ABSTRACT
Macroautophagy/autophagy can play a cytoprotective role after photodynamic damage to malignant cells, depending on the site of subcellular damage initiated by reactive oxygen species. There is evidence for such protection when mitochondria are among the targets. Targeting lysosomes has been reported to be more effective for photokilling, perhaps because autophagy offers no cytoprotection. Photodynamic damage to both lysosomes and mitochondria can, however, markedly enhance the overall level of photokilling. Two mechanisms have been proposed to account for this result. Lysosomal photodamage leads to the release of calcium ions, resulting in the activation of the protease CAPN (calpain). CAPN then cleaves ATG5 to a fragment (tATG5) capable of interacting with mitochondria to enhance pro-apoptotic signals. It has also been proposed that targeting lysosomes for photodynamic damage can impair mitophagy, a process that could mitigate the pro-apoptotic effects of mitochondrial targeting. The level of lysosomal photodamage required for suppression of mitophagy is unclear. The “tATG5 route” involves the catalytic action of CAPN, activated by a degree of lysosomal photodamage barely detectible by a viability assay. ER photodamage can also initiate paraptosis, a death pathway functional even in cell types with impaired apoptosis and apparently unaffected by autophagy.
Abbreviations: ALLN: N-acetyl-Leu-Leu-norleucinal (cell-permeable inhibitor of calpain); ATG: autophagy related; BPD: benzoporphyrin derivative (Visudyne); ER: endoplasmic reticulum; EtNBS: 5-ethylamino-9-diethyl-aminobenzo[a]phenothiazinium chloride; MTT: a tetrazolium dye; NPe6: mono N-aspartyl chlorin e6; PDT: photodynamic therapy; ROS: reactive oxygen species
After a few billion years of evolution, it is perhaps not surprising that many molecules involved in biologic processes can play multiple roles. For example, ATP is involved in energy transfer reactions, is a neurotransmitter and also a component of RNA and (with a reduced sugar molecule) DNA. This is also true for certain proteins associated with autophagy. Two examples of this phenomenon relate to cellular responses to photodynamic therapy (PDT).
PDT involves the interaction of light, a photosensitizing agent and molecular oxygen [Citation1]. Irradiation of cells can cause lethal damage when reactive oxygen species (ROS) are formed at sub-cellular sites where the photosensitizer has accumulated. Autophagy can be cytoprotective, indicated by a ’shoulder’ on the dose-response curve. A typical such curve is shown in . This illustrates the effect on survival (via clonogenic analysis) of increasing light doses on cells photosensitized with benzoporphyrin derivative (Visudyne, BPD), an agent that localizes in mitochondria and ER [Citation2]. When autophagy is impaired by silencing ATG5 or ATG7, the shoulder is lost and PDT efficacy enhanced.
Figure 1. Dose-response data from experiments involving 1c1c7 murine hepatoma cells. (A) Response to photokilling (BPD) targeting mitochondria + ER. (B) Response to photokilling that targets lysosomes (NPe6): wild type (●), ATG5 KD (○), ATG7 KD (▲). (Adapted from Refs. 2 and 3). Data represent clonogenic survival normalized to the plating efficiency of controls
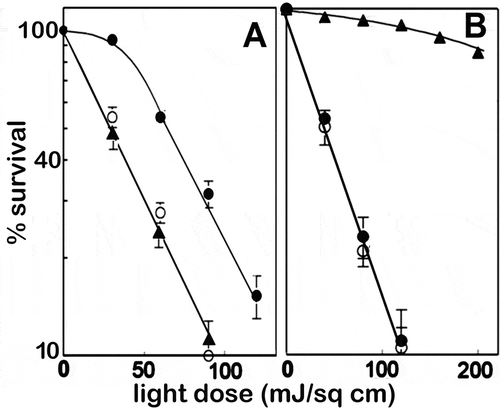
In contrast, when lysosomes are the sole PDT target, there is no shoulder on the dose-response curve and silencing of ATG5 has no effect [Citation3]. This is illustrated in . We interpret these data to indicate that autophagy has no cytoprotective effect: photodamaged lysosomes apparently cannot be sequestered before photodamage leads to apoptosis and cell death [Citation4]. Absence of such a cytoprotective effect could explain why photodynamic effects on lysosomes can be more lethal, at a given light dose, than when mitochondria are targeted [Citation5,Citation6].
