ABSTRACT
The de novo synthesis of autophagic vesicles is strongly dependent on sufficient lipid supply. Recently, the RAB GTPase RAB18 was shown to affect autophagy by mediating fatty acid release from lipid droplets, which are lipid sources for autophagosome formation. The stable loss of RAB18 interfered with fatty acid release from the lipid reservoirs and provoked autophagy network adaptations aiming to maintain autophagic activity under lipid limiting conditions.
Macroautophagy/autophagy is the key eukaryotic, lysosomal-dependent degradation pathway responsible for the removal and recycling of cytosolic components. The efficient function of autophagy, under constantly changing conditions, is essential for cellular homeostasis, cell function, and survival, and its dysfunction is linked to aging and several disorders [Citation1].
A central step of autophagy is the selective sorting of degradable materials into autophagosomes, which results in cargo separation from the cytosol. This separation enables the targeted mobilization of the autophagosome and its fusion with the lysosomes. Autophagosome formation starts with an ER-borne precursor membrane, the phagophore, which completely elongates around the cargo until this lipid-protein vesicle seals [Citation2]. While the protein network (ATG proteins) involved is well characterized [Citation3], the lipid sources of this de novo vesicle synthesis are still ambiguous, which include the ER, plasma membrane, mitochondria, the Golgi complex, the ER-Golgi intermediate compartment, recycling endosomes, as well as lipid droplets (LDs) [Citation4–Citation7]. Autophagic vesicles are constantly generated; however, their total numbers massively rise upon autophagy induction caused by conditional changes, such as starvation or disturbances in proteostasis. Thus, cellular autophagic protein activity, as well as the availability of lipids required for sufficient autophagosome formation, have to be instantly adjusted to the actual requirements.
Previously, we have characterized the RAB GTPase RAB18, as well as the RAB3GAP complex, as positive modulators of autophagy [Citation8–Citation10]. RAB18 is one of the most highly conserved and widely expressed RAB GTPases in different tissues throughout the human body. The protein displays a broad range of functions, including the involvement in the ER-Golgi vesicle trafficking, endocytosis, immune evasion, or the modulation of the secretory response [Citation11]. However, the best-characterized activity of RAB18 is its function in the metabolism of LDs, which includes its role in LD biogenesis and/or consumption [Citation12–Citation14]. The RAB3GAP complex consists of the enzymatic subunit RAB3GAP1 and the regulatory subunit RAB3GAP2 and represents an untypical RAB GTPase regulating protein since it exists as a dimeric complex. It is the only RAB GAP without the otherwise typical TBC (USP6/TRE2, TBC1D1/BUB2, CDC16) domain, and shows the name-giving GAP activity, but was recently characterized also to possess an additional GEF activity. RAB GEFs activate the RAB GTPases, and the RAB3GAP complex indeed acts as a GEF on RAB18 [Citation15]. This finding directly links RAB18 function and the RAB3GAP complex, also reflected by the fact that loss-of-function mutations in all three genes cause indistinguishable forms of the Warburg Micro syndrome, a severe (neuro-) developmental disorder [Citation16].
In a recent study, we have investigated the detailed molecular impact of RAB18 function on basal and starvation-induced autophagy and observed that this RAB GTPase affected the degradative pathway by modulating the release of fatty acids from LDs, resulting in a limited lipid availability for autophagosome formation in the absence of RAB18 [Citation17]. In fact, a very current study has demonstrated the importance of free fatty acids for autophagosome formation in yeast [Citation18]. Activated fatty acids are modified to phospholipids within the ER and are channeled to the phagophore to support its elongation.
Fluorescence and electron microscopy revealed that human RAB18 knockout cells showed a striking LD appearance. The lipid reservoirs accumulated in the perinuclear region of the cell and were enlarged in size and reduced in number when compared to wild type cells. Importantly, RAB3GAP1 knockout cells showed a similar LD phenotype, emphasizing the importance of RAB18 function in LD turnover. This significant LD appearance has also been observed by other studies describing perturbations in LD metabolism [e.g. [Citation14],]. Tracing dye-conjugated fatty acids and analyzing the induced consumption of LDs under starvation demonstrated that the fatty acid mobilization from the lipid reservoirs was strongly reduced in the absence of RAB18. However, the oleic acid-stimulated formation of LDs was unaffected. Thus, the loss of RAB18 resulted in inert LDs that were unable to release fatty acids efficiently. Importantly, so far, the only cellular alteration determined for Warburg Micro syndrome patient cells carrying loss-of-function mutations in RAB18 or RAB3GAP1 is an impact on LD metabolism [Citation19], which resembles the results obtained in our RAB18 and RAB3GAP1 KO cells.
