ABSTRACT
Macroautophagy/autophagy was recently shown to regulate unconventional protein secretion through a process called secretory autophagy. How the secretory cargo selectively enters into the secretory autophagosome has been a central question. Our recent studies indicate that cargo translocation into the ER-Golgi intermediate compartment, a compartment contributing membranes to the forming autophagosome, acts as a mechanism for secretory cargo entry into the vesicle and may be an early step for secretory autophagy.
A new function of autophagy, i.e. protein secretion, was identified recently. Multiple studies indicate that autophagy is involved in the secretion of cytosolic proteins without a signal peptide (leaderless cargoes) through unconventional protein secretion (UCPS), which was named as opposed to the well-known signal peptide-dependent and ER-Golgi trafficking-mediated conventional secretion. Evidence from imaging and membrane fractionation experiments implicate that a secretory autophagosome carries and delivers UCPS cargoes, including IL1B/interleukin-1β, SNCA/α-synuclein and yeast Acb1, out of the cell and meanwhile bypasses ER-Golgi trafficking. A question looms large as the study goes deep: how do the leaderless cargoes become sequestered within the autophagosome?
Autophagy receptors, e.g., SQSTM1/p62 or CALCOCO2/NDP52, regulate the selective incorporation of cytosolic components into the phagophore, the precursor of the autophagosome, for subsequent degradation. Deducing from this notion, it is conceivable that specific receptors may also determine the selective encapsulation of UCPS cargoes by the phagophore. If this is true, the UCPS cargoes will be engulfed in a similar manner to that which occurs during autophagy-mediated degradation, and the cargoes should localize in the inner lumen of the mature double-membrane autophagosome. At the time that the autophagosome outer membrane fuses with the plasma membrane, the UCPS cargoes should be released in the form of a vesicle comprised of the autophagosome inner membrane. However, our data indicated something different. First, blocking autophagosome formation does not affect the entry of the UCPS cargo IL1B into the vesicle; Second, the topological distribution of IL1B seems to be the inter-membrane lumen instead of the inner membrane lumen of the autophagosome; Third, IL1B is released in the form of a soluble protein rather than being contained within a vesicle (unpublished data).
If it is not engulfed by the phagophore, how does a UCPS cargo enter into the vesicle? We found that IL1B enters into the vesicle through a translocation process which is dependent on protein-unfolding and the assistance of a chaperone protein, HSP90. As a result of our effort to search for the channel involved in protein translocation, we identified a single-spanning membrane protein, TMED10 (transmembrane p24 trafficking protein 10) involved in IL1B secretion. Importantly, in addition to IL1B, TMED10 also regulates the release of many other cytosolic UCPS cargoes including the IL1 family members, CRYAB/HSPB5, MAPT/Tau and ANXA1 (annexin A1). Sequence analysis indicates a common motif, likely to be a UCPS signal peptide, which accounts for the selectivity of the TMED10-mediated UCPS. The mechanism seems to involve a direct interaction of the C terminus of TMED10 with the motif in the UCPS cargo. Using an in vitro reconstitution assay, we found that TMED10 directly translocates UCPS cargoes into a liposome, indicating that TMED10 is a protein channel. Oligomerization of TMED10 happens during translocation, which explains how a single-membrane protein operates as a channel. Incorporation of the cytosolic HSP90AB1 and lumenal HSP90B1/GRP94 dramatically enhance the efficiency of translocation (). In summary, the protein translocation system includes a protein channel and at least two chaperone proteins, which resembles the pathway of cargo translocation into the lysosome in chaperone-mediated autophagy (CMA) identified by Dr. Cuervo and Dr. Dice.
Figure 1. Possible routes of secretory autophagy. Cytosolic UCPS cargoes bind to HSP90AB1, which likely unfolds the cargo. The cargo binds to the ERGIC membrane protein TMED10 and triggers TMED10 oligomerization to form a protein channel. With aid of a lumenal chaperone HSP90B1/GRP94, the cargo is translocated into the lumen of the ERGIC. In this way, the cytosolic cargo enters into the membrane carrier. The ERGIC generates a vesicle containing the cargo, which likely depends on COPII and possibly contains SEC22B and TRIM16. The SEC22B-TRIM16 complex may direct the vesicle to form a secretory autophagosome, which may directly fuse with the plasma membrane or transits through the secretory endolysosomal compartment as an intermediate step. It is also possible that the ERGIC-derived vesicle targets to the plasma membrane without going to the secretory autophagosome. Fusion of these vesicles/compartments with the plasma membrane is likely to be completed by pairing of SEC22B with STX3-STX4 and SNAP23-SNAP29. GORASP2 is involved in multiple types of UCPS and may act to facilitate the targeting of an upstream vesicle/compartment to the downstream one through membrane tethering.
