ABSTRACT
High-throughput screening identified 5 chemical analogs (termed the WX8-family) that disrupted 3 events in lysosome homeostasis: (1) lysosome fission via tubulation without preventing homotypic lysosome fusion; (2) trafficking of molecules into lysosomes without altering lysosomal acidity, and (3) heterotypic fusion between lysosomes and autophagosomes. Remarkably, these compounds did not prevent homotypic fusion between lysosomes, despite the fact that homotypic fusion required some of the same machinery essential for heterotypic fusion. These effects varied 400-fold among WX8-family members, were time and concentration dependent, reversible, and resulted primarily from their ability to bind specifically to the PIKFYVE phosphoinositide kinase. The ability of the WX8-family to prevent lysosomes from participating in macroautophagy/autophagy suggested they have therapeutic potential in treating autophagy-dependent diseases. In fact, the most potent family member (WX8) was 100-times more lethal to ‘autophagy-addicted’ melanoma A375 cells than the lysosomal inhibitors hydroxychloroquine and chloroquine. In contrast, cells that were insensitive to hydroxychloroquine and chloroquine were also insensitive to WX8. Therefore, the WX8-family of PIKFYVE inhibitors provides a basis for developing drugs that could selectively kill autophagy-dependent cancer cells, as well as increasing the effectiveness of established anti-cancer therapies through combinatorial treatments.
Abbreviations
ACTB: actin beta; Baf: bafilomycin A1; BECN1: beclin 1; BODIPY: boron-dipyrromethene; BORC: BLOC-1 related complex; BRAF: B-Raf proto-oncogene, serine/threonine kinase; BSA: bovine serum albumin; CTSD: cathepsin D; CQ: chloroquine; DNA: deoxyribonucleic acid; EC50: half maximal effective concentration; GAPDH: glyceraldehyde-3-phosphate dehydrogenase; GFP: green fluorescent protein; HCQ: hydroxychloroquine; HOPS complex: homotypic fusion and protein sorting complex; Kd: equilibrium binding constant; IC50: half maximal inhibitory concentration; KO: knockout; LAMP1: lysosomal associated membrane protein 1; MAP1LC3A: microtubule associated protein 1 light chain 3 alpha; MES: 2-(N-morpholino)ethanesulphonic acid; MTOR: mechanistic target of rapamycin kinase; μM: micromolar; NDF: 3-methylbenzaldehyde (2,6-dimorpholin-4-ylpyrimidin-4-yl)hydrazine;NEM: N-ethylmaleimide; NSF: N-ethylmaleimide sensitive factor; PBS: phosphate-buffered saline; PIKFYVE: phosphoinositide kinase, FYVE-type zinc finger containing; PIP4K2C: phosphatidylinositol-5-phosphate 4-kinase type 2 gamma; PtdIns3P: phosphatidylinositol 3-phosphate; PtdIns(3,5)P2: phosphatidylinositol 3,5-biphosphate; RFP: red fluorescent protein; RPS6: ribosomal protein S6; RPS6KB1: ribosomal protein S6 kinase B1; SQSTM1: sequestosome 1; TWEEN 20: polysorbate 20; V-ATPase: vacuolar-type H+-translocating ATPase; VPS39: VPS39 subunit of HOPS complex; VPS41: VPS41 subunit of HOPS complex; WWL: benzaldehyde [2,6-di(4-morpholinyl)-4-pyrimidinyl]hydrazone; WX8: 1H-indole-3-carbaldehyde [4-anilino-6-(4-morpholinyl)-1,3,5-triazin-2-yl]hydrazine; XBA: N-(3-chloro-4-fluorophenyl)-4,6-dimorpholino-1,3,5-triazin-2-amine hydrochloride; XB6: N-(4-ethylphenyl)-4,6-dimorpholino-1,3,5-triazin-2-amine hydrochloride
Introduction
The formation, function and dissolution of lysosomes constitute lysosome homeostasis, an integral component of nutrient mobilization and energy homeostasis in all human cells and tissues [Citation1]. Lysosomes are the acidic organelles that digest macromolecules for the regeneration of basic building blocks, cellular housekeeping, or pathogen degradation. They receive their substrates either by direct engulfment of a cytoplasmic cargo (microautophagy), or by fusing with endosomes or autophagosomes (macroautophagy). Macroautophagy (herein referred to as autophagy) is a highly-conserved mechanism that delivers dispensable or damaged cytoplasmic material to lysosomes for degradation [Citation2]. Autophagy occurs through a sequence of events that include phagophore formation, engulfment of cytoplasmic cargo to form an autophagosome, fusion of the autophagosome with lysosomes to form an autolysosome, cargo degradation, recycling the degradation products to the cytoplasm, and finally, lysosome reformation [Citation3].
Autophagy plays a critical role in cancer survival through its effects on the host immune surveillance system, cell death pathways, deregulation of cellular metabolism, and drug resistance [Citation4,Citation5]. Current efforts to inhibit autophagy in cancer chemotherapy have relied on chloroquine and hydroxychloroquine [Citation6–Citation8]. These drugs diffuse into acidic compartments where they are protonated and trapped, thereby decreasing lysosomal acidity and rendering pH-dependent lysosomal hydrolases nonfunctional [Citation9,Citation10]. Consequently, autophagy-mediated cell survival is impaired, and tumor cells treated with chloroquine are less able to withstand therapeutic treatments and are therefore sensitized to therapy [Citation11,Citation12]. Cancer cells, however, can survive acidic stress by upregulating autophagy, suggesting that interrupting autophagy with chloroquine might not be achieved in an acidic tumor microenvironment [Citation13,Citation14]. Therefore, therapeutic strategies that disrupt multiple events in lysosome homeostasis are required in order to suppress nutrient recovery and energy production [Citation15–Citation17].
Here we report the discovery of a family of PIKFYVE inhibitors that disrupt lysosome fission, traffic into lysosomes, and formation of autolysosomes. They prevented heterotypic fusion between lysosomes and autophagosomes, thereby impairing autophagy. Remarkably, they did not prevent homotypic fusion between lysosomes, but they did prevent lysosome fission, thereby disrupting lysosome turnover. Consequently, lysosomes became enlarged and functionally impaired. Such extensive disruption of lysosome homeostasis at 3 distinct events likely accounts for the fact that the most potent member of this family (WX8) could selectively kill autophagy-dependent cancer cells without affecting either the proliferation or viability of non-malignant cells, thereby revealing the WX8-family’s potential in cancer chemotherapy.
Results
The WX8-family induced accumulation of cytoplasmic vacuoles
A family of 5 small molecules that induced cytoplasmic vacuolization in human cells was discovered in a high throughput screen for compounds that induce excess DNA replication selectively in cancer cells compared to nonmalignant cells [Citation18,Citation19]. Of the 127 most promising candidates from the 343,078 molecules screened, 77 were rescreened at 12 different concentrations in order to confirm the primary results. Of these compounds, 40 were selected for further analysis. Unexpectedly, 5 of these compounds ()) rapidly induced accumulation of cytoplasmic vacuoles that were readily visible by light microscopy ()). Vacuolization was induced in a wide variety of human cells, including normal mammary epithelia and fibroblasts, immortalized cells, and cells derived from human cancers and from patients with Huntington, Parkinson, Alzheimer or Hutchinson-Gilford progeria disease. The potency of these molecules to induce vacuolization depended on which molecule was tested, its concentration, and the length of time that cells were cultured in its presence (Figures. S1–S3). The concentration required to induce vacuolization in osteosarcoma U2OS cells varied 400-fold, with WX8 the most potent and XBA the least ()).
Figure 1. The WX8-family and its effects on osteosarcoma U2OS cells. (a) The WX8-family of small molecules. Shaded regions indicate homologies. WWL and NDF consist of a pyrimidine-4-amine core with 2 morpholine adducts. XB6 and XBA consist of a 1,3,5-triazin-2-amine core with 2 morpholine adducts. WX8 contains the same 1,3,5-triazin-2-amine core with only one morpholine adduct. The remaining components consist of a benzaldehyde hydrazone adduct in NDF and WWL, a simple benzene adduct in XB6 and XBA, and benzene and a purine-like adduct in WX8. (b) Differential interference microscopic images of U2OS cells cultured for 24 h with 1 µM of the indicated compound. Vehicle is the concentration of DMSO that was introduced by addition of compounds. Fewer than 1% of the cells treated with vehicle exhibited a vacuole. (c) The WX8-family of small molecules are listed in PubChem as either a compound or a substance (https://www.ncbi.nlm.nih.gov/pubmed/). Summarized here are their effective concentrations (EC) for inducing vacuoles in U2OS cells within 4 h (Figs. S1-S3), their half maximal effective concentration (EC50) for inducing LC3-II accumulation within 4 to 8 h (Figure S4(a)), their half maximal inhibitory concentration (IC50) for suppressing cell proliferation within 3 d (Figure S4(b)), and their IC50 for reducing cellular ATP levels (viability) within 4 d (Figure S4(c)).
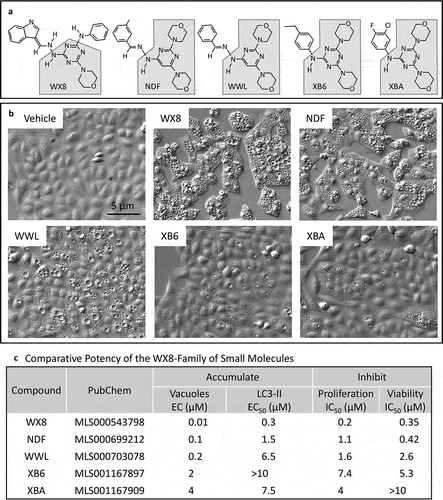
This family of small molecules also induced accumulation of 2 autophagosome biomarkers, the phosphatidylethanolamine conjugate (LC3-II) of MAP1LC3A protein (LC3-I), and the SQSTM1/p62 ubiquitin-binding protein (sequestosome 1) (examples in ). As with vacuolization, the concentrations required to detect these effects depended on the molecule tested (summarized in )). The concentration required to detect accumulation of LC3-II varied by >36-fold among the 5 molecules (Figure S4(a)). The concentration required to inhibit cell proliferation varied by 37-fold (Figure S4(b)), and the concentration required to inhibit viability as measured by loss of ATP varied by >29-fold (Figure S4(c)). Furthermore, the concentrations required to induce LC3-II accumulation, to inhibit cell proliferation, or to inhibit viability as measured by loss of ATP were greater than the concentrations required to observe vacuolization of the same cells. This difference was about 30-fold for WX8, about 10-fold for NDF, about 15-fold for WWL, and 2- to 3-fold for either XB6 and XBA ()).
Given the consistency in their relative potency among 4 different biological assays (WX8>NDF>WWL>XB6 or XBA), and the similarity in their chemical structures, they are referred to hereafter as the WX8-family.
Vacuolization resulted from lysosomal enlargement
The WX8-family rapidly induced accumulation of vacuoles in human cells that were visible by light microscopy ()). Electron microscopy of cells treated with WX8 revealed the accumulation of large empty vacuoles with a single membrane ()). Fluorescence microscopy showed that these vacuoles were labeled with RFP-tagged LAMP1 (LAMP1-RFP) ()), thereby identifying them as enlarged lysosomes. The size of LAMP1-labeled vacuoles increased 9-fold within 20 h as the number of detectable lysosomes decreased by an equivalent amount ()). Western immuno-blotting analysis revealed a comparable increase in the cellular level of endogenous LAMP1 protein (,e)), revealing that WX8 did not prevent lysosome synthesis. Therefore, the accumulation of enlarged lysosomes must have resulted either from increased lysosome fusion, or from decreased lysosome fission.
Figure 2. WX8-induced accumulation of enlarged lysosomes. (a) U2OS cells were cultured in the presence of either vehicle or 2 µM WX8 for 2 h before electron microscopy analysis of thin sections of cell pellets. (b) Cells were transfected with baculovirus expressing LAMP1-RFP and then cultured overnight to label lysosomes before addition of either vehicle or 1 µM WX8 for 4 h. Fluorescence emission from fixed cells was visualized by confocal microscopy. Nuclei were stained with DAPI. (c) The diameters of LAMP1-RFP-labeled vacuoles (lysosomes) were measured using ImageJ (https://imagej.nih.gov/ij/) with lysosomes approximated as circles. The selection of circular, enlarged, lysosomes was carried out using a size mask of 0.5 to 5.0 µm2. Punctate lysosomes were selected with a size mask of 0.1 to 1.5 µm2. Thresholds were the same for all images. Clustered lysosomes were not included in the size analysis. The mean area (µm2) ±SEM was plotted (∘). From 235 to 545 lysosomes were measured for each time point. The number of lysosomes per µm2 (□) was determined at the beginning and end of this experiment and plotted on a separate Y-axis. (d) U2OS cells were seeded into 6-well plates (105 cells/well) and cultured overnight before adding 1 µM WX8 and culturing for the times indicated. LAMP1 protein was identified in total cell lysates by western immuno-blotting using ACTB/β-actin as a loading control. (e) The ratios of LAMP1 to ACTB were determined by densitometry for 2 independent experiments (panel E are •,▪).