Because autophagosome formation is strongly dependent on adequate lipid supply, the reduced LD-derived lipid availability upon the loss of RAB18 function indirectly affected autophagy. Combining SILAC-based phospho-proteomics, qPCR array, and immunoblot analyzes revealed that distinct factors of the autophagy network were altered to compensate for the reduced lipid availability and nevertheless maintain autophagic activity ().
Figure 1. Lipid droplet-derived lipids support autophagosome formation. The reduced fatty acid release from LDs upon RAB18 knockout provokes autophagy network adaptations aiming to maintain autophagic activity under lipid limiting conditions. The adjustments comprise augmented expression and phosphorylation of ATG2B, increased formation of the ATG12–ATG5 conjugate, as well as enhanced phosphorylation of ATG9A, resulting in an increased ATG9A trafficking. These alterations emphasize an exciting on-demand autophagy network plasticity to ensure autophagic activity under unfavorable conditions.
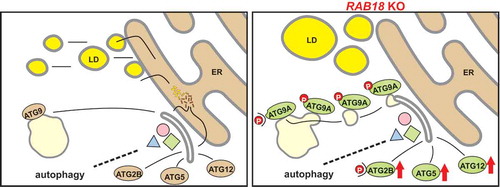
At the level of ATGs, one striking alteration observed in RAB18 KO cells was increased ATG12–ATG5 conjugate levels, which were based on the enhanced expression of both proteins. By a ubiquitin-like conjugation system, ATG12 and ATG5 are covalently linked, which is a pre-requisite for the formation of the ATG12–ATG5-ATG16L1 complex [Citation20]. This complex localizes to the phagophore and serves for the last step of the second ubiquitin-like conjugation process of autophagy, linking phosphatidylethanolamine to Atg8 proteins, promoting autophagosome formation. However, in the absence of ATG5, elongated phagophore structures and autophagosome-like vesicles are still detected, although at strongly decreased levels [Citation21]. This report emphasizes the importance of both conjugation systems for efficient autophagosome formation but also indicates that their absence can be partially compensated. Importantly, increased ATG12–ATG5 conjugate levels enhance autophagic activity [Citation22]. Therefore, the observed increased conjugate levels may directly support the maintenance of autophagic activity upon lipid-limiting conditions.
A second significant modification of the ATGs uncovered upon reduced lipid availability was the enhanced expression and altered phosphorylation of ATG2B at amino acid residue S493. Mammalian cells possess two functionally redundant ATG2 proteins, ATG2A and ATG2B. Recently, the ATG2 proteins have been characterized as lipid transfer and/or membrane tethering factors, which support autophagosome formation by mediating the lipid transfer from the ER to the growing phagophore [Citation23–Citation25]. Thus, modulating the expression levels of ATG2B appears as a direct functional response to the limitations in lipid availability. Remarkably, ATG2 proteins have also been shown to localize to LDs, and their transient knockdown results in the accumulation of enlarged lipid reservoirs [Citation26], indicating that ATG2 proteins may also modulate the metabolism of LDs. The functional consequence of the enhanced phosphorylation of residue S493 of ATG2B is not characterized yet, and the responsible kinase is unclear. However, the conserved ATG2 protein in yeast is phosphorylated by ULK1/Atg1 kinase [Citation27], highlighting this autophagy kinase as a potential modifier.
