth factor limitation, oxidative stress, and accumulation of damaged organelles or misfolded proteins.Citation1 In eukaryotes, the underlying mechanisms for regulating autophagy exist at various levels, from transcriptional activation to post-translational protein modification.Citation2 Recent advances in understanding the molecular mechanisms of autophagy have highlighted the importance of transcription machinery, for example, via the regulation of TFEB (transcription factor EB), on autophagy-related gene expression. TFEB is a member of the basic-helix-loop-helix leucine-zipper transcription factor MiTF/TFE family. In the past 5 y, TFEB has been identified as a master regulator of antophagic and lysosomal biogenesis.Citation3,4 In response to starvation or lysosomal dysfunction, TFEB positively regulates the expression of genes belonging to the Coordinated Lysosomal Expression and Regulation (CLEAR) network, including ATG9, LC3, SQSTM1, and LAMP1. These genes are involved in the sequential steps of autophagy, from cargo recognition and autophagosome formation to vesicle fusion and substrate degradation.Citation3,5-7
The transcriptional activity of TFEB is closely related to phosphorylation on specific amino acid residues. Under normal conditions, TFEB is located in the cytoplasm in an inactive, phosphorylated form. However, under specific conditions, when cells are stimulated, such as hunger, drugs, and oxidative stress, TFEB is dephosphorylated and translocated into the nucleus, thus leading to a robust transactivation of its target genes.Citation6,8 To date, at least 3 different kinases have been shown to phosphorylate TFEB: MTORC1, MAPK1/ERK2, and PRKCB/protein kinase Cβ.Citation9 These kinases can phosphorylate multiple serine residues of TFEB, which have distinct effects in regulating its activity. For instance, phosphorylation of Ser142 and Ser211 by MTORC1 and/or MAPK1 is crucial in determining the subcellular localization of TFEB.Citation3,5,8 In contrast, PRKCB-induced phosphorylation of Ser461, Ser466, and Ser468 is suggested to stabilize TFEB and increases its activity.Citation10 Among these kinases, MTORC1 has been extensively investigated because it is a major kinase complex that controls cell growth and negatively regulates autophagy. Under conditions of nutrient sufficiency, MTORC1 is active and inhibits autophagy by phosphorylating ATG13 and ULK1, thus repressing ULK1 kinase activity. More importantly, MTORC1 has recently been identified as a key regulator of TFEB function.Citation5,11,12 In the presence of nutrients, MTORC1 phosphorylates TFEB at the lysosome surface, which promotes the binding of TFEB to YWHA/14-3-3 proteins and inhibits its transport into the nucleus. Conversely, conditions that impair MTORC1 activity reduce TFEB phosphorylation, and increase the accumulation of TFEB in the nucleus, where it orchestrates the expansion of lysosomal and autophagic compartments.Citation13
The phosphorylation status of TFEB is not only influenced by the above-mentioned kinases, but also by protein phosphatases. For instance, Torin1, an MTOR inhibitor, can promote TFEB dephosphorylation, and leads to TFEB nuclear localization in human pancreatic cancer cells. In contrast, pre-treatment with calyculin A, a serine/threonine phosphatase inhibitor, prevents Torin1-induced TFEB mobility shift, supporting a role for endogenous serine/threonine phosphatases in the dephosphorylation of TFEB.Citation14 However, until recently the phosphatase responsible for TFEB dephosphorylation has remained elusive; now it has become clear that calcineurin, an endogenous Ca2+-calmodulin-dependent protein phosphatase, is involved in the process of TFEB dephosphorylation. Medina et al. report that lysosomal calcium signaling can induce autophagy and lysosomal biogenesis through calcineurin-mediated TFEB activation (see the punctum in issue 11–6 of Autophagy).Citation15 To identify the phosphatase that dephosphorylate TFEB, Medina and colleagues tested the effects of the specific inhibition of 231 phosphatases on TFEB translocation, and identified calcineurin as a candidate phosphatase responsible for TFEB dephosphorylation. The authors next analyzed the possible interaction between lysosomal calcium signaling, calcineurin, and TFEB in mammalian cells using these genetic and pharmacological tools. Finally, they found that lysosomal Ca2+ release through MCOLN1 (mucolipin 1) activates calcineurin, which dephosphorylates TFEB at Ser211 and Ser142, thus promoting its nuclear translocation.