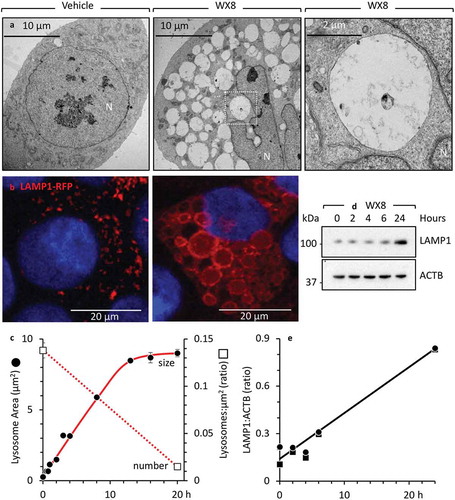
Lysosomal enlargement required homotypic lysosome fusion
The size of cytoplasmic vesicles is the result of a tightly controlled balance between fusion and fission [Citation20]. To determine whether or not lysosomal enlargement involved homotypic lysosome fusion, cells were transfected with a LAMP1-RFP expression vector and then treated with WX8, as in ). Time-lapse imaging of live cells expressing LAMP1-RFP protein revealed that lysosomes were undergoing homotypic fusion in the presence of WX8 (Figure S7 video). For example, in a 3-min segment from this video, a large red lysosomal mass can be seen fusing with an adjacent enlarged lysosome ()). Electron micrographs of thin sections through these cells revealed structures consistent with lysosomes undergoing fusion (,)).
Figure 3. WX8 inhibited lysosomal fission without effecting homotypic lysosomal fusion. (a) U2OS cells expressing LAMP1-RFP were cultured with 1 µM WX8 for 40 min, during which time live cell images were collected. Arrows indicate one example of fusion between 2 lysosomes to produce a larger lysosome. The video is available in Supplemental Materials as Figure S7. (b) U2OS cells were cultured as in panel A, and electron microscopic images of thin sections were taken at 2 h. (c) The section indicated in (B) was enlarged 4X. Arrows indicate sites of lysosome-to-lysosome fusion.
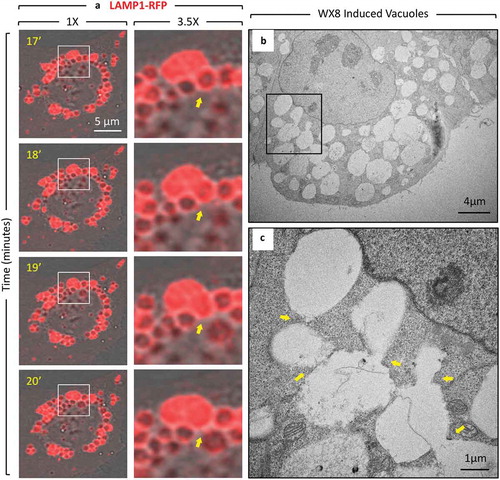
Homotypic fusion between lysosomes and heterotypic fusion between lysosomes and other organelles involves assembly of a trans-SNARE complex and the function of several regulators, including the multi-subunit HOPS and BORC complexes [Citation21–Citation27]. Therefore, to determine whether or not WX8-induced lysosomal enlargement also required these proteins, cells were challenged by 3 different manipulations. First, cells were treated briefly with N-ethylmaleimide (NEM) in order to irreversibly inactivate the NSF (N-ethylmaleimide sensitive factor, vesicle fusing ATPase) required for SNARE complex disassembly and recycling [Citation28,Citation29] (enlargements ,); fields Figure S8). Second, cells were transfected with siRNAs targeting the HOPS subunits VPS39 and VPS41 [Citation21] in order to suppress expression of these subunits before adding either vehicle or WX8 to the culture medium (enlargements ); fields Figure S9). Finally, cells lacking the BORCS5/myrlysin subunit of BORC [Citation21] were cultured in the presence of either vehicle or WX8 (enlargements –l); fields Figure S10). Staining for endogenous LAMP1 showed that all of the above treatments reduced swelling of lysosomes induced by WX8. Taken together, these experiments demonstrated that ongoing lysosome fusion was a prerequisite for WX8-induced lysosome enlargement and concomitant aggregation at the perinuclear area.
Figure 4. Inhibition of homotypic lysosomal fusion prevented WX8-induced lysosomal enlargement. (a-d) HeLa cells were cultured for 10 min in the presence of either vehicle or 10 μM N-ethylmalaimide (NEM) before washing them with phosphate-buffered saline. Fresh medium was then added containing either vehicle or 1 µM WX8, and the cells were cultured for 1 h. Cells were then stained with anti-LAMP1 antibody, a fluorescent-conjugated secondary antibody to identify lysosomes, and DAPI to identify nuclei, as previously described [Citation22]. Fluorescent images were viewed by confocal microscopy. Scale bar: 5 µm. (e-g) HeLa cells were transfected for 2-days, 2 consecutive times, with a pool of siRNAs against the genes encoding the HOPS-specific subunits VPS39 and VPS41, as previously described [Citation22]. Cells were then incubated for 30 min either with vehicle (V) or with 1 μM WX8 (W). Scale bar: 10 µm. (h) Western immuno-blot with non-targeting (nt) siRNA as a negative control, and GAPDH protein as an internal loading control. (i-l) Wild-type HeLa cells and a derivative HeLa cell line in which the BORCS5 gene was ablated were cultured for 30 min in the presence of either vehicle or 1 µM WX8. Scale bar: 10 µm. Bar graphs indicate the mean ±SD for the lysosomal area in 2 independent experiments for each image.
![Figure 4. Inhibition of homotypic lysosomal fusion prevented WX8-induced lysosomal enlargement. (a-d) HeLa cells were cultured for 10 min in the presence of either vehicle or 10 μM N-ethylmalaimide (NEM) before washing them with phosphate-buffered saline. Fresh medium was then added containing either vehicle or 1 µM WX8, and the cells were cultured for 1 h. Cells were then stained with anti-LAMP1 antibody, a fluorescent-conjugated secondary antibody to identify lysosomes, and DAPI to identify nuclei, as previously described [Citation22]. Fluorescent images were viewed by confocal microscopy. Scale bar: 5 µm. (e-g) HeLa cells were transfected for 2-days, 2 consecutive times, with a pool of siRNAs against the genes encoding the HOPS-specific subunits VPS39 and VPS41, as previously described [Citation22]. Cells were then incubated for 30 min either with vehicle (V) or with 1 μM WX8 (W). Scale bar: 10 µm. (h) Western immuno-blot with non-targeting (nt) siRNA as a negative control, and GAPDH protein as an internal loading control. (i-l) Wild-type HeLa cells and a derivative HeLa cell line in which the BORCS5 gene was ablated were cultured for 30 min in the presence of either vehicle or 1 µM WX8. Scale bar: 10 µm. Bar graphs indicate the mean ±SD for the lysosomal area in 2 independent experiments for each image.](/cms/asset/b7317fc0-a897-4bb2-92c1-827657f2849f/kaup_a_1586257_f0004_c.jpg)
Lysosomal enlargement resulted from reversible inhibition of lysosome fission
Cells cultured in the presence of any member of the WX8-family for 24 h accumulated vacuoles (Figure S5A), and when these cells were then transferred to fresh culture medium without the compound present, the vacuoles disappeared (Figure S5B). Moreover, time lapse microscopy of live cells expressing LAMP1-RFP protein demonstrated that the enlarged lysosomes that accumulated in the presence of WX8 as cytoplasmic vacuoles reverted into small fluorescent puncta when cells were transferred to fresh culture medium without WX8 ()). Enlarged lysosomes quickly began undergoing fission into smaller lysosomes (Figure S6 video). Lysosomal motility and size reverted to their original state within 1 h. Multiple events of lysosome tubules budding from single lysosomes could be observed throughout the field of view (,)). When WX8 was removed, the removal of membrane from single enlarged lysosomes in the form of tubules led to the reduction of the size of the lysosome, thereby demonstrating that WX8-family compounds inhibited lysosome fission.
Figure 5. Enlarged lysosomes that formed in the presence of WX8 underwent fission via tubulation when WX8 was removed. (a) U2OS cells expressing LAMP1-RFP were cultured with 0.1 µM WX8 for 2 h. The cells were then washed twice with phosphate-buffered saline before transferring them to fresh culture medium without WX8. Cells were monitored by live-cell imaging for 1 h with one exposure every 3 s (video Figure S6). Images in (b) and (c) start at 13 min and 33:36 min after WX8 removal, respectively, and show magnified time-lapse images of the LAMP1-labeled vesicles present at the times indicated inside the insets and in the whole cells shown in panel A. Arrows facilitate tracking of tubule fate. Scale bar: 20 µm.
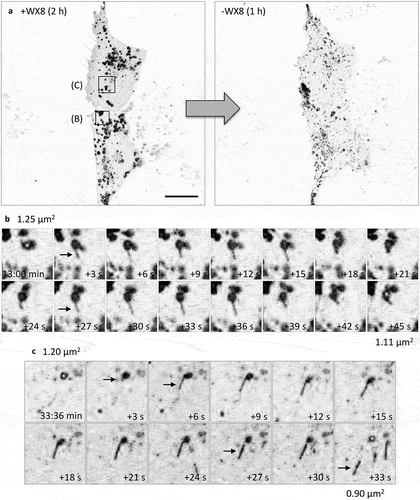
Lysosomal enlargement impaired trafficking not acidification
Lysosomes have an acidic lumen (pH 4.5–5.0) and contain approximately 60 different soluble hydrolases that are active only under these conditions [Citation30]. To determine whether or not lysosomal enlargement induced by the WX8-family altered lysosomal acidification, the pH of individual lysosomes was quantified using a ratiometric protocol. Previous studies using a similar ratiometric method for quantifying lysosomal pH in RAW264.7 cells, a mouse macrophage-like cell line, concluded that the PIKFYVE inhibitor apilimod did not affect normal lysosomal acidity of pH 4.8 [Citation31]. Therefore, to confirm and extend this study to WX8, lysosomes in RAW264.7 cells were preloaded with Oregon Green 488 dextran, and the cells were then cultured in the presence of either 1 µM WX8 for 4 h, or 0.2 µM apilimod for 2 h before measuring the ratio of fluorescence at 2 separate wavelengths. As previously reported, apilimod did not affect lysosomal acidity in RAW264.7 cells, and neither did WX8, although lysosomal pH under our conditions was 5.3 ()). Similar results were obtained with human U2OS osteosarcoma cells, except that lysosomal pH in cells treated with WX8 were slightly more acidic at pH 5 ()). Fluorescent images of the cells in both experiments confir-med that both apilimod and WX8 treated cells contained enlarged lysosomes.
Figure 6. WX8 did not impair lysosomal acidity. (a) Mouse RAW 264.7 cells were cultured as previously described [Citation31], and pre-loaded with Oregon Green Dextran in preparation for ratiometric analysis of the pH of individual lysosomes. Cells were cultured for 4 h with vehicle, 1 µM WX8, or 50 mM ammonium chloride, or for 2 h with 0.2 µM apilimod. The results of 2 independent experiments were averaged together. Data were analyzed by one-way ANOVA and Tukey’s post-hoc test. **p < 0.0001. Bars: 10 µm. (b) Human U2OS cells were pre-loaded with Oregon Green Dextran in preparation for ratiometric analysis of the pH of individual lysosomes. The preloaded cells were then cultured as in (a). The results of 3 independent experiments were averaged together. Data were analyzed as in (a). *p = 0.0005, **p < 0.0001. (c) U2OS cells were cultured for 4 h in the presence of either vehicle or 1 µM WX8, and then live cells were stained with LysoTracker Green DND-26 according to the manufacturer’s instructions and viewed immediately by confocal microscopy. (d) U2OS cells were cultured for 4 h with vehicle (V), WX8 or 50 nM bafilomycin A1 (BafA1). Mature and immature CTSD, LC3-I, LC3-II, and ACTB proteins were identified by western immuno-blotting, under the same conditions, and detected with specific antibodies and by cofractionation with molecular mass markers (kDa).
![Figure 6. WX8 did not impair lysosomal acidity. (a) Mouse RAW 264.7 cells were cultured as previously described [Citation31], and pre-loaded with Oregon Green Dextran in preparation for ratiometric analysis of the pH of individual lysosomes. Cells were cultured for 4 h with vehicle, 1 µM WX8, or 50 mM ammonium chloride, or for 2 h with 0.2 µM apilimod. The results of 2 independent experiments were averaged together. Data were analyzed by one-way ANOVA and Tukey’s post-hoc test. **p < 0.0001. Bars: 10 µm. (b) Human U2OS cells were pre-loaded with Oregon Green Dextran in preparation for ratiometric analysis of the pH of individual lysosomes. The preloaded cells were then cultured as in (a). The results of 3 independent experiments were averaged together. Data were analyzed as in (a). *p = 0.0005, **p < 0.0001. (c) U2OS cells were cultured for 4 h in the presence of either vehicle or 1 µM WX8, and then live cells were stained with LysoTracker Green DND-26 according to the manufacturer’s instructions and viewed immediately by confocal microscopy. (d) U2OS cells were cultured for 4 h with vehicle (V), WX8 or 50 nM bafilomycin A1 (BafA1). Mature and immature CTSD, LC3-I, LC3-II, and ACTB proteins were identified by western immuno-blotting, under the same conditions, and detected with specific antibodies and by cofractionation with molecular mass markers (kDa).](/cms/asset/451bdeed-5bf8-45c6-b802-0d4ce4aad6d3/kaup_a_1586257_f0006_c.jpg)
To confirm these results, lysosomal acidity was evaluated in WX8 treated U2OS cells by several additional methods. LysoTracker Green DND-26, a fluorescent probe commonly used for staining acidic compartments in live cells [Citation32], gave results consistent with those obtained by ratiometric analysis ()). Because the pKa for LysoTracker was not available, U2OS cells were also stained with LysoSensor Green DND-189, a fluorescent probe with a pKa~5.2 that fluoresces only in the acidic compartments of live cells. As expected, LysoSensor Green produced green puncta throughout the cytoplasm of cells cultured with vehicle that were largely coincident with LAMP1-RFP labeled puncta in the same cells, but not in cells treated with bafilomycin A1, a specific inhibitor of the vacuolar-type H+-translocating ATPase essential for lysosome acidification [Citation33] ()). However, LysoSensor Green did not produce green puncta in cells treated with WX8. Similar results were obtained with BODIPY FL-pepstatin A ()), a green-fluorescent probe that stains lysosomes in live cells by binding selectively to mature CTSD (cathepsin D) at pH 4.5 [Citation34]. These results suggested that WX8-induced lysosomal enlargement accompanied by lysosomal deacidification.