Finally, a third adaptive alteration in the ATG network could be assigned to ATG9A. ATG9A is the only transmembrane protein of the autophagy network and responsible for vesicle transfer from the cellular periphery to the site of autophagosome formation, delivering proteins and lipids required for the generation and/or elongation of the phagophore [Citation5,Citation28,Citation29]. Under basal autophagy conditions, ATG9A is mainly located at the Golgi complex and partially in the endosomal compartment. Upon autophagy induction, the Golgi-association of ATG9A decreases, and the transmembrane protein is mostly found in a vesicular population, showing an enhanced trafficking activity, which corresponds to an increased autophagosome formation. The activity of ATG9A is regulated via ULK1 and SRC kinase-mediated phosphorylation at the amino acid residues S14 and Y8, respectively [Citation27,Citation30]. Both residues show increased phosphorylation under induced autophagy conditions. Importantly, in RAB18 KO, as well as RAB3GAP1 KO cells, the ATG9A phosphorylation was enhanced already under basal autophagy conditions, which was linked to an augmented ATG9A trafficking and enhanced numbers of phagophore assembly sites. The pharmacological inhibition of SRC kinase activity reduced Y8 phosphorylation and resulted in the localization of the majority of ATG9A at the Golgi complex, confirming the importance of this modification for ATG9A trafficking. Notably, decreasing ATG9A trafficking in RAB18 KO cells resulted in a clear reduction in autophagic activity, emphasizing that the enhanced activity of ATG9A is important for the maintenance of autophagy under lipid limiting conditions.
Thus, in our study, we conclude that RAB18 affects autophagy by modulating the lipid transfer via LD-derived fatty acids to the site of autophagosome formation. In combination, the identified alterations of autophagy network factors were sufficient to maintain basal autophagic activity under lipid limiting conditions. The network adaptations displayed fascinating on-demand plasticity, which, importantly, was independent of the autophagy induction system. However, increased autophagy rates upon starvation, characterized by an elevated lipid demand, were not possible, which exposes limitations in autophagy network adaptations to this particular condition and emphasizes the importance of LD-derived lipids for autophagosome formation.
Abbreviations
Table
Acknowledgments
This work is supported by grants in the framework of Collaborative Research Center CRC1177 of the DFG to AK and CB.
Disclosure statement
No potential conflict of interest was reported by the authors.
References
- Yang Y, Klionsky DJ. Autophagy and disease: unanswered questions. Cell Death Differ. 2020 Jan 3;27(3):858–871.
- Hayashi-Nishino M, Fujita N, Noda T, et al. A subdomain of the endoplasmic reticulum forms a cradle for autophagosome formation. Nat Cell Biol. 2009 Dec;11(12):1433–1437.
- Behrends C, Sowa ME, Gygi SP, et al. Network organization of the human autophagy system. Nature. 2010 Jul 1;466(7302):68–76.
- Dupont N, Chauhan S, Arko-Mensah J, et al. Neutral lipid stores and lipase PNPLA5 contribute to autophagosome biogenesis. Curr Biol. 2014 Mar 17;24(6):609–620.
- Mercer TJ, Gubas A, Tooze SA. A molecular perspective of mammalian autophagosome biogenesis. J Biol Chem. 2018 Apr 13;293(15):5386–5395.
- Rubinsztein DC, Shpilka T, Elazar Z. Mechanisms of autophagosome biogenesis. Curr Biol. 2012 Jan 10;22(1):R29–34.
- Shpilka T, Welter E, Borovsky N, et al. Lipid droplets and their component triglycerides and steryl esters regulate autophagosome biogenesis. Embo J. 2015 Aug 13;34(16):2117–2131.
- Feldmann A, Bekbulat F, Huesmann H, et al. The RAB GTPase RAB18 modulates macroautophagy and proteostasis. Biochem Biophys Res Commun. 2017 May 6;486(3):738–743.
- Kern A, Dikic I, Behl C. The integration of autophagy and cellular trafficking pathways via RAB GAPs. Autophagy. 2015;11(12):2393–2397.
- Spang N, Feldmann A, Huesmann H, et al. RAB3GAP1 and RAB3GAP2 modulate basal and rapamycin-induced autophagy. Autophagy. 2014;10(12):2297–2309. .
- Dejgaard SY, Presley JF. Rab18: new insights into the function of an essential protein. Cell Mol Life Sci. 2019 May;76(10):1935–1945.