Ca2+ is one of the most important regulators of cell survival and death processes. As a second messenger, Ca2+ is able to activate various regulatory proteins such as enzymes and transcriptional factors. Physiological roles of calcium signaling range widely, including the regulation of muscle contraction, neuronal transmission, cellular motility, fertilization, and cell growth and proliferation.Citation16 The 10,000-fold difference between extracellular and cytosolic Ca2+ concentration is maintained by the impermeability of the plasma membrane to Ca2+ and by transport mechanisms that remove Ca2+ from the cytoplasm. Furthermore, intracellular Ca2+ is actively pumped from the cytosol across the plasma membrane and is sequestered in the endoplasmic reticulum, mitochondria, and lysosomes.Citation17
It is now well established that intracellular Ca2+ is one of the regulators of autophagy. Although it is still controversial as to whether calcium is an activator or inhibitor for cell autophagy, accumulating evidence suggests that it has dual roles in autophagy regulation. In healthy cells, a constitutive Ca2+ release from the ER through ITPR/inositol-1,4,5-trisphosphate receptor in response to basal levels of inositol-1,4,5-trisphosphate is present. Due to the presence of ER-mitochondria microdomains, this Ca2+ will be taken up by mitochondria, where it stimulates mitochondrial bioenergetics, suppressing basal autophagy. However, stress conditions may induce elevation of cytoplasmic Ca2+ levels by promoting Ca2+ influx into, or inhibiting Ca2+ efflux from, the cytoplasm. Subsequently, sustained Ca2+ signaling in the cytosol will result in autophagy activation through many effector molecules.Citation18,19 In this respect, a mechanism for activation of autophagy by inhibition of the MTOR pathway has been proposed. Ca2+ released from intracellular stores or imported from the extracellular space via distinct calcium channels activates CAMKK2/CaMKKβ, which phosphorylates and activates AMPK. Activated AMPK inactivates MTORC1, releasing its break on autophagy ().Citation20 In contrast to TFEB regulation mediated by protein kinases, Medina et al. propose a new signaling pathway of calcium–activated transcriptional regulation by protein phosphatases. Calcineurin, also known as protein phosphatase 2B, is a serine/threonine-specific protein phosphatase that is active only after binding calmodulin in response to elevated cytosolic Ca2+. Calcineurin is ubiquitously distributed in eukaryotes, with high levels in most types of neurons and lymphocytes. Calcineurin participates in a number of cellular processes and Ca2+-dependent signal transduction pathways.
Figure 1. Regulation of TFEB activity by intracellular calcium signaling. Extra- and intracellular stimuli induce elevation of cytoplasmic Ca2+ levels by releasing Ca2+ from the lysosome. Elevated cytosol Ca2+ can suppress MTORC1-dependent pathways, and (or) activate calcineurin-dependent pathways, subsequently inducing dephosphorylation of TFEB, and resulting in the activation of autophagy.
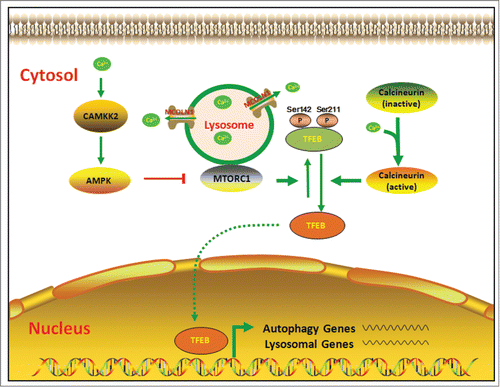
Like autophagy, disturbance of intracellular calcium homeostasis is a key molecular event that determines cell survival and cell death. Increasing evidence supports the idea that both dysfunction of autophagy and deregulation of calcineurin signaling have been implicated in the pathogenesis of several human diseases, including cancer, neurodegeneration, and infectious diseases.Citation21–25 The study by Medina et al. has demonstrated an interplay between calcium and autophagy, in which calcium can indirectly drive the transcription of autophagy-related genes through the calcineurin-dependent dephosphorylation and nuclear translocation of TFEB ().
Medina et al.'s work reveals an important mechanism that regulates autophagy. Meanwhile, their study also sets the stage for further investigation. Open questions include the following: This research suggests that calcium derived from lysosomes can activate TFEB under starvation conditions and during physical exercise. However, some pathological conditions, especially oxidative stress and toxic stimuli can induce a calcium increase from the extracellular space and other organelles. Whether the nonlysosomal calcium signaling can also activate TFEB still remains unclear. In addition to the aforementioned dephosphorylation modification, there is the possibility of other machinery, through which calcium activates TFEB transcription. A recent study confirmed that both NR1H4/FXR and CREB can regulate expression of TFEB.Citation26 Moreover, Singh et al. found that CRE activation involves Ca2+-calcineurin-dependent nuclear translocation of transducers of regulated CREBBP (CREB binding protein).Citation27 These studies provide some new clues with regard to the function of calcium-mediated TFEB regulation via transcription factor networks. Studies addressing these questions will help to clarify the role of calcium in autophagy.