Figure 7. WX8 impaired lysosomal trafficking. U2OS cells were cultured for 4 h in the presence of either vehicle, 1 µM WX8 or 50 nM bafilomycin A1 (BafA1), and then live cells were stained as indicated according to the manufacturer’s instructions and viewed immediately by confocal microscopy. (a) Cells were stained with acridine orange by seeding them into chambered tissue culture slides, adding either vehicle or WX8 the following day and then culturing for 4 h before medium was removed, cells washed once with PBS, then covered with 1 mg/ml acridine orange in PBS and imaged by confocal microscopy at 40X. (b) Cells that had been transfected with LAMP1-RFP expression vector were then stained with LysoSensor Green DND-189 according to the manufacturer’s instructions. (c) Cells that had been transfected with LAMP1-RFP expression vector were then stained with BODIPY-FL-Pepstatin-A, and then fixed 15 min later, according to the manufacturer’s instructions. Nuclei were stained with DAPI, and cells were visualized by confocal microscopy. Bars: 20 µm.
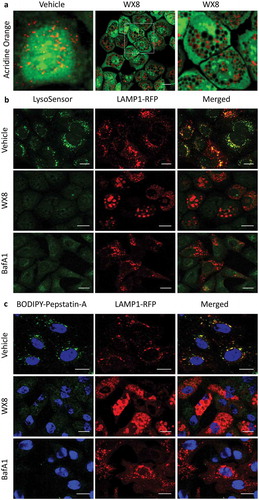
A simple explanation for this conundrum arose when cells were stained with acridine orange. In acidic compartments, acridine orange emits orange fluorescence, whereas in neutral pH environments, it emits green fluorescence [Citation35]. Acridine orange also emits green fluorescence when bound to double-stranded DNA and orange fluorescence when bound to single-stranded DNA or RNA. Thus, in cells cultured with the vehicle, nuclear DNA appeared as bright intense green and cytoplasm appeared as light speckled green, but lysosomes were detected as bright orange puncta ()). In stark contrast, some of the vacuoles in cells treated with WX8 stained orange, but most of them were devoid of any fluorescence. Because the pKa of acridine orange is 9.65, it should fluoresce in cells wherever it accumulates. However, the metachromatic shift observed when acridine orange accumulates in vesicles results from its increased concentration rather than a decrease in pH [Citation35]. Therefore, the accumulation of dark vacuoles revealed that acridine orange was eventually excluded from lysosomes as they continued to undergo homotypic fusion in the absence of fission. This exclusion mechanism likely accounted for the absence of lysosome staining by either LysoSensor or BODIPY FL-pepstatin A.
To determine whether or not the WX8-family affected enzymatic activities in lysosomes, the distribution of CTSD was examined before and after treatment of U2OS cells with WX8 ()). CTSD is synthesized on the rough endoplasmic reticulum as a pre-pro-enzyme that undergoes several proteolytic cleavages during biosynthesis to produce the mature form [Citation36]. The 52-kDa pro-CTSD is targeted to lysosomes, where it is cleaved into a mature enzyme consisting of 2 chains, 34 kDa and 14 kDa in size. The cellular levels of mature CTSD in WX8-treated cells were about 50% of vehicle treated cells, suggesting that WX8 impaired trafficking of pro-CTSD into lysosomes.
The WX8-family induced accumulation of autophagosomal biomarkers
Autophagy begins with the formation of a double-membrane structure that engulfs cargoes such as mitochondria, peroxisomes, ribosomes, and protein aggregates to form autophagosomes, a process requiring approximately 10 min [Citation37]. LC3-II is recruited to phagophore membranes, and SQSTM1 is an autophagy receptor that interacts directly with LC3-II as well as with ubiquitinated proteins. Autophagosomes survive for 10 to 25 min [Citation38,Citation39] before fusing with lysosomes to form autolysosomes, which proceed to degrade the cargo. The entire process, termed autophagic flux, is active throughout the cell cycle [Citation40].
Under the same conditions in which the WX8-family induced the accumulation of cytoplasmic vacuoles, western immuno-blotting analysis revealed a concentration-dependent increase in the amount of LC3-II and SQSTM1, as illustrated using WX8 (,)), as well as a time-dependent accumulation of LC3-II and SQSTM1 in which SQSTM1 accumulation was delayed about 3 h relative to LC3-II (,)). These results were consistent with the accumulation of autophagosomes. However, whereas 80% of the cells were vacuolated by 4 h in 0.01–0.1 µM WX8, near maximum levels of LC3-II accumulation required 0.1–0.5 µM WX8 (). Thus, inhibition of lysosome fission was at least 5-fold more sensitive to WX8 than accumulation of autophagosomes. Nevertheless, the accumulated LC3-II and SQSTM1 proteins, like accumulated enlarged lysosomes, returned to their baseline values within 24 h when WX8-family compounds were removed (Figure S5(d)), demonstrating that the ability of the WX8-family to induce accumulation of autophagosomes, like their ability to induce accumulation of enlarged lysosomes, was readily reversible.
Figure 8. The WX8-family induced accumulation of autophagosome biomarkers. (a) U2OS cells were seeded in 6-well plates (0.7 x 105/well) and 24 h later added the indicated compound to give the indicated concentration (0 µM WX8 received vehicle alone). Cells were cultured for 4 h before total cell extracts were subjected to western immuno-blotting for LC3, SQSTM1 and ACTB/β-actin. Blot was developed with film. (ai) The ratios of LC3-II:ACTB and SQSTM1:ACTB in panel A for cells treated with vehicle alone (0 µM WX8) were subtracted from same ratios in samples treated with WX8. The results were normalized to the maximum ratio. Ratio in cells treated with vehicle (0 µM WX8) was plotted as 0.001 µM WX8 in order to apply a logarithmic scale. (b) U20S cells were cultured for the times indicated in the presence of 1.2 µM WX8 to produce 80% of the maximum observed in panel A. Total cell extracts were assayed at the times indicated. (bi) The ratios of LC3-II:ACTB and SQSTM1:ACTB in panel B were plotted as in panel Ai. (c) Western immuno-blots of LC3 protein present in total cell extracts at 4 h and at 8 h of culture in the presence of the indicated WX8-family member. The ratios of LC3-II:ACTB were plotted (Figure S4(a)). The 4 h samples were developed using the Azure c600 imaging system. The 8-h samples were developed with film. All blots were stained with Ponceau S to visualize the ACTB loading control.
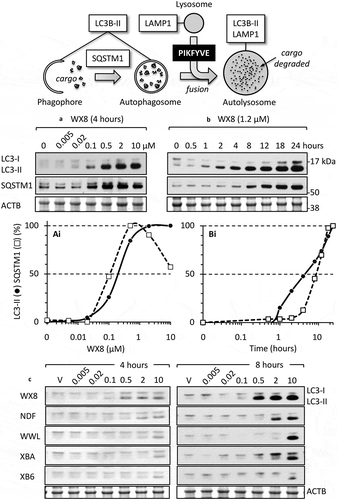
The WX8-family prevented heterotypic fusion of lysosomes with autophagosomes
In addition to enlarged lysosomes, electron microscopy of cells treated briefly with WX8-family compounds revealed vesicular structures consistent with early and late stages in autophagy (Figure S11(a–c)). Moreover, immuno-fluorescence microscopy revealed the presence of autophagosomes containing ubiquitinated cargos. LC3 puncta were evident either by staining cells with LC3 antibody (,)), or by ectopic expression of GFP-tagged LC3 (,)), and WX8 treated cells contained about 5-times the number of LC3 puncta than cells treated with vehicle. Moreover, cells ectopically expressing both GFP-tagged LC3 and RFP-tagged SQSTM1 accumulated both green and red puncta that colocalized to produce yellow puncta (,)).
Figure 9. WX8-induced accumulation of autophagosomes. (a) U2OS cells were cultured in the presence of either vehicle or 1 µM WX8 for 4 h, and then endogenous autophagosomes were identified by staining cells with fluorescent tagged anti-LC3 antibody. (b) U2OS cells were transfected with a baculovirus expressing GFP-LC3 and then culturing them overnight before addition of either vehicle or 1 µM WX8. Fluorescence was analyzed by confocal microscopy 4 h later. (AI, BI) LC3 and GFP-LC3-labeled puncta per cell were quantified in 50 cells from each of 3 independent experiments (mean ±SEM; Student’s t-test, p < 0.0001). Both LC3 and GFP-LC3-labeled puncta increased 6- to 8-fold in the presence of WX8. (c) Autophagosomes containing cargo were identified by co-transfecting U2OS cells with 2 separate baculovirus expression vectors, one for GFP-LC3 and the other for RFP-SQSTM1. Cells were then cultured overnight before addition of either vehicle or 1 µM WX8. Fluorescence was analyzed by confocal microscopy 4 h later. (d) Using the same protocol as in panel C, U2OS cells expressing tandemly labeled RFP-GFP-LC3B protein were treated with either vehicle or 1 µM WX8 for 4 h. (ci, di) The fraction of yellow puncta was quantified in 50 cells from each of 3 independent experiments (mean ±SEM; Student’s t-test, p < 0.0001). The fraction of yellow puncta increased 4-fold in the presence of WX8.
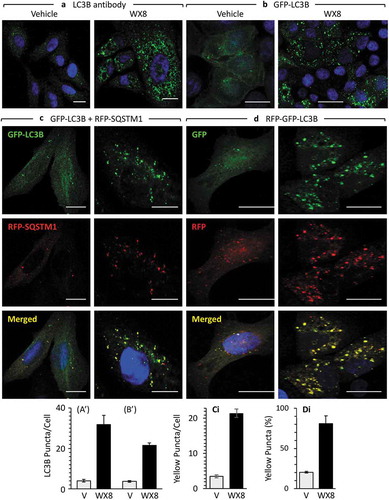
To determine whether the accumulation of autophagosomes resulted from induction of autophagy or from disruption of autophagic flux, cells were transfected with a vector expressing a modified tandem sensor RFP-GFP-tagged LC3B protein In which the green signal from a mutated GFP was suppressed in an acidic environment, with little or no effect on the red signal from RFP [Citation41]. Therefore, in merged images, only red puncta indicated acidic autolysosomes; yellow puncta indicated either autophagosomes or non-acidic autolysosomes. Thus, normal autophagic flux is characterized by the presence of both red and yellow puncta, whereas disruption of autophagic flux produces yellow puncta.
When cells were cultured with WX8, the fraction of yellow puncta increased 4-fold. Both red and yellow puncta were evident in cells treated with vehicle and in cells treated with WX8, but when the colors were merged at least 80% of the puncta in cells treated with WX8 were yellow (,)). Therefore, WX8-family compounds disrupted autophagic flux by preventing formation of an acidic environment.
To determine whether or not the absence of an acidic environment resulted from failure of lysosomes to fuse with autophagosomes, cells were transfected with both GFP-LC3 and LAMP1-RFP expression vectors, and then cultured for 4 h in the presence of vehicle, rapamycin, or both rapamycin and WX8 (). Rapamycin is an established inducer of autophagy [Citation42,Citation43]. As expected, rapamycin induced accumulation of GFP-LC3 labeled autophagosomes (green), some of which were autolysosomes, as shown by colocalization with LAMP1-RFP on control cells. In contrast, GFP-LC3 and LAMP1-RFP labeled different populations of vesicles in cells cultured with either WX8 or rapamycin and WX8, despite the fact that the density of autophagosomes was at least 3-fold greater. Taken together, these experiments demonstrated autophagosomes accumulated in the presence of WX8, because they failed to fuse with lysosomes.
Figure 10. WX8 prevented formation of autolysosomes. U2OS cells were co-transfected with GFP-LC3 and LAMP1-RFP expression vectors. Cells were then treated for 4 h with 1 µM rapamycin to induce autophagy, or with 1 µM WX8 to disrupt autophagy, or with 1 µM rapamycin plus 1 µM WX8. Cells were then fixed and viewed by confocal microscopy. Nuclei were stained with DAPI. The fractions of yellow puncta in merged images of 25 cells is given as the mean ±SEM for 3 independent experiments.
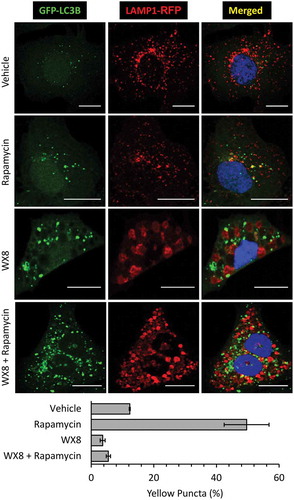
WX8-family and bafilomycin A1 have different mechanisms
Bafilomycin A1 (BafA1) is a specific inhibitor of the V-ATPase that disrupts autophagic flux by inhibiting both lysosome acidification and fusion of autophagosomes with lysosomes to form autolysosomes, 2 properties that are shared by the WX8-family, but BafA1 does not induce accumulation of large vacuoles [Citation44,Citation45].