Loss of ATG7 has a different effect, leading to a greatly reduced phototoxic response (). The latter is based on the release of lysosomal proteases (cathepsins) that subsequently cleave the protein BID (BH3-interacting-domain death agonist) to a truncated form (tBID). Interactions between tBID and mitochondria promote the release of CYCS (cytochrome c, somatic), leading to apoptosis and cell death [Citation4,Citation7]. Loss of ATG7 prevents release of large molecules, e.g., cathepsins, from damaged lysosomes [Citation2].
Another example of a multiple role for an autophagy-associated protein was provided by Simon’s group. Release of Ca2+ from lysosomes into the cytoplasm activates CAPN. Among the targets of the resulting proteolytic activity is the protein ATG5, resulting in the formation of a truncated molecule (tATG5) that can bind to mitochondria and promote apoptosis [Citation8]. This effect explains the ability to target both lysosomes and mitochondria for PDT-induced photodamage to substantially promote tumor eradication. The chlorin NPe6 [Citation9] and the thiazine EtNBS [Citation10] both target lysosomes for photodynamic damage while BPD targets mitochondria + ER [Citation11]. A 1996 report demonstrated that co-administration of EtNBS + BPD, followed by irradiation, markedly promoted the efficacy of PDT in tumor-bearing rats, extending the depth of killing to 10 mm from the tumor surface [Citation12]. This is substantially greater than the expected 3–5 mm depth of PDT-induced tumor eradication [Citation13]. As predicted by Simon’s report [Citation8], lysosomal photodamage led to a CAPN-mediated cleavage of ATG5 to a truncated form (tATG5). This resulted in an enhanced pro-apoptotic effect when coupled with PDT-induced damage to mitochondria [Citation10,Citation11,Citation14,Citation15].
Some photosensitizing agents have “dark” effects at high concentrations, resulting in impaired autophagy. This effect, unrelated to light-mediated reactions, has been observed for both BPD [Citation16], discussed in Ref. 2, and NPe6 [Citation17], discussed in Ref. 15. While concentrations needed are in excess of what is required for lethal photodynamic effects, these reports illustrate the multiple interactions that drugs can have with biologic systems. NPe6 poorly penetrates cell membranes, so a relatively high (~20 μM) level is needed to initiate lysosomal photodamage. When the concentration was increased to a level (40 μM) that inhibits autophagy [Citation17], there was no effect on the ability of lysosomal photodamage to markedly promote PDT efficacy [Citation2]. This result provides additional evidence that autophagy is not involved in enhanced photokilling when lysosomes and mitochondria are PDT targets.
Other studies suggesting a role of lysosomes for potentiation of PDT efficacy have been reported. Exposure of malignant cells to bafilomycin or chloroquine promotes lethality of PDT directed at mitochondria by raising the lysosomal pH and altering lysosomal permeability barriers. This leads to migration of lysosomal Fe++ to mitochondria and an increased formation of cytotoxic hydroxyl radicals via Fenton chemistry [Citation18,Citation19]. Using a fluorescent probe for ferrous iron, we confirmed this observation in hepatoma 1c1c7 cells. PDT-induced photodamage to lysosomes was, however, unable to promote Fe++ migration from lysosomes to mitochondria [Citation20]. Another report indicated that simultaneous targeting of Golgi and lysosomes also promoted PDT-induced apoptotic death, although the pertinent mechanism remains unknown [Citation21,Citation22].