Collectively, Medina and colleagues have identified a novel mechanism that results in a prolonged and sustained activation of autophagy. Autophagy dysfunction has been linked to several human disorders and enhancement of autophagy can have a therapeutic effect in certain animal models. This opens the possibility of using Ca2+ channel modulators in control of autophagy for therapeutic purposes.
Disclosure of Potential Conflicts of Interest
No potential conflicts of interest were disclosed.
Funding
This work was supported by The Fundamental Research Funds of Shandong University (No. 2014JC018).
References
- Mizushima N, Klionsky DJ. Protein turnover via autophagy: implications for metabolism. Annu Rev Nutrit 2007; 27:19-40; http://dx.doi.org/10.1146/annurev.nutr.27.061406.093749
- He CC, Klionsky DJ. Regulation mechanisms and signaling pathways of autophagy. Annu Rev Genet 2009; 43:67–93; PMID:19653858; http://dx.doi.org/10.1146/annurev-genet-102808-114910
- Settembre C, Di Malta C, Polito VA, Garcia Arencibia M, Vetrini F, Erdin S, Erdin SU, Huynh T, Medina D, Colella P, et al. TFEB links autophagy to lysosomal biogenesis. Science 2011; 332:1429–33; PMID:21617040; http://dx.doi.org/10.1126/science.1204592
- Sardiello M, Palmieri M, di Ronza A, Medina DL, Valenza M, Gennarino VA, Di Malta C, Donaudy F, Embrione V, Polishchuk RS, et al. A Gene Network Regulating Lysosomal Biogenesis and Function. Science 2009; 325:473–7; PMID:19556463
- Roczniak-Ferguson A, Petit CS, Froehlich F, Qian S, Ky J, Angarola B, Walther TC, Ferguson SM. The Transcription Factor TFEB Links mTORC1 Signaling to Transcriptional Control of Lysosome Homeostasis. Sci Signal 2012; 5; PMID:22692423
- Fullgrabe J, Klionsky DJ, Joseph B. The return of the nucleus: transcriptional and epigenetic control of autophagy. Nat Rev Mol Cell Bio 2014; 15:65–74; http://dx.doi.org/10.1038/nrm3716
- Settembre C, Ballabio A. TFEB regulates autophagy: an integrated coordination of cellular degradation and recycling processes. Autophagy 2011; 7:1379–81; PMID:21785263; http://dx.doi.org/10.4161/auto.7.11.17166
- Martina JA, Chen Y, Gucek M, Puertollano R. MTORC1 functions as a transcriptional regulator of autophagy by preventing nuclear transport of TFEB. Autophagy 2012; 8:903–14; PMID:22576015; http://dx.doi.org/10.4161/auto.19653
- Settembre C, Fraldi A, Medina DL, Ballabio A. Signals from the lysosome: a control centre for cellular clearance and energy metabolism. Nat Rev Mol Cell Biol 2013; 14:283–96; PMID:23609508; http://dx.doi.org/10.1038/nrm3565
- Ferron M, Settembre C, Shimazu J, Lacombe J, Kato S, Rawlings DJ, Ballabio A, Karsenty G. A RANKL-PKC b-TFEB signaling cascade is necessary for lysosomal biogenesis in osteoclasts. Gene Dev 2013; 27:955-69; PMID:23599343; http://dx.doi.org/10.1101/gad.213827.113
- Laplante M, Sabatini DM. Regulation of mTORC1 and its impact on gene expression at a glance. J Cell Sci 2013; 126:1713–9; PMID:23641065; http://dx.doi.org/10.1242/jcs.125773
- Settembre C, Zoncu R, Medina DL, Vetrini F, Erdin S, Erdin S, Huynh T, Ferron M, Karsenty G, Vellard MC, et al. A lysosome-to-nucleus signalling mechanism senses and regulates the lysosome via mTOR and TFEB. EMBO J 2012; 31:1095–108; PMID:22343943; http://dx.doi.org/10.1038/emboj.2012.32
- Settembre C, Medina DL. TFEB and the CLEAR network. Method Cell Biol 2015; 126:45–62; PMID:25665440