Therefore, to compare WX8-compounds directly with BafA1, GFP-LC3 and LAMP1-RFP proteins were expressed in U2OS cells, and the cells were then cultured in the presence of BafA1, WX8, or both BafA1 and WX8 ()). The results confirmed that BafA1 did not induce formation of enlarged lysosomes, and that neither BafA1 nor WX8 induced accumulation of autolysosomes. However, the LC3 puncta that accumulated in the presence of WX8 were distinctly larger than those that accumulated in the presence of BafA1, suggesting that WX8 promoted aggregation of autophagosomes ()). Electron microscopy of cells cultured in both WX8 and BafA1 revealed double-membrane autophagosomes (Figure S11(f)) as well as small empty single-membrane vacuoles ~0.1 µm in diameter (Figure S11(d,e)), characteristic of lysosomes [Citation46].
Figure 11. The vacuolar-type H+-ATPase was required for accumulation of enlarged lysosomes. (a) U2OS cells cultured as in to label both LC3 and LAMP1 proteins, and then the cells were cultured in the presence of vehicle, 50 nM bafilomycin A1 (BafA1), 1 µM rapamycin (Rap), 1 µM WX8, or BafA1 plus WX8 for 4 h before viewing with confocal microscopy. (b) Phase contrast images (20X) of cells treated with vehicle, BafA1, WX8, or WX8 plus BafA1 for 4 h. Rap treated cells were indistinguishable from vehicle treated cells. Bars: 20 µm. (c) U2OS cells were cultured for 24 h in the presence of vehicle, 50 nM bafilomycin A1 (BafA1), 1 µM rapamycin (Rap), or Rap plus BafA1, 1 µM WX8, or WX8 plus BafA1. Total cellular LC3 protein was analyzed by western immuno-blotting with ACTB protein as a loading control.
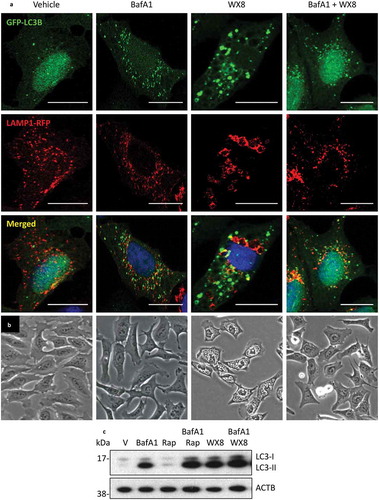
To evaluate this hypothesis, the cellular levels of LC3 protein in BafA1- and WX8-treated cells were compared. BafA1 induced the accumulation of LC3-II above the level observed either in control cells or in cells treated with rapamycin. A comparable increase in LC3-II was induced by WX8. However, addition of BafA1 to cells cultured in the presence of WX8 did not significantly alter the level of LC3-II ()). Thus, both BafA1 and WX8-compounds caused accumulation of autophagosomes, but only WX8-compounds caused aggregation of autophagosomes and accumulation of enlarged lysosomes.
BafA1 also has been shown to prevent formation of cytoplasmic vacuoles that are induced by inhibition of the PIKFYVE phosphoinositide kinase [Citation47]. To determine whether or not BafA1 also prevented induction of the cytoplasmic vacuoles that are induced by the WX8-family, U2OS cells were cultured in the presence of BafA1, WX8, or both BafA1 and WX8 ()). The results revealed that BafA1 prevented induction of vacuole accumulation by the WX8-family, suggesting that the WX8-family inhibited PIKFYVE activity.
The WX8-family selectively inhibited the PIKFYVE phosphoinositide kinase
The ability of BafA1 to prevent the WX8-family from inducing accumulation of enlarged lysosomes, and the similarity of molecules in ) to molecules that bind protein kinases [Citation48] suggested that the WX8-family might inhibit phosphatidylinositol kinases. To address this hypothesis, a panel of 468 human kinases were screened for their ability to bind WX8. In the presence of 10 µM WX8, 10 candidates had binding affinities for WX8 of 25% or greater ()). The top 2 candidates were PIKFYVE (phosphoinositide kinase, FYVE-type zinc finger containing) and PIP4K2C (phosphatidylinositol-5-phosphate 4-kinase type 2 gamma). PIKFYVE is a kinase that is essential for mouse development [Citation49] and that prevents endosome enlargement and cytoplasmic vacuolization [Citation50,Citation51]. PIP4K2C is a kinase that is not essential for mouse growth and viability, but is essential for the immune system [Citation52], MTORC1 signaling [Citation53], and regulation of autophagy [Citation54]. The third candidate was MTOR, a kinase that regulates autophagy [Citation55]. Therefore, the binding constants (Kd) for all 5 members of the WX8-family were determined for each of these 3 proteins from 2 independent titration curves, as shown for WX8 ()). The results ()) revealed that WX8 bound PIKFYVE 340 times better than PIP4K2C and 7200 times better than MTOR. The Kd values with PIKFYVE for all 5 molecules ranged from 1 nM (WX8) to 16 nM (XBA), and the binding of all 5 molecules to PIKFYVE was ~300 times greater than to PIP4K2C and ~7000 times greater than to MTOR. Therefore, of 468 human kinases, the entire WX8-family of molecules bound specifically to PIKFYVE, with WX8 as the most potent member, and NDF the most specific.
Figure 12. The WX8-family bound specifically to the PIKFYVE phosphoinositide kinase protein. (a) The binding affinity of 10 µM WX8 was profiled against 468 human kinases by DiscoverX KINOMEscan (San Diego, CA). Compounds that bind to the active site of a protein kinase prevented the protein from binding to an immobilized active site ligand, thereby reducing the amount of protein captured on a solid support. Each kinase was tagged with a unique DNA sequence that allowed the amount of protein bound to the solid support to be quantified by PCR. (b) The mean equilibrium dissociation constant (Kd) for WX8 with PIKFYVE, PIP4K2C and MTOR was determined from 2 independent titration curves. A dissociation constant (Kd) was calculated by measuring the amount of kinase captured on the solid support as a function of the test compound concentration (nM) on a log10 scale. (c) Mean Kd values (±range) are given for each WX8-family member. The ratio of PIP4K2C to PIKFYVE indicates relative specificity for these 2 kinases.
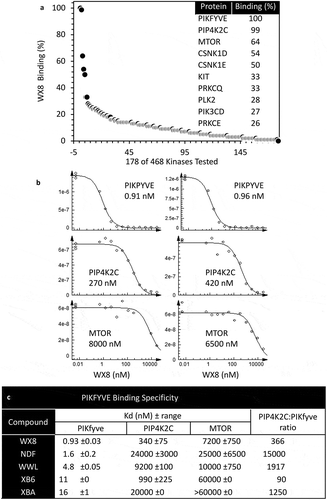
To determine which kinase was responsible for the effects of WX8, U2OS cells were treated with siRNAs targeting either PIKFYVE or PIP4K2C. Although expression of both PIKFYVE ()) and PIP4K2C ()) were strongly suppressed, only siRNAs against PIKFYVE were equivalent to WX8 at promoting LC3-II accumulation (,b)) and inducing cytoplasmic vacuolization ()). In fact, siPIP4K2C caused U2OS cells to detach from the plate and shrink in size. Those cells that remained attached contained no more vacuoles than observed using ‘no target’ siRNA. Treatment of U2OS cells with rapamycin, a specific inhibitor of MTOR activity [Citation56], also did not induce vacuolization ()), and it did not cause cells to detach and die, although it did inhibit their proliferation.
Figure 13. The WX8-family mimicked the effects of suppressing PIKFYVE. U2OS cells were cultured overnight, and then transfected for 7 h with 50 pmol siRNA targeted against either PIKFYVE (a) or PIP4K2C (b) mRNA according to the manufacturer’s instructions. Cells were then cultured for 36 h and then total cell extracts were subjected to western immuno-blotting. As a control, cells were also cultured in the presence of 0.1 µM WX8 or 1 µM rapamycin for the same length of time. (c) At end of 36 h, the extent of vacuolization was determined by phase contrast microscopy (40X). (d) To determine whether or not the WX8-family mimicked the MTOR kinase-specific inhibitor rapamycin, U2OS cells were cultured for 24 h in the presence of vehicle (V), or the indicated concentrations of rapamycin (Rap), WX8, NDF, WWL, or XB6. Whole cell extracts were then subjected to western immuno-blotting for LC3, SQSTM1, RPS6 (ribosomal protein S6) and its phosphorylated form (p-RPS6). ACTB was included as a loading control. The positions of molecular mass markers are indicated (kDa).
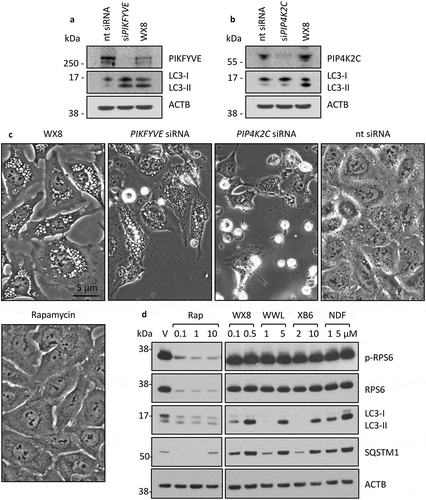
The MTOR signal transduction pathway activates the protein kinase RPS6KB1, which phosphorylates RPS6 protein and suppresses translation of the RPS6 gene [Citation57]. As expected, rapamycin inhibited expression of both RPS6 and phosphorylated RPS6 (p-RPS6) ()), thereby confirming that although rapamycin did not induce vacuolization, it did inhibit MTOR activity. In contrast, none of the WX8-family compounds affected the levels of either RPS6 or p-RPS6, whereas they did induce accumulation of LC3-II and SQSTM1. Therefore, the WX8-family did not inhibit MTOR activity. Taken together, these results demonstrated that the WX8-family primarily, if not exclusively, disrupted lysosome homeostasis by inhibiting PIKFYVE activity.
The WX8-family selectively killed autophagy-dependent cancer cells
The multiple disruptions of lysosome homeostasis induced by the WX8-family suggested that these-compounds would disrupt autophagy in cancer cells. To test this hypothesis, the effects of WX8 on the vacuolization, proliferation and viability of melanoma A375 cells were compared with the effects of the lysosomal inhibitors hydroxychloroquine (HCQ) and chloroquine (CQ). Melanoma A375 cells are homozygous for the BRAFV600E mutation and have been termed ‘autophagy-addicted’, because ablation of genes essential for autophagy in models of BRAFV600E-driven cancer impairs mitochondrial metabolism and increases the survival of BRAFV600E tumor-bearing mice [Citation7,Citation58]. Consequently, A375 cells require autophagy for cell growth, proliferation, and viability even when cultured in a rich medium, as evidenced by its sensitivity to hydroxychloroquine (HCQ) and chloroquine (CQ) [Citation59].
Cells were seeded at a low density (250 cells/cm2) to insure multiple rounds of proliferation. As expected, WX8-induced extensive cytoplasmic vacuolization in A375 cells, an event not observed with either HCQ or CQ (). However, all 3 compounds inhibited A375 cell proliferation, as evident from plate assays ()), although WX8 was at least 100-times more effective than either HCQ or CQ. Moreover, WX8 was at least 100-times more lethal than either HCQ or CQ, as evident from the release of cells into the medium within 2 d ()), the loss of cellular ATP within 4 d ()), and the loss of both viable and non-viable cells within 7 d, as identified by staining with trypan blue ()). These results confirmed that A375 cell viability not only required functional lysosomes, but they were exquisitely sensitive to compounds that disrupted multiple events in lysosome homeostasis.
Figure 14. WX8 selectively killed ‘autophagy-addicted’ melanoma cells. Human melanoma A375 cells were seeded into 12-well plates (1,000 cells/well) and cultured for 1-day before adding WX8, hydroxychloroquine (HCQ), or chloroquine (CQ) to give the concentrations indicated. (a) Cells were photographed 2 d later. (b) Seven days after WX8, HCQ or CQ was added, adherent cells were stained with crystal violet [Citation104]. (c) Cells were seeded into 96-well plates (1,000 cells/well), and WX8, HCQ or CQ was added the following day to give the indicated concentration. Cells were cultured for 4 d before quantifying total cellular ATP using the CellTiter-Glo luminescent cell viability assay (Promega, G7572) according to the manufacturer’s instructions. (d) In separate 12-well plates, attached cells were collected by trypsinization, combined with unattached cells, and stained with trypan blue to identify dead cells. Total live cells in each well were plotted as a percentage of the number of live cells in the vehicle control. Vehicle (0 µM WX8) was plotted as 0.001 µM WX8 in order to apply a logarithmic scale. (e) The half maximal inhibitory concentration (IC50) was the concentration (µM) of WX8, HCQ and CQ that produced a 50% reduction in either cell proliferation (panels B, C) or viability (panel D). All data were plotted as the mean ±SEM for 3 independent experiments. Results for human U2OS (Figure S12), 293T (Figure S13) and HFF (Figure S14) cells are provided as supplementary figures. Assays were performed in duplicate and each experiment was repeated a minimum of 3 times to obtain average EC50 values.