Baptista’s group proposed a role for autophagy in the promotion of efficacy of therapeutics involving mitochondrial and lysosomal targets. The ability of mitophagy to protect cells from lethal effects of drug-induced mitochondrial damage was reversed by bafilomycin or chloroquine, agents that impair the activity of lysosomal proteases [Citation23]. Enhanced efficacy of cytotoxic drugs that targeted both mitochondria and lysosomes was attributed to interference with the cytoprotective action of mitophagy [Citation23]. These studies were recently extended to include photodynamic effects on both mitochondria and lysosomes [Citation24]. It was noted that silencing ATG5 did not alter the lethal effects of when lysosomes were targeted. This agrees with our studies, illustrated in [Citation7]. Ref. 24 describes enhanced PDT efficacy, when mitochondrial and lysosomes are targeted, as a demonstration of “autophagy-associated cell death”. This term may be imprecise since the synergistic effect is said to result from the impairment of mitophagy.
A test proposed for assessing the role of autophagy incorporated three measurements: neutral red and crystal violet labeling and an MTT assay [Citation25]. From the results, an estimate of the role of “autophagic death” was derived. Neither of the first two assays unambiguously measures effects on cellular proliferation, something that can be readily determined by clonogenic studies. The assumption is that averaging the results of two imprecise tests can provide a better indication than either alone. The MTT assay, a measure of the activity of mitochondrial dehydrogenases, may be correlated with apoptotic death resulting from cytochrome c release, but results can be affected by delayed toxicity or repair of mitochondrial damage. Uptake of normally impermeable dyes, e.g., propidium iodide or crystal violet, can be a reliable index of survival if necrosis or cell fragmentation is involved. Use of neutral red for assessing autophagy is questionable since this dye can also label any acidic non-autophagic vacuole and has other limitations [Citation26]. Moreover, neutral red uptake is impaired by cell death [Citation27]. In studies described in Ref. 14, we employed CYTO-ID (ENZO), a probe that appears to be more appropriate for monitoring autophagy [Citation26].
While impaired mitophagy may play a role in the improved efficacy of combination PDT, several studies have confirmed a role for ATG5. Formation of tATG5 was observed after targeting of lysosomes [Citation15]. Silencing ATG5 did not affect the ability of lysosomal targeting to initiate apoptosis via conversion of BID to a pro-apoptotic tBID fragment [Citation4]. But loss of ATG5 did abolish the ability of lysosomal photodamage to promote photokilling resulting from mitochondrial photodamage [Citation10]. The calpain antagonist ALLN had the same effect [Citation28]. Prior or simultaneous lysosomal targeting markedly enhanced the ability of PDT aimed at mitochondria to promote apoptosis as indicated by increased CASP3 (caspase 3) activation [Citation10,Citation11,Citation15,Citation20,Citation28]. With regard to the role of ATG5, this protein is apparently not an obligatory element for all stages of mitophagy [Citation29]. Silencing ATG5 may not necessarily abolish any pro-survival effects of mitophagy.
In the initial combination PDT studies, two photosensitizers were employed for targeting both mitochondria and lysosomes [Citation10–12]. BPD is commonly administered as a liposomal formulation that targets mitochondria and ER. If BPD is conjugated to a lipid before incorporation into liposomes, drug accumulation then occurs via an endosomal process leading to lysosomal localization. A combination of free BPD + lipid-anchored BPD incorporated into liposomes can, therefore, target both mitochondria and lysosomes using a single agent. Using this approach in 2- and 3-D culture systems, it was found that a barely perceptible level of PDT-induced damage to lysosomes markedly potentiated the pro-apoptotic effects of PDT directed at mitochondria [Citation30,Citation31]. This formulation extends the targeting range of BPD and eliminates the need for more than one wavelength of light.