- Marchand B, Arsenault D, Raymond-Fleury A, Boisvert FM, Boucher MJ. Glycogen Synthase Kinase-3 (GSK3) Inhibition Induces Prosurvival Autophagic Signals in Human Pancreatic Cancer Cells. J Biol Chem 2015; 290:5592–605; PMID:25561726; http://dx.doi.org/10.1074/jbc.M114.616714
- Medina DL, Di Paola S, Peluso I, Armani A, De Stefani D, Venditti R, Montefusco S, Scotto-Rosato A, Prezioso C, Forrester A, et al. Lysosomal calcium signalling regulates autophagy through calcineurin and TFEB. Nat Cell Biol 2015; 17:288–99; PMID:25720963; http://dx.doi.org/10.1038/ncb3114
- Berridge MJ, Lipp P, Bootman MD. The versatility and universality of calcium signalling. Nat Rev Mol Cell Biol 2000; 1:11–21; PMID:11413485; http://dx.doi.org/10.1038/35036035
- Morgan AJ, Platt FM, Lloyd-Evans E, Galione A. Molecular mechanisms of endolysosomal Ca2+ signalling in health and disease. Biochem J 2011; 439:349–74; PMID:21992097; http://dx.doi.org/10.1042/BJ20110949
- Decuypere JP, Bultynck G, Parys JB. A dual role for Ca2+ in autophagy regulation. Cell Calcium 2011; 50:242–50; PMID:21571367; http://dx.doi.org/10.1016/j.ceca.2011.04.001
- Parys JB, Decuypere JP, Bultynck G. Role of the inositol 1,4,5-trisphosphate receptor/Ca2+-release channel in autophagy. Cell Commun Signal 2012; 10; PMID:22487193
- Hoyer-Hansen M, Bastholm L, Szyniarowski P, Campanella M, Szabadkai G, Farkas T, Bianchi K, Fehrenbacher N, Elling F, Rizzuto R, et al. Control of macroautophagy by calcium, calmodulin-dependent kinase kinase-b, and Bcl−2. Molecular Cell 2007; 25:193–205; PMID:17244528; http://dx.doi.org/10.1016/j.molcel.2006.12.009
- Shi M, Zhang T, Sun L, Luo Y, Liu DH, Xie ST, Song XY, Wang GF, Chen XL, Zhou BC, et al. Calpain, Atg5 and Bak play important roles in the crosstalk between apoptosis and autophagy induced by influx of extracellular calcium. Apoptosis 2013; 18:435–51; PMID:23242420; http://dx.doi.org/10.1007/s10495-012-0786-2
- Das A, Pushparaj C, Herreros J, Nager M, Vilella R, Portero M, Pamplona R, Matias-Guiu X, Martí RM, Cantí C. T-type calcium channel blockers inhibit autophagy and promote apoptosis of malignant melanoma cells. Pigm Cell Melanoma R 2013; 26; PMID:23931340; http://dx.doi.org/10.1111/pcmr.12155
- Knoferle J, Koch JC, Ostendorf T, Michel U, Planchamp V, Vutova P, Tönges L, Stadelmann C, Brück W, Bähr M, et al. Mechanisms of acute axonal degeneration in the optic nerve in vivo. P Natl Acad Sci USA 2010; 107:6064–9; PMID:20231460; http://dx.doi.org/10.1073/pnas.0909794107
- Vicencio JM, Lavandero S, Szabadkai G. Ca2+, autophagy and protein degradation: Thrown off balance in neurodegenerative disease. Cell Calcium 2010; 47:112–21; PMID:20097418; http://dx.doi.org/10.1016/j.ceca.2009.12.013
- Singhal J, Agrawal N, Vashishta M, Priya NG, Tiwari BK, Singh Y, Raman R, Natarajan K. Suppression of Dendritic Cell-mediated Responses by Genes in Calcium and Cysteine Protease Pathways during Mycobacterium tuberculosis Infection. J Biol Chem 2012; 287:11108–21; PMID:22337888; http://dx.doi.org/10.1074/jbc.M111.300319
- Seok S, Fu T, Choi SE, Li Y, Zhu R, Kumar S, Sun X, Yoon G, Kang Y, Zhong W, et al. Transcriptional regulation of autophagy by an FXR-CREB axis. Nature 2014; 516:108–U274; PMID:25383523
- Singh N, Yadav M, Singh AK, Kumar H, Dwivedi SKD, Mishra JS, Gurjar A, Manhas A, Chandra S, Yadav PN, et al. Synthetic FXR Agonist GW4064 Is a Modulator of Multiple G Protein-Coupled Receptors. Mol Endocrinol 2014; 28:659–73; PMID:24597548; http://dx.doi.org/10.1210/me.2013-1353