![Figure 14. WX8 selectively killed ‘autophagy-addicted’ melanoma cells. Human melanoma A375 cells were seeded into 12-well plates (1,000 cells/well) and cultured for 1-day before adding WX8, hydroxychloroquine (HCQ), or chloroquine (CQ) to give the concentrations indicated. (a) Cells were photographed 2 d later. (b) Seven days after WX8, HCQ or CQ was added, adherent cells were stained with crystal violet [Citation104]. (c) Cells were seeded into 96-well plates (1,000 cells/well), and WX8, HCQ or CQ was added the following day to give the indicated concentration. Cells were cultured for 4 d before quantifying total cellular ATP using the CellTiter-Glo luminescent cell viability assay (Promega, G7572) according to the manufacturer’s instructions. (d) In separate 12-well plates, attached cells were collected by trypsinization, combined with unattached cells, and stained with trypan blue to identify dead cells. Total live cells in each well were plotted as a percentage of the number of live cells in the vehicle control. Vehicle (0 µM WX8) was plotted as 0.001 µM WX8 in order to apply a logarithmic scale. (e) The half maximal inhibitory concentration (IC50) was the concentration (µM) of WX8, HCQ and CQ that produced a 50% reduction in either cell proliferation (panels B, C) or viability (panel D). All data were plotted as the mean ±SEM for 3 independent experiments. Results for human U2OS (Figure S12), 293T (Figure S13) and HFF (Figure S14) cells are provided as supplementary figures. Assays were performed in duplicate and each experiment was repeated a minimum of 3 times to obtain average EC50 values.](/cms/asset/3e835394-8d8a-457a-8cce-e4c5900376c4/kaup_a_1586257_f0014_b.gif)
The osteosarcoma U2OS cells used to characterize WX8-compounds in this study do not harbor a BRAF or KRAS mutation [Citation60], and they have not been reported to be autophagy-dependent. Nevertheless, HCQ and CQ inhibited U2OS cell growth and proliferation as well as viability (); S13) without inducing vacuolization, and WX8 was 21-times more effective than either HCQ or CQ at inhibiting U2OS cell proliferation, and 13-times more effective at reducing viability. In contrast to both cancer cell lines, the EC50 values for WX8, HCQ or CQ on either immortalized human embryonic kidney 293T cells (Figure S13) or human foreskin fibroblasts HFF (Figure S14) were greater than 10 µM ()). Thus, although WX8-induced comparable levels of vacuolization in each of these 4 cell lines, A375 cells were 1000X more sensitive to WX8 than were these non-malignant cell lines, and U2OS cells were 50X more sensitive, thereby providing proof-of-principle that the WX8-family has therapeutic potential in the treatment of autophagy-dependent cancers.
Discussion
The goal of the present study was to characterize a family of 5 chemical analogs (the WX8-family) that were discovered in a high throughput screen for molecules that inhibit proliferation selectively in human cancer cells. These molecules shared in common the ability to rapidly and reversibly induce accumulation of enlarged lysosomes (cytoplasmic vacuoles) with an efficacy that was compound, concentration, and time dependent. They also bound specifically to the PIKFYVE phosphoinositide kinase.
Selective inhibition of PIKFYVE phosphoinositide kinase
PIKFYVE is a lipid kinase targeted to the cytoplasmic face of endosomal membranes via interactions between the FYVE domain and phosphatidylinositol-3-phosphate (PtdIns3P). PIKFYVE phosphorylates PtdIns3P to generate phosphatidylinositol 3,5-biphosphate (PtdIns [Citation3,Citation5]P2), and plays a crucial role in the regulation of endosome trafficking [Citation61–Citation63]. Screening a panel of 468 human kinases against 10 µM WX8 identified PIKFYVE as the primary target. Subsequent determination of the dissociation constant for each of the WX8-family members revealed that PIKFYVE was the only high-affinity target among this group. The ability of the WX8-family to induce accumulation of large cytoplasmic vacuoles was consistent with previous reports of PIKFYVE inhibition by specific chemicals, by siRNA, and by PIKFYVE mutations [Citation47,Citation62–Citation66]. Reduction of WX8-family induced vacuolization in the presence of BafA1, an inhibitor of the vacuolar-type ATPase, further confirmed that the WX8-family were ‘PIKFYVE inhibitors’ [Citation47].
Two previously reported PIKFYVE inhibitors are members of the WX8-family. Vacuolin and WX8 share in common the 1,3,5-triazin-2-amine core with a morpholine adduct at position 6, and apilimod and NDF share in common the 3-methylbenzaldehyde (6-morpholin-4-ylpyrimidin-4-yl)hydrazine core illustrated in ). A third PIKFYVE inhibitor, YM201636 is only distantly related to the WX8-family in that it shares the 1,3-diazine ring core with a morpholine adduct at position 4; the adducts at position 2 and 5,6 are quite different. As expected, all 3 of these PIKFYVE inhibitors were effective at inhibiting proliferation of melanoma A375 cells, although they differed in efficacy by 30-fold (Figure S15). The fact that WX8 was twice as effective as its analog vacuolin demonstrates that this family of 8 PIKFYVE inhibitors provides an excellent basis on which to develop drugs that can selectively arrest or kill autophagy-dependent cancer cells, with little or no effect on normal cells.
However, the differences that exist in their chemical structures suggest that off-target effects are likely to exist, some of which might affect either toxicity or potency. For example, WX8-induced equivalent levels of vacuolization in U2OS, A375 and HFF cells, although its effect on their proliferation and viability differed significantly. The exact mechanism by which each PIKFYVE inhibitor selectively arrests proliferation or terminates the viability of specific types of cancer cells without harming non-malignant cells, and the efficacy by which they do so, remain to be elucidated.
Disruption of lysosome homeostasis
Previous studies have shown that inhibition of PIKFYVE by either chemical or genetic means results in the accumulation of large cytoplasmic vacuoles, identified in various reports as endosomes [Citation67–Citation69], exosomes [Citation70,Citation71], lysosomes [Citation63,Citation72,Citation73], or autophagosomes [Citation62,Citation74]. Thus, PIKFYVE appeared to play multiple roles in membrane vesicle trafficking. The results presented here show that the WX8-family of PIKFYVE inhibitors disrupts 3 specific events in lysosome homeostasis. (1) They inhibit lysosome fission but not homotypic lysosome fusion, thereby causing accumulation of enlarged lysosomes and preventing lysosome turnover. (2) They impair trafficking of molecules into lysosomes, thereby impairing lysosome function, although they do not decrease lysosome acidity. (3) They prevent heterotypic fusion between lysosomes and autophagosomes, thereby preventing degradation of autophagic cargoes.
With WX8, vacuoles could be detected by light microscopy in U2OS cells within 20 min, accumulation of LC3-II and SQSTM1 within 4 h, and inhibition of cell proliferation within 24 to 48 h. The vacuoles identified here were subsequently shown to be enlarged lysosomes that did not fuse with autophagosomes. Moreover, they resulted from lysosome fusion in the absence of lysosome fission, and the enlarged lysosomes differed from normal lysosomes in their ability to restrict entry of some molecules, but not others. Thus, lysosome homeostasis was disrupted by these molecules at 3 specific events: lysosome fission, traffic into lysosomes, and fusion with autophagosomes. All 3 disruptions were detected with each of the 5 members of the WX8-family, and their relative potencies at inducing formation of large cytoplasmic vacuoles, accumulation of LC3-II and SQSTM1, arrest of cell proliferation, and loss of cell viability were WX8>NDF>WWL>XB6 or XBA ()).
Selective inhibition of lysosome fission and heterotypic lysosome fusion
Lysosome homeostasis depends on continued homotypic lysosome fusion and fission [Citation20]. The results presented here show that PIKFYVE is essential for lysosome fission, but not for homotypic lysosome fusion. This finding accounts for the widely reported observation that inhibition of PIKFYVE resulted in the accumulation of enlarged cytoplasmic vacuoles. The results presented here further show that PIKFYVE is also essential for fusion between lysosomes and autophagosomes. This result is consistent with previous studies showing that heterotypic lysosome fusion events require a delicate balance between PtdIns3P and PtdIns(3,5)P2 on the lysosomal membrane, a balance that is established by the PIKFYVE phosphoinositide kinase and INPP5E (inositol polyphosphate-5-phosphatase E) [Citation75,Citation76]. Notably, the requirement for PIKFYVE in heterotypic lysosome fusion was not overcome by the fact that impaired lysosome fission rapidly filled the cytoplasm with enlarged lysosomes, thereby increasing contact between lysosomes and other membrane vesicles.
Inhibition of lysosome fission by WX8-family compounds was evident from the fact that LAMP1 synthesis was not disrupted, but the size of LAMP1-labeled vacuoles increased dramatically, with a concurrent decrease in the number of lysosomes (). Live cell imaging revealed that lysosomes fused together in the presence of a WX8-family compound to produce enlarged lysosomes ( and S7), and then dissolve into masses of puncta when the compound was removed (Figure S5). High-resolution live cell imaging revealed individual lysosomes undergoing fission via tubulation upon removal of WX8 ( and S6). Lysosome tubules serve as a platform for lysosome reformation, a process through which membrane lipids and proteins are recycled via membrane fission [Citation77]. These results are consistent with a role for PtdIns(3,5)P2 (the product of PIKFYVE activity) in lysosome tubulation [Citation78].
Inhibition of lysosome fusion to autophagosomes was evident from the fact WX8-compounds rapidly induced accumulation of autophagosomal markers LC3-II and SQSTM1 (), and LC3 and SQSTM1 colocalized as cytoplasmic puncta (). Electron microscopy confirmed the accumulation of vesicular structures consistent with early and late stages in autophagy (Figure S11). Cells transfected with a vector expressing a modified tandem sensor RFP-GFP-tagged LC3B protein in which the green signal from a mutated GFP was suppressed in an acidic environment revealed that autophagosomes did not mature into autolysosomes in the presence of WX8 (), and cells transfected with both GFP-LC3 and LAMP1-RFP expression vectors revealed that the absence of an acidic environment resulted from failure of lysosomes to fuse with autophagosomes ().
Previous studies have shown that heterotypic lysosome-to-autophagosome fusion requires NSF, the HOPS complex, and the BORC complex [Citation21,Citation79]. The N-ethylmaleimide sensitive factor (NSF) is a AAA ATPase that promotes disassembly of trans-SNARE complexes for reutilization of the SNAREs in further rounds of fusion [Citation80]. HOPS is essential to tether the opposing membranes and orchestrate SNARE-dependent fusion, and BORC is required for recruitment of HOPS to lysosomes and for lysosome positioning [Citation81]. Each of these components was required for WX8-induced lysosome enlargement (). These results are consistent with recently published studies showing that the number of lysosomes is reduced within 1 to 2 h of addition of either YM201636 [Citation82] or apilimod [Citation83] as they fuse together to form enlarged lysosomes. These results extended previous studies by showing that lysosomal enlargement required components of the SNARE fusion machinery that are essential for both homotypic and heterotypic lysosome fusion events.
Although PIKFYVE was not essential for homotypic lysosome fusion, PIKFYVE was essential for heterotypic fusion of lysosomes to autophagosomes, and perhaps lysosome-to-endosome fusion, as well [Citation62]. However, inhibition of autolysosome formation by the WX8-family required approximately 10-times higher concentrations than required to prevent lysosome fission. Nevertheless, specific inhibition of PIKFYVE by the WX8-family still occurred at these concentrations ()) and resulted in the accumulation of LC3-II and SQSTM1 (), as previously reported using other methods for PIKFYVE inhibition [Citation62,Citation64,Citation84,Citation85]. Furthermore, colocalization of fluorescent-tagged LC3 and SQSTM1 proteins in situ confirmed the accumulation of autophagosomes in the absence of autolysosomes (). The absence of autolysosomes was further demonstrated by the accumulation of a tandem RFP-GFP-LC3B protein in which the pH sensitive GFP remained unquenched, thereby confirming a nonacidic environment, and by the fact that LC3-labeled puncta did not colocalize with LAMP1-labeled enlarged lysosomes (). Similar results have also been reported for vacuolin [Citation84].
Lysosomal acidification versus lysosomal trafficking
Previous studies on the importance of PIKFYVE in maintaining lysosomal acidity resulted in apparently contradictory conclusions. Some studies concluded that PIKFYVE was not essential to maintain lysosomal acidity either in yeast or in RAW264.7 cells, a macrophage-like mouse cell line [Citation31,Citation86], whereas other studies concluded that PIKFYVE was required to maintain lysosome acidification in rat neuronal dendrites [Citation87], mouse fibroblasts [Citation65] and human HeLa cervical adenocarcinoma cells [Citation84]. These studies, however, not only utilized cells from different species, but different PIKFYVE inhibitors and different methods of analyses. For example, analysis of lysosomal pH in HeLa cells using both a ratiometric method and LysoSensor concluded that vacuolin-1 reduced lysosomal acidity from pH 4.8 to pH 5.3 [Citation84].
In the present study, a ratiometric analysis of lysosomes preloaded with Oregon Green Dextran revealed that inhibition of PIKFYVE with either WX8 or apilimod did not result in deacidification of lysosomes either in mouse RAW264.7 cells ()), or in human U2OS osteosarcoma cells ()). These results were consistent with the fact that LysoTracker stained all of the lysosomes in WX8-treated U2OS cells, although to varying extents ()), as well as in RAW cells [Citation31]. Therefore, in U2OS and RAW cells, inhibition of PIKFYVE, as confirmed by the rapid accumulation of enlarged lysosomes, did not cause lysosome deacidification. However, it did impair trafficking of molecules into lysosomes.