- Martin S, Driessen K, Nixon SJ, et al. Regulated localization of Rab18 to lipid droplets: effects of lipolytic stimulation and inhibition of lipid droplet catabolism. J Biol Chem. 2005 Dec 23;280(51):42325–42335.
- Pulido MR, Diaz-Ruiz A, Jimenez-Gomez Y, et al. Rab18 dynamics in adipocytes in relation to lipogenesis, lipolysis and obesity. PLoS One. 2011;6(7):e22931. .
- Xu D, Li Y, Wu L, et al. Rab18 promotes lipid droplet (LD) growth by tethering the ER to LDs through SNARE and NRZ interactions. J Cell Biol. 2018 Mar 5;217(3):975–995.
- Gerondopoulos A, Bastos RN, Yoshimura S, et al. Rab18 and a Rab18 GEF complex are required for normal ER structure. J Cell Biol. 2014 Jun 9;205(5):707–720.
- Handley MT, Aligianis IA. RAB3GAP1, RAB3GAP2 and RAB18: disease genes in Micro and Martsolf syndromes. Biochem Soc Trans. 2012 Dec 1;40(6):1394–1397.
- Bekbulat F, Schmitt D, Feldmann A, et al. RAB18 loss interferes with lipid droplet catabolism and provokes autophagy network adaptations. J Mol Biol. 2019 Dec 24;432(4):1216–1234.
- Schutter M, Giavalisco P, Brodesser S, et al. Local fatty acid channeling into phospholipid synthesis drives phagophore expansion during autophagy. Cell. 2020 Jan 9;180(1):135–149 e14.
- Liegel RP, Handley MT, Ronchetti A, et al. Loss-of-function mutations in TBC1D20 cause cataracts and male infertility in blind sterile mice and Warburg micro syndrome in humans. Am J Hum Genet. 2013 Dec 5;93(6):1001–1014.
- Hanada T, Noda NN, Satomi Y, et al. The Atg12-Atg5 conjugate has a novel E3-like activity for protein lipidation in autophagy. J Biol Chem. 2007 Dec 28;282(52):37298–37302.
- Mizushima N. The ATG conjugation systems in autophagy. Curr Opin Cell Biol. 2019 Dec;31(63):1–10.
- Pyo JO, Yoo SM, Ahn HH, et al. Overexpression of Atg5 in mice activates autophagy and extends lifespan. Nat Commun. 2013;4:2300.
- Osawa T, Ishii Y, Noda NN. Human ATG2B possesses a lipid transfer activity which is accelerated by negatively charged lipids and WIPI4. Genes Cells. 2020 Jan;25(1):65–70.
- Osawa T, Kotani T, Kawaoka T, et al. Atg2 mediates direct lipid transfer between membranes for autophagosome formation. Nat Struct Mol Biol. 2019 Apr;26(4):281–288.
- Valverde DP, Yu S, Boggavarapu V, et al. ATG2 transports lipids to promote autophagosome biogenesis. J Cell Biol. 2019 Apr 5;218(6):1787–1798.
- Velikkakath AK, Nishimura T, Oita E, et al. Mammalian Atg2 proteins are essential for autophagosome formation and important for regulation of size and distribution of lipid droplets. Mol Biol Cell. 2012 Mar;23(5):896–909. .
- Papinski D, Schuschnig M, Reiter W, et al. Early steps in autophagy depend on direct phosphorylation of Atg9 by the Atg1 kinase. Mol Cell. 2014 Feb 6;53(3):471–483.
- Imai K, Hao F, Fujita N, et al. Atg9A trafficking through the recycling endosomes is required for autophagosome formation. J Cell Sci. 2016 Oct 15;129(20):3781–3791.
- Judith D, Jefferies HBJ, Boeing S, et al. ATG9A shapes the forming autophagosome through Arfaptin 2 and phosphatidylinositol 4-kinase IIIbeta. J Cell Biol. 2019 May 6;218(5):1634–1652.
- Zhou C, Ma K, Gao R, et al. Regulation of mATG9 trafficking by Src- and ULK1-mediated phosphorylation in basal and starvation-induced autophagy. Cell Res. 2017 Feb;27(2):184–201.