An additional factor in the efficacy of photokilling by BPD is the simultaneous targeting of ER. This activates a death pathway termed paraptosis, characterized by a network of vacuoles that gradually fills the entire cytoplasmic space [Citation32,Citation33]. In cell lines we have studied, ER photodamage led to the appearance of paraptosis [Citation14]. The cytoplasmic vacuoles lack the double membranes associated with autophagy and can also be distinguished from autophagosomes by staining techniques [Citation14,Citation15]. Paraptosis appears to be a functional death pathway for cells with an impaired apoptotic response [Citation34]. A role for autophagy in modulating paraptosis has yet to be examined. Preliminary studies have detected no shoulder on the dose-response curve involving PDT directed at ER, suggesting that, as in the case of lysosomes, autophagy is not cytoprotective. Ref. 24 misinterprets the implications of studies identifying apoptosis as a late consequence of ER photodamage [Citation14]. The pathway to paraptosis is unrelated to caspase activation or other elements of the apoptotic process [Citation32,Citation33]. At very high light doses, cross-linking of ER proteins was detected and paraptosis impaired. Cross-linking and the resulting immobilization of ER proteins will likely interfere with the mobility necessary for vacuole formation. At such supra-lethal PDT doses, other death pathways were detected: apoptosis and necrosis [Citation34,Citation35]. It seems likely that high levels of irradiation can lead to “off-target” effects, involving mitochondria (leading to apoptosis) or the plasma membrane (resulting in necrosis).
Disclosure statement
No potential conflicts of interest were disclosed.
Additional information
Funding
References
- Cengel KA, Simone CB 2nd, Glatstein E. PDT: what’s past is prologue. Cancer Res. 2016;76:2497–2499.
- Andrzejak M, Price M, Kessel DH. Apoptotic and autophagic responses to photodynamic therapy in 1c1c7 murine hepatoma cells. Autophagy. 2011;7::979–984.
- Kessel D, Price M, Reiners JJ Jr. ATG7 deficiency suppresses apoptosis and cell death induced by lysosomal photodamage. Autophagy. 2012;8:1333–1341.
- Reiners JJ Jr, Caruso JA, Mathieu P, et al. Release of cytochrome c and activation of pro-caspase-9 following lysosomal photodamage involves Bid cleavage. Cell Death Differ. 2002;9:934–944.
- Rodriguez ME, Zhang P, Azizuddin K, et al. Structural factors and mechanisms underlying the improved photodynamic cell killing with silicon phthalocyanine photosensitizers directed to lysosomes versus mitochondria. Photochem Photobiol. 2009;85:1189–1200.
- Tsubone TM, Martins WK, Pavani C, et al. Enhanced efficiency of cell death by lysosome-specific photodamage. Sci Rep. 2017;7:6734.
- Kessel D, Luo Y, Mathieu P, et al. Determinants of the apoptotic response to lysosomal photodamage. Photochem Photobiol. 2000;71:196–200.
- Yousefi S, Perozzo R, Schmid I, et al. Calpain-mediated cleavage of Atg5 switches autophagy to apoptosis. Nat Cell Biol. 2006;8(10):1124–1132.
- Roberts WG, Liaw LH, Berns MW. In vitro photosensitization II. An electron microscopy study of cellular destruction with mono-L-aspartyl chlorin e6 and photofrin II. Lasers Surg Med. 1989;9:102–108.
- Kessel D, Reiners JJ Jr. Promotion of proapoptotic signals by lysosomal photodamage. Photochem Photobiol. 2015;91:931–936.
- Kessel D, Reiners JJ Jr. Effects of combined lysosomal and mitochondrial photodamage in a non-small-cell lung cancer cell line: the role of paraptosis. Photochem Photobiol. 2017;93:1502–1508.
- Cincotta L, Szeto D, Lampros E, et al. Benzophenothiazine and benzoporphyrin derivative combination phototherapy effectively eradicates large murine sarcomas. Photochem Photobiol. 1996;63:229–237.
- Wilson BC. Photodynamic therapy: light delivery and dosage for second generation photosensitizers. In: Bock G, Harnett SM, editors. Ciba foundation symposium 146 photosensitizing compounds: their chemistry, biology and clinical use. Chichester UK: Wiley; 1989. p. pp. 60–77.
- Kessel D. Apoptosis, paraptosis and autophagy: death and survival pathways associated with photodynamic therapy. Photochem Photobiol. 2019;95:119–125.
- Kessel D, Evans CL. Promotion of proapoptotic signals by lysosomal photodamage: mechanistic aspects and influence of autophagy. Photochem Photobiol. 2016;92:620–623.