The ratiometric method revealed a mean pH of 5.3 for lysosomes in untreated U2OS cells and 5.0 for lysosomes in WX8 treated U2OS cells (). Therefore, LysoSensor, with a pKa of 5.2, should have stained lysosomes under both conditions. Similarly, BODIPY-pepstatin-A, which binds specifically to mature CTSD at acidic pH, should also have stained both untreated and treated cells. The fact that both of these agents stained lysosomes only in untreated cells strongly suggests that their ability to enter the enlarged lysosomes of treated cells was impaired.
This conclusion was confirmed in 2 ways. First, acridine orange stained lysosomes in untreated U2OS cells, and it stained a few of the smaller lysosomes in WX8 treated cells. However, most of the enlarged lysosomes in WX8-treated cells were neither red nor green; they were empty of dye ()). In contrast, analysis of autophagy using the red:green fluorescence intensity ratio from acridine orange measured with accuracy the increase in autophagy induced by starvation or rapamycin, and the reduction in autophagy produced by BafA1 or depletion of Beclin1 (BECN1) or ATG7 without encountering ‘black holes’ [Citation35]. Second, the fraction of mature CTSD was reduced in U20S cells treated with WX8 ()). Taken together, these results reveal that lysosomes in both U2OS and RAW cells treated with PIKFYVE inhibitors are dysfunctional, not because they are no longer acidic, but because traffic into these vesicles is impaired. Given the facts that the efficacy of the WX8-family of PIKFYVE inhibitors varied at least 400-fold ()), that their efficacy was time and concentration dependent and reversible, and that the sensitivity of different human cell lines to WX8 varied as much as 1000-fold ()), it would be surprising if the effects of PIKFYVE inhibition were not sensitive to experimental conditions.
Although the mechanism by which PIKFYVE inhibitors impair the ability of molecules to enter lysosomes is unknown, it does not involve inhibiting V-ATPase, because BafA1 (a specific inhibitor of V-ATPase activity) prevents lysosome acidification (Figure S11) without inducing lysosomal enlargement. In yeast, vacuole fusion requires the physical presence of V-ATPase, but not its pump activity, whereas fission requires V-ATPase activity [Citation88]. If the same were true in mammals, BafA1 would have been expected to cause lysosomal enlargement. The fact that BafA1 prevented the WX8-family from inducing lysosomal enlargement () confirmed that V-ATPase is required for lysosomal enlargement when PIKFYVE activity is suppressed [Citation47] and suggests that V-ATPase is required for homotypic lysosome fusion. In Drosophila, however, lysosomal acidification is not a prerequisite for heterotypic lysosome fusion, because V-ATPase-deficient lysosomes can fuse with autophagosomes and endosomes [Citation44]. BafA1 prevents autophagosome-lysosome fusion in Drosophila cells by depletion of the calcium ion pump, a secondary target of BafA1. Whether or not the same is true for homotypic lysosome fusion remains to be investigated.
Therapeutic potential against autophagy-dependent cancer cells
Activation and inhibition of autophagy are of significant interest as potential therapeutic approaches to infection, inflammation, immunity, and neurodegenerative diseases [Citation89,Citation90]. However, the role of autophagy in cancer remains paradoxical. In non-malignant cells, autophagy promotes genomic stability by maintaining homeostasis, thereby suppressing cancer, but in malignant cells, autophagy promotes cancer by allowing them to proliferate and migrate under conditions where normal cells become quiescent [reviewed in [Citation91–Citation94]]. Therefore, systemic disruption of autophagy by experimental gene ablation or naturally occurring gene mutations can lead to cancer [Citation95–Citation98], whereas pharmacological inhibition of autophagy in humans with either hydroxychloroquine or chloroquine, alone or in combination with chemotherapy, causes tumor shrinkage [reviewed in [Citation6,Citation99]].
Here we provide proof-of-principle for the therapeutic potential of the WX8-family of PIKFYVE inhibitors in the treatment of autophagy-dependent human cancers. The ability of the WX8-family of PIKFYVE inhibitors to effectively disrupt multiple events in lysosome homeostasis presumably accounts for the fact that WX8 was at least 100X more effective at killing autophagy-addicted melanoma cells than the lysosome deacidifiers HCQ and CQ, 2 drugs currently used in clinical trials to disrupt autophagy by inhibiting the ability of lysosomes to degrade proteins [Citation6,Citation59,Citation99–Citation101]. WX8 was still 20X more effective than HCQ and CQ at arresting the growth and proliferation of cancer cells that depend on autophagy, but that are not addicted. Non-malignant human cells were unaffected by WX8 at concentrations 1000X greater than required to kill autophagy-addicted cells. These results suggest that the multiple disruptions in lysosome homeostasis induced by WX8 provide enhanced therapeutic potential over drugs that disrupt autophagy at a single event. The fact that concentrations of WX8 that could arrest or kill autophagy-dependent cancer cells had little, if any, effect on cell proliferation or viability of nonmalignant cells, as well as fact that apilimod can reduce the growth of mouse xenografts of B-cell non-Hodgkin lymphoma [Citation63], strongly support further investigation into the application of PIKFYVE inhibitors in cancer chemotherapy.
Materials and methods
Reagents
WX8, NDF, WWL, XB6 and XBA (Specs, ChemDiv, Life Chemicals), rapamycin (Selleck Chemicals, S1039), apilimod (Santa Cruz Biotechnology, sc-480051), vacuolin (Cayman Chemicals, 20425) and YM201636 (Cayman Chemicals, 13576) were dissolved in dimethyl sulfoxide (DMSO) at 20 mM concentrations and stored at −20°C. WX8-family compounds were assayed for purity by high-pressure liquid chromatography and their identity confirmed by mass spectroscopy. Solutions were stable for at least 2 y. Bafilomycin A1 (B1793), chloroquine (C6628), and hydroxychloroquine (H0915) were from Sigma-Aldrich Chemicals. Cell-Titer Glo kit (G7570) used to assess cell viability was from Promega Biotechnology. N-ethylmaleimide (E3876) was from Sigma-Aldrich. ONTARGET SMART-pools and non-targeting control siRNAs against human VPS39 and VPS41 were from GE Healthcare Dharmacon. PIKFYVE siRNA (Sc-39142), PIP4K2C siRNA (Sc-95832) and transfection reagent (Sc-29528) were from Santa Cruz Biotechnology. Premo Autophagy Sensors GFP-LC3 (BacMam 2.0), Tandem Sensor RFP-GFP-LC3B (P36239), RFP-SQSTM1/p62 (P36241, LAMP1-RFP (C10504), LysoTracker Green DND-26 (L7526), LysoSensor Green DND-189 (L7535), BODIPY FL-pepstatin A (P12271), and acridine orange (A3568) were from ThermoFisher Scientific.
Cells culture
Cells were obtained from the American Type Culture Collection [U2OS (HTB96), A375 (CRL-1619), 293T (CRL-3216), HFF-1 (SCRC-1041)]. BORCS5-KO HeLa cells have been described previously [Citation19]. Osteosarcoma U2OS were routinely seeded in 6-well plates (0.5 x 105 cells/well) and cultured in Dulbecco’s Modified Eagle’s medium supplemented with L-glutamine, 4.5 g/L glucose, sodium pyruvate, phenol red, and either heat-inactivated or normal 10% fetal calf serum at 37°C in 5% CO2. Approximately 15–19 h later, the indicated compound was added in 1:1000 dilutions. Results were the same using either heat-inactivated or normal fetal calf serum. Melanoma A375, embryonic kidney 293T and human foreskin fibroblast HFF cells were cultured in the same medium, except that cells were seeded into 12-well plates at 103 cells/well.
Microscopy
Phase contrast photographs were taken on an Olympus CKX41 microscope. Confocal photographs were taken on a Leica TCS-SP5II. For immunofluorescence microscopy, the cells were fixed in 4% paraformaldehyde (Electron Microscopy Sciences, 15714) in PBS for 12 min, permeabilized with 0.2% Triton X-100 (American BIO, AB2025-00100) in PBS for 10 min, and blocked in 0.2% BSA (Sigma-Aldrich, A7030) in PBS for 30 min at 37°C. For endogenous LC3 staining, cells were permeabilized in methanol for 20 min at −20°C after the paraformaldehyde fixation. The cells were sequentially incubated with primary antibodies against LAMP1 (Developmental Studies Hybridoma Bank), LC3 and LAMTOR4 (Cell Signaling Technology, 12741S, 13140S), Monoclonal FLAG-epitope M2 antibody (Sigma-Aldrich, F3165) and then secondary antibodies conjugated to either Donkey Anti-mouse Alexa Fluor 488 or 555 (Life Technologies, A21202; A31570) diluted with 0.2% bovine serum albumin (mpbio, 160069) in phosphate buffered saline (PBS) for 30 min at 37°C. Coverslips were mounted on glass slides using Fluoromount-G (Electron Microscopy Sciences, 17984–24). Cells were imaged using a Zeiss LSM880 with AiryScan confocal microscope (Carl Zeiss AG, Oberkochen, Germany) or a Leica TCS-SP5II. The final composite images were created and properly analyzed for lysosomal size examination using ImageJ (NIH).
Ratiometric analysis of lysosomal pH
To determine the pH of individual lysosomes, dual-wavelength ratio imaging was conducted as previously described [Citation102], with minor modifications. U2OS and RAW264.7 cells were cultured in imaging chambers (Cellvis, C4-1.5/h-N) in complete culture medium at 37°C and 5% CO2. Cells were incubated overnight with 50 µg/mL Oregon Green 488 dextran (Invitrogen, D7170). The following day, the cells were cultured with dextran-free complete culture medium for at least 2 h to ensure lysosomal targeting of the probe. Cells were then cultured with WX8 or apilimod for the indicated times and concentrations. These results were compared with cells cultured with DMSO at the same volume used to deliver WX8 and apilimod (vehicle), and with cells cultured with ammonium chloride to increase the pH of lysosomes [Citation103] (positive control). Confocal micrographs were immediately acquired for each condition, followed by a pH calibration using buffers that span pH 4 to pH 7. pH calibration buffers were prepared fresh, as previously described [Citation102], filter sterilized, and then warmed to 37°C. The 488:440 nm ratios of 30–50 lysosomes per condition for each of 3 independent experiments were used to calculate lysosomal pH values from the calibration curve.
Western immuno-blotting analysis
Total cell lysates were prepared (in 4X dye). Proteins were fractionated at 200V in a NuPAGE 4–12% bis-tris polyacrylamide gel (Novex, NP0323) using NuPAGE MES running buffer (Novex, NP0002) and then stained with PageBlue (Thermo Fisher Scientific, 24260) in order to quantify the relative amounts of histones in each sample. These data were then used to normalize loading of samples for western immuno-blotting analysis. Proteins were wet-transferred onto nitrocellulose membranes (Bio-Rad, 162–0112) using NuPAGE transfer buffer (Novex, NP-0006–1) for 1 h at 30 volts. Membranes were then stained with dilute Ponceau S solution, blocked in 5% milk for 30 min at room temperature, and incubated overnight at 4°C in primary antibody. Membranes were washed with phosphate-buffered saline with TWEEN 20 (Sigma, P9416) (PBST) 3 times for 10 min each, incubated with secondary antibodies in 5% milk for 1 h, washed 3 times with PBST again, covered in SuperSignal West Pico chemiluminescent substrate (Thermo Fisher Scientific, 34080) and exposed to film (Denville Scientific, HyBlot ES, E3218).
Antibodies from Cell Signaling Technology were diluted as follows: SQSTM1/p62 (8025) 1:5,000, LC3 I/II (12741) 1:5,000, p-RPS6 S240/244 (5364) 1:20,000, RPS6 (2217) 1:20,000, and CTSD (2284S) 1:1,000, and HRP-conjugated secondary anti-rabbit (7074) 1:10,000. Antibodies from Sigma-Aldrich were PIP4K2C (SAB1407977) 1:10,000, ACTB/β-actin (A5441) 1:20,000, and anti-mouse (A4416) 1:10,000. Antibodies from Santa Cruz Biotechnology were VPS39 (sc-514762,) 1:1,000, VPS41 (sc-377118) 1:1,000, and GAPDH (sc-20357) 1:1,000. Other antibodies were PIKFYVE (Millipore, MABS522), and LAMP1 (Developmental studies hybridoma bank, H4A3) 1:5,000.
Acknowlegements
We thank Louis Dye and Michal Jarnik (NICHD Microscopy and Imaging Core) for electron microscopy, and Paul Shinn (NCATS) for assaying the purity of WX8 compounds and confirming their identity by mass spectroscopy
Supplemental Material
Download Zip (68.9 MB)Disclosure statement
No potential conflict of interest was reported by the authors.
Supplemental material
Supplemental data for this article can be accessed here.
Additional information
Funding
References
- Mony VK, Benjamin S, O’Rourke EJ. A lysosome-centered view of nutrient homeostasis. Autophagy. 2016;12(4):619–631. PubMed PMID: 27050453; PubMed Central PMCID: PMC4836021
- Galluzzi L, Bravo-San Pedro JM, Levine B, et al. Pharmacological modulation of autophagy: therapeutic potential and persisting obstacles. Nat Rev Drug Discov. 2017 Jul;16(7):487–511. PubMed PMID: 28529316; PubMed Central PMCID: PMC5713640.