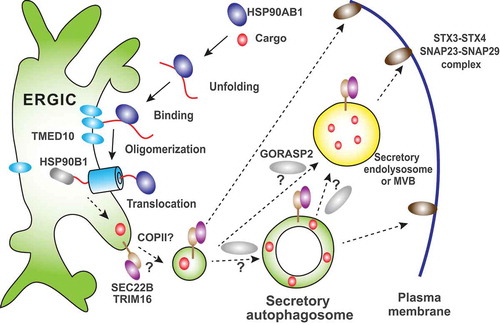
TMED10 is an ER-Golgi intermediate compartment (ERGIC)-localized protein. Indeed, we found the transport of UCPS cargoes into the ERGIC during the secretion process. The data indicate that the TMED10-mediated translocation of UCPS cargoes into the ERGIC is an initial step for UCPS. So, what is the connection to secretory autophagy? Coincidentally, we previously found that the ERGIC is a membrane source for the forming autophagosome, and acts by generation of a special class of COPII vesicles, the ERGIC-COPII vesicles. Therefore, the ERGIC itself may be a hub linking UCPS cargo entry to secretory autophagy by incorporating UCPS cargoes and meanwhile generating autophagosomal precursors.
Based on this model, we pose the following question: How does the ERGIC differentiate precursors for degradative and secretory autophagosomes? Recently, the Deretic lab found that TRIM16 binds the UCPS cargo IL1B and regulates its entry into phagophores that will become secretory autophagosomes. Furthermore, the SNARE protein SEC22B associates with TRIM16 and is involved in regulating the targeting of the secretory autophagosome to the plasma membrane by pairing with STX3 (syntaxin 3)-STX4 as well as SNAP23-SNAP29. Notably, SEC22B is a SNARE enriched in the ERGIC. Therefore, one possible answer to the question is that the ERGIC SNARE SEC22B and the binding partner TRIM16 may cooperate to sort UCPS cargoes into a vesicle that contributes to secretory autophagosome biogenesis (). Although SEC22B could be a good explanation for determining the secretory role of the autophagosome, it is unknown if secretory autophagosomes are physically different from degradative autophagosomes and, if so, how secretory autophagosomes avoid incorporation of autophagic cargoes for degradation, e.g. a damaged mitochondrion.
The ERGIC is a tubulo-vesicular compartment closely associated with the ER-exit sites (ERES). Interestingly, the Malhotra lab identified a special compartment, called the compartment for unconventional protein secretion (CUPS), which regulates UCPS of Acb1 in yeast. The CUPS localizes adjacent to the ERES and is characterized by a concentric structure made of tubular membranes that may contain parts of the ER, the autophagosome and the endosome. The similarity of function, localization and morphology between the CUPS and the ERGIC indicates that they may be functional equivalents in the case of UCPS. However, compared to the CUPS, the ERGIC is multifunctional, including ER-Golgi trafficking and biogenesis of secretory and degradative autophagosomes. To accommodate these multiple roles, the ERGIC may be sub-compartmentalized, and the possibility of this scenario needs further investigation. It has been shown that GORASP2/GRASP55 (yeast Grh1) plays an essential role in regulating the formation of the CUPS (and UCPS in yeast). In mammals, GORASP2 is a Golgi-localized protein that is also involved in multiple sorts of UCPS as well as fusion between the autophagosome and the lysosome. It is likely that the GORASP2 may act downstream of the ERGIC (), but the exact crosstalk is pending further investigation in the future.
Acknowledgments
The work is funded by National Key R&D Program of China (2019YFA0508602), National Natural Science Foundation of China (91854114, 31872832, 31872826, 31741082), State Key Laboratory of Membrane Biology, Tsinghua Independent Research Program (2019Z06QCX02), Tsinghua-Peking Center for Life Sciences, and Thousand Young Talents Program.
Disclosure statement
No potential conflict of interest was reported by the authors.