- Donohue E, Tovey A, Vogl AW, et al. Inhibition of autophagosome formation by the benzoporphyrin derivative verteporfin. J Biol Chem. 2011;286:7290–7300.
- Miki Y, Akimoto J, Moritake K, et al. Photodynamic therapy using talaporfin sodium induces concentration-dependent programmed necroptosis in human glioblastoma T98G cells. Lasers Med Sci. 2015;30:1739–1745.
- Saggu S, Hung SI, Quiogue G, et al. Lysosomal signaling enhances mitochondria-mediated photodynamic therapy in A431 cancer cells: role of iron. Photochem Photobiol. 2012;88:461–468.
- Hung HI, Schwartz JM, Maldonado EN, et al. Mitoferrin-2-dependent mitochondrial iron uptake sensitizes human head and neck squamous carcinoma cells to photodynamic therapy. J Biol Chem. 2013;288:677–686.
- Kessel D, Reiners JJ. Enhanced efficacy of photodynamic therapy via a sequential targeting protocol. Photochem Photobiol. 2014;90:889–895.
- Villanueva A, Stockert JC, Cañete M, et al. A new protocol in photodynamic therapy: enhanced tumour cell death by combining two different photosensitizers. Photochem Photobiol Sci. 2010;9:295–297.
- Acedo P, Stockert JC, Cañete M, et al. Two combined photosensitizers: a goal for more effective photodynamic therapy of cancer. Cell Death Dis. 2014;5:e1122.
- Martins WK, ÉT C, Cruz MC, et al. Parallel damage in mitochondrial and lysosomal compartments promotes efficient cell death with autophagy: the case of the pentacyclic triterpenoids. Sci Rep. 2015;5:12425.
- Martins WK, Santos NF, Rocha CS, et al. Parallel damage in mitochondria and lysosomes is an efficient way to photoinduce cell death. Autophagy. 2019;15:259–279.
- Martins WK, Severino D, Souza C, et al. Rapid screening of potential autophagic inductor agents using mammalian cell lines. Biotechnol J. 2013;8:730–737.
- Klionsky DJ, Abdelmohsen K, Abe A, et al. Guidelines for the use and interpretation of assays for monitoring autophagy (3nd edition). Autophagy. 2016;12:1–222.
- Cummings BS, Wills LP, Schnellmann RG. Measurement of cell death in mammalian cells. Curr Protoc Pharmacol. 2004;12. DOI:10.1002/0471141755.ph1208s25.
- Kessel D. Subcellular targeting as a determinant of the efficacy of photodynamic therapy. Photochem Photobiol. 2017;93:609–612.
- Ma T, Li J, Xu Y, et al. Atg5-independent autophagy regulates mitochondrial clearance and is essential for iPSC reprogramming. Nat Cell Biol. 2015;17:1379–1387.
- Rizvi I, Nath S, Obaid G, et al. A combination of Visudyne and a lipid-anchored liposomal formulation of benzoporphyrin derivative enhances photodynamic therapy efficacy in a 3D model for ovarian cancer. Photochem Photobiol. 2019;95:419–429.
- Rizvi I, Obaid G, Bano S, et al. Photodynamic therapy: promoting in vitro efficacy of photodynamic therapy by liposomal formulations of a photosensitizing agent. Lasers Surg Med. 2018;50(5):499–505.
- Sperandio S, Poksay KS, Schilling B, et al. Identification of new modulators and protein alterations in non-apoptotic programmed cell death. J Cell Biochem. 2010;111:1401–1412.
- Sperandio S, Poksay K, de Belle I, et al. Paraptosis: mediation by MAP kinases and inhibition by AIP-1/Alix. Cell Death Differ. 2004;1:1066–1075.
- Kessel D, Cho WJ, Rakowski J, et al. Effects of HPV status on responsiveness to ionizing radiation vs photodynamic therapy in head and neck cancer cell lines. Photochem Photobiol. 2020;96(3):652–657.
- Kessel D Paraptosis and photodynamic therapy: a progress report. Photochem Photobiol. 2020. in press. DOI:10.1111/php.13242.