- Yu L, Chen Y, Tooze SA, Autophagy pathway: cellular and molecular mechanisms. Autophagy. 2017 Sep 21:1–9. DOI:10.1080/15548627.2017.1378838 PubMed PMID: 28933638.
- Hanahan D, Weinberg RA. Hallmarks of cancer: the next generation. Cell. 2011 Mar 4;144(5):646–674. PubMed PMID: 21376230.
- Gotink KJ, Broxterman HJ, Labots M, et al. Lysosomal sequestration of sunitinib: a novel mechanism of drug resistance. Clin Cancer Res. 2011 Dec 1;17(23):7337–7346. PubMed PMID: 21980135; PubMed Central PMCID: PMC4461037.
- Levy JMM, Towers CG, Thorburn A. Targeting autophagy in cancer. Nat Rev Cancer. 2017 Sep;17(9):528–542. PubMed PMID: 28751651.
- Mulcahy Levy JM, Zahedi S, Griesinger AM, et al. Autophagy inhibition overcomes multiple mechanisms of resistance to BRAF inhibition in brain tumors. Elife. 2017 Jan 17;6. PubMed PMID: 28094001; PubMed Central PMCID: PMC5241115.
- Cufi S, Vazquez-Martin A, Oliveras-Ferraros C, et al. The anti-malarial chloroquine overcomes primary resistance and restores sensitivity to trastuzumab in HER2-positive breast cancer. Sci Rep. 2013;3:2469. PubMed PMID: 23965851; PubMed Central PMCID: PMC3749547.
- Foley M, Tilley L. Quinoline antimalarials: mechanisms of action and resistance and prospects for new agents. Pharmacol Ther. 1998 Jul;79(1):55–87. PubMed PMID: 9719345.
- Solomon VR, Lee H. Chloroquine and its analogs: a new promise of an old drug for effective and safe cancer therapies. Eur J Pharmacol. 2009 Dec 25;625(1–3):220–233. PubMed PMID: 19836374.
- Goardon N, Marchi E, Atzberger A, et al. Coexistence of LMPP-like and GMP-like leukemia stem cells in acute myeloid leukemia. Cancer Cell. 2011 Jan 18;19(1):138–152. PubMed PMID: 21251617.
- Yang S, Wang X, Contino G, et al. Pancreatic cancers require autophagy for tumor growth. Genes Dev. 2011 Apr 01;25(7):717–729. PubMed PMID: 21406549; PubMed Central PMCID: PMC3070934.
- Pellegrini P, Strambi A, Zipoli C, et al. Acidic extracellular pH neutralizes the autophagy-inhibiting activity of chloroquine: implications for cancer therapies. Autophagy. 2014 Apr;10(4):562–571. PubMed PMID: 24492472; PubMed Central PMCID: PMC3984580.
- Marino ML, Pellegrini P, Di Lernia G, et al. Autophagy is a protective mechanism for human melanoma cells under acidic stress. J Biol Chem. 2012 Aug 31;287(36):30664–30676. PubMed PMID: 22761435; PubMed Central PMCID: PMC3436311.
- Piao S, Amaravadi RK. Targeting the lysosome in cancer. Ann N Y Acad Sci. 2016 May;1371(1):45–54. PubMed PMID: 26599426; PubMed Central PMCID: PMC4879098
- Davidson SM, Vander Heiden MG. Critical functions of the lysosome in cancer biology. Annu Rev Pharmacol Toxicol. 2017 Jan 6;57:481–507. PubMed PMID: 27732799.
- Rebecca VW, Nicastri MC, McLaughlin N, et al. A unified approach to targeting the lysosome’s degradative and growth signaling roles. Cancer Discov. 2017 Nov;7(11):1266–1283. PubMed PMID: 28899863.
- Lee CY, Johnson RL, Wichterman-Kouznetsova J, et al. High-throughput screening for genes that prevent excess DNA replication in human cells and for molecules that inhibit them. Methods. 2012 Jun;57(2):234–248. PubMed PMID: 22503772; PubMed Central PMCID: PMC4149752.
- Zhu W, Lee CY, Johnson RL, et al. An image-based, high-throughput screening assay for molecules that induce excess DNA replication in human cancer cells. Mol Cancer Res. 2011 Mar;93:294–310. [pii]. PubMed PMID: 21257818; PubMed Central PMCID: PMC3060295. eng.
- Chernomordik LV, Kozlov MM. Protein-lipid interplay in fusion and fission of biological membranes. Annu Rev Biochem. 2003;72:175–207. PubMed PMID: 14527322.
- Jia R, Guardia CM, Pu J, et al. BORC coordinates encounter and fusion of lysosomes with autophagosomes. Autophagy. 2017 Oct 3;13(10):1648–1663. PubMed PMID: 28825857; PubMed Central PMCID: PMC5640200.
- Pu J, Schindler C, Jia R, et al. BORC, a multisubunit complex that regulates lysosome positioning. Dev Cell. 2015 Apr 20;33(2):176–188. PubMed PMID: 25898167; PubMed Central PMCID: PMC4788105.
- Niwa S, Tao L, Lu SY, et al. BORC regulates the axonal transport of synaptic vesicle precursors by activating ARL-8. Curr Biol. 2017 Sep 11;27(17):2569–2578 e4. PubMed PMID: 28823680; PubMed Central PMCID: PMC5693321.
- Khatter D, Raina VB, Dwivedi D, et al. The small GTPase Arl8b regulates assembly of the mammalian HOPS complex on lysosomes. J Cell Sci. 2015 May 1;128(9):1746–1761. PubMed PMID: 25908847; PubMed Central PMCID: PMC4432227.
- Pols MS, ten Brink C, Gosavi P, et al. The HOPS proteins hVps41 and hVps39 are required for homotypic and heterotypic late endosome fusion. Traffic. 2013 Feb;14(2):219–232. PubMed PMID: 23167963.
- Orr A, Wickner W, Rusin SF, et al. Yeast vacuolar HOPS, regulated by its kinase, exploits affinities for acidic lipids and Rab: gTPfor membrane binding and to catalyze tethering and fusion. Mol Biol Cell. 2015 Jan 15;26(2):305–315. PubMed PMID: 25411340; PubMed Central PMCID: PMC4294677.
- Martens S, McMahon HT. Mechanisms of membrane fusion: disparate players and common principles. Nat Rev Mol Cell Biol. 2008 Jul;9(7):543–556. PubMed PMID: 18496517.
- Beckers CJ, Block MR, Glick BS, et al. Vesicular transport between the endoplasmic reticulum and the Golgi stack requires the NEM-sensitive fusion protein. Nature. 1989 Jun 1;339(6223):397–398. PubMed PMID: 2542798.
- Wang T, Li L, Hong W. SNARE proteins in membrane trafficking. Traffic. 2017 Dec;18(12):767–775. PubMed PMID: 28857378.
- Mindell JA. Lysosomal acidification mechanisms. Annu Rev Physiol. 2012;74:69–86. PubMed PMID: 22335796.
- Ho CY, Choy CH, Wattson CA, et al. The Fab1/PIKfyve phosphoinositide phosphate kinase is not necessary to maintain the pH of lysosomes and of the yeast vacuole. J Biol Chem. 2015 Apr 10;290(15):9919–9928. PubMed PMID: 25713145; PubMed Central PMCID: PMC4392288.
- Yapici NB, Bi Y, Li P, et al. Highly stable and sensitive fluorescent probes (LysoProbes) for lysosomal labeling and tracking. Sci Rep. 2015 Feb;26(5):8576. PubMed PMID: 25715948; PubMed Central PMCID: PMC4341211.
- Maxson ME, Grinstein S. The vacuolar-type H(+)-ATPase at a glance - more than a proton pump. J Cell Sci. 2014 Dec 1;127(Pt 23):4987–4993. PubMed PMID: 25453113.
- Chen CS, Chen WN, Zhou M, et al. Probing the cathepsin D using a BODIPY FL-pepstatin A: applications in fluorescence polarization and microscopy. J Biochem Biophys Methods. 2000 Mar 16;42(3):137–151. PubMed PMID: 10737220.
- Thome MP, Filippi-Chiela EC, Villodre ES, et al. Ratiometric analysis of Acridine Orange staining in the study of acidic organelles and autophagy. J Cell Sci. 2016 Dec 15;129(24):4622–4632. PubMed PMID: 27875278.
- Laurent-Matha V, Derocq D, Prebois C, et al. Processing of human cathepsin D is independent of its catalytic function and auto-activation: involvement of cathepsins L and B. J Biochem. 2006 Mar;139(3):363–371. PubMed PMID: 16567401; PubMed Central PMCID: PMC2376303.
- Mizushima N, Yamamoto A, Hatano M, et al. Dissection of autophagosome formation using Apg5-deficient mouse embryonic stem cells. J Cell Biol. 2001 Feb 19;152(4):657–668. PubMed PMID: 11266458; PubMed Central PMCID: PMC2195787.
- Pfeifer U. Inhibition by insulin of the formation of autophagic vacuoles in rat liver. A morphometric approach to the kinetics of intracellular degradation by autophagy. J Cell Biol. 1978 Jul;78(1):152–167. PubMed PMID: 670291; PubMed Central PMCID: PMC2110173.
- Schworer CM, Shiffer KA, Mortimore GE. Quantitative relationship between autophagy and proteolysis during graded amino acid deprivation in perfused rat liver. J Biol Chem. 1981 Jul 25;256(14):7652–7658. PubMed PMID: 7019210.
- Li Z, Ji X, Wang D, et al. Autophagic flux is highly active in early mitosis and differentially regulated throughout the cell cycle. Oncotarget. 2016 Jun 28;7(26):39705–39718. PubMed PMID: 27213594; PubMed Central PMCID: PMC5129964.
- Kimura S, Noda T, Yoshimori T. Dissection of the autophagosome maturation process by a novel reporter protein, tandem fluorescent-tagged LC3. Autophagy. 2007 Sep–oct;3(5):452–460. PubMed PMID: 17534139.
- Ravikumar B, Duden R, Rubinsztein DC. Aggregate-prone proteins with polyglutamine and polyalanine expansions are degraded by autophagy. Hum Mol Genet. 2002 May 01;11(9):1107–1117. PubMed PMID: 11978769.
- Sarkar S, Ravikumar B, Floto RA, et al. Rapamycin and mTOR-independent autophagy inducers ameliorate toxicity of polyglutamine-expanded huntingtin and related proteinopathies. Cell Death Differ. 2009 Jan;16(1):46–56. PubMed PMID: 18636076.
- Mauvezin C, Nagy P, Juhasz G, et al. Autophagosome-lysosome fusion is independent of V-ATPase-mediated acidification. Nat Commun. 2015 May;11(6):7007. PubMed PMID: 25959678; PubMed Central PMCID: PMC4428688.
- Mauvezin C, Neufeld TP. Bafilomycin A1 disrupts autophagic flux by inhibiting both V-ATPase-dependent acidification and Ca-P60A/SERCA-dependent autophagosome-lysosome fusion. Autophagy. 2015;11(8):1437–1438. PubMed PMID: 26156798; PubMed Central PMCID: PMC4590655.
- Luzio JP, Pryor PR, Bright NA. Lysosomes: fusion and function. Nat Rev Mol Cell Biol. 2007 Aug;8(8):622–632. PubMed PMID: 17637737.
- Compton LM, Ikonomov OC, Sbrissa D, et al. Active vacuolar H+ ATPase and functional cycle of Rab5 are required for the vacuolation defect triggered by PtdIns(3,5)P2 loss under PIKfyve or Vps34 deficiency. Am J Physiol Cell Physiol. 2016 Sep 01;311(3):C366–C77. PubMed PMID: 27335171; PubMed Central PMCID: PMC5129759.
- Davis MI, Hunt JP, Herrgard S, et al. Comprehensive analysis of kinase inhibitor selectivity. Nat Biotechnol. 2011 Nov;29(11):1046–1051. PubMed PMID: 22037378.
- Ikonomov OC, Sbrissa D, Delvecchio K, et al. The phosphoinositide kinase PIKfyve is vital in early embryonic development: preimplantation lethality of PIKfyve-/- embryos but normality of PIKfyve± mice. J Biol Chem. 2011 Apr 15;286(15):13404–13413. PubMed PMID: 21349843; PubMed Central PMCID: PMC3075686.
- Ikonomov OC, Sbrissa D, Shisheva A. Localized PtdIns 3,5-P2 synthesis to regulate early endosome dynamics and fusion. Am J Physiol Cell Physiol. 2006 Aug;291(2):C393–C404. PubMed PMID: 16510848.
- Sbrissa D, Ikonomov OC, Fu Z, et al. Core protein machinery for mammalian phosphatidylinositol 3,5-bisphosphate synthesis and turnover that regulates the progression of endosomal transport. Novel Sac phosphatase joins the ArPIKfyve-PIKfyve complex. J Biol Chem. 2007 Aug 17;282(33):23878–23891. PubMed PMID: 17556371.
- Shim H, Wu C, Ramsamooj S, et al. Deletion of the gene Pip4k2c, a novel phosphatidylinositol kinase, results in hyperactivation of the immune system. Proc Natl Acad Sci U S A. 2016 Jul 05;113(27):7596–7601. PubMed PMID: 27313209; PubMed Central PMCID: PMC4941458.
- Mackey AM, Sarkes DA, Bettencourt I, et al. PIP4kgamma is a substrate for mTORC1 that maintains basal mTORC1 signaling during starvation. Sci Signal. 2014 Nov 4;7(350):ra104. PubMed PMID: 25372051; PubMed Central PMCID: PMC4579097.
- Al-Ramahi I, Giridharan SSP, Chen YC, et al. Inhibition of PIP4Kgamma ameliorates the pathological effects of mutant huntingtin protein. Elife. 2017 Dec 26:6. DOI:10.7554/eLife.29123 PubMed PMID: 29256861; PubMed Central PMCID: PMC5743427.
- Laplante M, Sabatini DM. mTOR signaling at a glance. J Cell Sci. 2009 Oct 15;122(Pt 20):3589–3594. PubMed PMID: 19812304; PubMed Central PMCID: PMC2758797.
- Huang S, Houghton PJ. Inhibitors of mammalian target of rapamycin as novel antitumor agents: from bench to clinic. Curr Opin Invest Drugs. 2002 Feb;3(2):295–304. PubMed PMID: 12020063.
- Magnuson B, Ekim B, Fingar DC. Regulation and function of ribosomal protein S6 kinase (S6K) within mTOR signalling networks. Biochem J. 2012 Jan 1;441(1):1–21. PubMed PMID: 22168436.
- Strohecker AM, White E. Targeting mitochondrial metabolism by inhibiting autophagy in BRAF-driven cancers. Cancer Discov. 2014 Jul;4(7):766–772. PubMed PMID: 24860158; PubMed Central PMCID: PMC4090279.
- Goodall ML, Wang T, Martin KR, et al. Development of potent autophagy inhibitors that sensitize oncogenic BRAF V600E mutant melanoma tumor cells to vemurafenib. Autophagy. 2014 Jun;10(6):1120–1136. PubMed PMID: 24879157; PubMed Central PMCID: PMC4091172.
- Akan P, Alexeyenko A, Costea PI, et al. Comprehensive analysis of the genome transcriptome and proteome landscapes of three tumor cell lines. Genome Med. 2012;4(11):86. PubMed PMID: 23158748; PubMed Central PMCID: PMC3580420.
- Ikonomov OC, Sbrissa D, Shisheva A. Mammalian cell morphology and endocytic membrane homeostasis require enzymatically active phosphoinositide 5-kinase PIKfyve. J Biol Chem. 2001 Jul 13;276(28):26141–26147. PubMed PMID: 11285266.
- de Lartigue J, Polson H, Feldman M, et al. PIKfyve regulation of endosome-linked pathways. Traffic. 2009 Jul;10(7):883–893. PubMed PMID: 19582903; PubMed Central PMCID: PMC2723830.
- Gayle S, Landrette S, Beeharry N, et al. Identification of apilimod as a first-in-class PIKfyve kinase inhibitor for treatment of B-cell non-Hodgkin lymphoma. Blood. 2017 Mar 30;129(13):1768–1778. PubMed PMID: 28104689.
- Sano O, Kazetani K, Funata M, et al. Vacuolin-1 inhibits autophagy by impairing lysosomal maturation via PIKfyve inhibition. FEBS Lett. 2016 Jun;590(11):1576–1585. PubMed PMID: 27135648.
- Jefferies HB, Cooke FT, Jat P, et al. A selective PIKfyve inhibitor blocks PtdIns(3,5)P(2) production and disrupts endomembrane transport and retroviral budding. EMBO Rep. 2008 Feb;9(2):164–170. PubMed PMID: 18188180; PubMed Central PMCID: PMC2246419.
- Cai X, Xu Y, Cheung AK, et al. PIKfyve, a class III PI kinase, is the target of the small molecular IL-12/IL-23 inhibitor apilimod and a player in Toll-like receptor signaling. Chem Biol. 2013 Jul 25;20(7):912–921. PubMed PMID: 23890009; PubMed Central PMCID: PMC4878021.
- Dove SK, Dong K, Kobayashi T, et al. Phosphatidylinositol 3,5-bisphosphate and Fab1p/PIKfyve underPPIn endo-lysosome function. Biochem J. 2009 Apr 1;419(1):1–13. PubMed PMID: 19272020.
- Nicot AS, Laporte J. Endosomal phosphoinositides and human diseases. Traffic. 2008 Aug;9(8):1240–1249. PubMed PMID: 18429927; PubMed Central PMCID: PMC2607523.
- Shisheva A. PIKfyve: partners, significance, debates and paradoxes. Cell Biol Int. 2008 Jun;32(6):591–604. PubMed PMID: 18304842; PubMed Central PMCID: PMC2491398.
- Hessvik NP, Overbye A, Brech A, et al. PIKfyve inhibition increases exosome release and induces secretory autophagy. Cell Mol Life Sci. 2016 Dec;73(24):4717–4737. PubMed PMID: 27438886.
- Demirsoy S, Martin S, Motamedi S, et al. ATP13A2/PARK9 regulates endo-/lysosomal cargo sorting and proteostasis through a novel PI(3, 5)P2-mediated scaffolding function. Hum Mol Genet. 2017 May 1;26(9):1656–1669. PubMed PMID: 28334751.
- Ho CY, Alghamdi TA, Botelho RJ. Phosphatidylinositol-3,5-bisphosphate: no longer the poor PIP2. Traffic. 2012 Jan;13(1):1–8. PubMed PMID: 21736686.
- Jin N, Lang MJ, Weisman LS. Phosphatidylinositol 3,5-bisphosphate: regulation of cellular events in space and time. Biochem Soc Trans. 2016 Feb;44(1):177–184. PubMed PMID: 26862203; PubMed Central PMCID: PMC4836390
- Vicinanza M, Korolchuk VI, Ashkenazi A, et al. PI(5)P regulates autophagosome biogenesis. Mol Cell. 2015 Jan 22;57(2):219–234. PubMed PMID: 25578879; PubMed Central PMCID: PMC4306530.
- Hasegawa J, Iwamoto R, Otomo T, et al. Autophagosome-lysosome fusion in neurons requires INPP5E, a protein associated with Joubert syndrome. Embo J. 2016 Sep 1;35(17):1853–1867. PubMed PMID: 27340123; PubMed Central PMCID: PMC5007553.
- Hasegawa J, Strunk BS, Weisman LS. PI5P and PI(3,5)P2: minor, but essential phosphoinositides. Cell Struct Funct. 2017 May 3;42(1):49–60. PubMed PMID: 28302928.
- Yu L, McPhee CK, Zheng L, et al. Termination of autophagy and reformation of lysosomes regulated by mTOR. Nature. 2010 Jun 17;465(7300):942–946. PubMed PMID: 20526321; PubMed Central PMCID: PMC2920749.
- Li X, Rydzewski N, Hider A, et al. A molecular mechanism to regulate lysosome motility for lysosome positioning and tubulation. Nat Cell Biol. 2016 Apr;18(4):404–417. PubMed PMID: 26950892; PubMed Central PMCID: PMC4871318.
- Wartosch L, Gunesdogan U, Graham SC, et al. Recruitment of VPS33A to HOPS by VPS16 is required for lysosome fusion with endosomes and autophagosomes. Traffic. 2015 Jul;16(7):727–742. PubMed PMID: 25783203; PubMed Central PMCID: PMC4510706.
- Vivona S, Cipriano DJ, O’Leary S, et al. Disassembly of all SNARE complexes by N-ethylmaleimide-sensitive factor (NSF) is initiated by a conserved 1:1 interaction between alpha-soluble NSF attachment protein (SNAP)and SNARE complex. J Biol Chem. 2013 Aug 23;288(34):24984–24991. PubMed PMID: 23836889; PubMed Central PMCID: PMC3750193.
- Pu J, Guardia CM, Keren-Kaplan T, et al. Mechanisms and functions of lysosome positioning. J Cell Sci. 2016 Dec 1;129(23):4329–4339. PubMed PMID: 27799357; PubMed Central PMCID: PMC5201012.
- Bissig C, Hurbain I, Raposo G, et al. PIKfyve activity regulates reformation of terminal storage lysosomes from endolysosomes. Traffic. 2017 Nov;18(11):747–757. PubMed PMID: 28857423.
- Choy CH, Saffi G, Gray MA, et al. Lysosome enlargement during inhibition of the lipid kinase PIKfyve proceeds through lysosome coalescence. J Cell Sci. 2018 May 21;131(10). DOI:10.1242/jcs.213587 PubMed PMID: 29661845.
- Lu Y, Dong S, Hao B, et al. Vacuolin-1 potently and reversibly inhibits autophagosome-lysosome fusion by activating RAB5A. Autophagy. 2014;10(11):1895–1905. PubMed PMID: 25483964; PubMed Central PMCID: PMC4502727.
- Martin S, Harper CB, May LM, et al. Inhibition of PIKfyve by YM-201636 dysregulates autophagy and leads to apoptosis-independent neuronal cell death. PLoS One. 2013;8(3):e60152. PubMed PMID: 23544129; PubMed Central PMCID: PMC3609765.
- Kim GH, Dayam RM, Prashar A, et al. PIKfyve inhibition interferes with phagosome and endosome maturation in macrophages. Traffic. 2014 Oct;15(10):1143–1163. PubMed PMID: 25041080.
- Tsuruta F, Dolmetsch RE. PIKfyve mediates the motility of late endosomes and lysosomes in neuronal dendrites. Neurosci Lett. 2015 Sep 25;605:18–23. PubMed PMID: 26232680.
- Baars TL, Petri S, Peters C, et al. Role of the V-ATPase in regulation of the vacuolar fission-fusion equilibrium. Mol Biol Cell. 2007 Oct;18(10):3873–3882. PubMed PMID: 17652457; PubMed Central PMCID: PMC1995711.
- Walter C, Clemens LE, Muller AJ, et al. Activation of AMPK-induced autophagy ameliorates Huntington disease pathology in vitro. Neuropharmacology. 2016 Sep;108:24–38. PubMed PMID: 27133377.
- Deretic V, Saitoh T, Akira S. Autophagy in infection, inflammation and immunity. Nat Rev Immunol. 2013 Oct;13(10):722–737. PubMed PMID: 24064518; PubMed Central PMCID: PMC5340150.
- Kimmelman AC, White E. Autophagy and tumor metabolism. Cell Metab. 2017 May 2;25(5):1037–1043. PubMed PMID: 28467923; PubMed Central PMCID: PMC5604466.
- Nyfeler B, Eng CH. Revisiting autophagy addiction of tumor cells. Autophagy. 2016 Jul 2;12(7):1206–1207. PubMed PMID: 27097231; PubMed Central PMCID: PMC4990994.
- Mainz L, Rosenfeldt MT. Autophagy and cancer - insights from mouse models. FEBS J. 2017 Sep 16. DOI:10.1111/febs.14274 PubMed PMID: 28921866.
- Mowers EE, Sharifi MN, Macleod KF. Autophagy in cancer metastasis. Oncogene. 2017 Mar 23;36(12):1619–1630. PubMed PMID: 27593926; PubMed Central PMCID: PMC5337449.
- Mathew R, Karp CM, Beaudoin B, et al. Autophagy suppresses tumorigenesis through elimination of p62. Cell. 2009 Jun 12;137(6):1062–1075. PubMed PMID: 19524509; PubMed Central PMCID: PMC2802318.
- Degenhardt K, Mathew R, Beaudoin B, et al. Autophagy promotes tumor cell survival and restricts necrosis, inflammation, and tumorigenesis. Cancer Cell. 2006 Jul;10(1):51–64. PubMed PMID: 16843265; PubMed Central PMCID: PMC2857533.
- Liu XD, Yao J, Tripathi DN, et al. Autophagy mediates HIF2alpha degradation and suppresses renal tumorigenesis. Oncogene. 2015 May 7;34(19):2450–2460. PubMed PMID: 24998849; PubMed Central PMCID: PMC4286517.
- Karantza-Wadsworth V, Patel S, Kravchuk O, et al. Autophagy mitigates metabolic stress and genome damage in mammary tumorigenesis. Genes Dev. 2007 Jul 1;21(13):1621–1635. PubMed PMID: 17606641; PubMed Central PMCID: PMC1899472.
- Chude CI, Amaravadi RK. Targeting autophagy in cancer: update on clinical trials and novel inhibitors. Int J Mol Sci. 2017 June 16;18(6). DOI:10.3390/ijms18061279 PubMed PMID: 28621712; PubMed Central PMCID:PMC5486101.
- Rangwala R, Chang YC, Hu J, et al. Combined MTOR and autophagy inhibition: phase I trial of hydroxychloroquine and temsirolimus in patients with advanced solid tumors and melanoma. Autophagy. 2014 Aug;10(8):1391–1402. PubMed PMID: 24991838; PubMed Central PMCID: PMC4203516.
- Xie X, Koh JY, Price S, et al. Atg7 overcomes senescence and promotes growth of brafV600E-driven melanoma. Cancer Discov. 2015 Apr;5(4):410–423. PubMed PMID: 25673642; PubMed Central PMCID: PMC4390491.
- Saric A, Grinstein S, Freeman SA. Measurement of autolysosomal pH by dual-wavelength ratio imaging. Methods Enzymol. 2017;588:15–29. PubMed PMID: 28237098.
- Ohkuma S, Poole B. Fluorescence probe measurement of the intralysosomal pH in living cells and the perturbation of pH by various agents. Proc Natl Acad Sci U S A. 1978 Jul;75(7):3327–3331. PubMed PMID: 28524; PubMed Central PMCID: PMC392768
- Crowley LC, Christensen ME, Waterhouse NJ. Measuring survival of adherent cells with the colony-forming assay. Cold Spring Harb Protoc. 2016 Aug 1;2016(8). DOI:10.1101/pdb.prot087171 PubMed PMID: 27480717.