ABSTRACT
There is increasing evidence that mitophagy, a specialized form of autophagy to degrade and clear long-lived or damaged mitochondria, is impaired in aging and age-related disease. Previous study has demonstrated the obesity-exposed oocytes accumulate and transmit damaged mitochondria due to an inability to activate mitophagy. However, it remains unknown whether mitophagy functions in oocyte and what’s the regulatory mechanism in oocyte aging. In the study, when fully grown oocytes were treated with CCCP, an uncoupling agent to induce mitophagy, we found the activation of the PRKN-mediated mitophagy pathway accompanied the blockage of meiosis at metaphase I stage. Our result then demonstrated its association with the decreased activity of RAB7 and all the observed defects in CCCP treated oocytes could be effectively rescued by microinjection of mRNA encoding active RAB7Q67L or treatment with the RAB7 activator ML098. Further study indicated PRKN protein level as a rate-limiting factor to facilitate degradation of RAB7 and its GEF (guanine nucleotide exchange factor) complex CCZ1-MON1 through the ubiquitin-proteasome system. In GV oocytes collected during ovarian aging, we found the age-related increase of PINK1 and PRKN proteins and a significant decrease of RAB7 which resulted in defects of mitophagosome formation and the accumulation of damaged mitochondria. The age-related retardation of female fertility was improved after in vivo treatment of ML098. Thus, RAB7 activity is required to maintain the balance between mitophagy and chromosome stability and RAB7 activator is a good candidate to ameliorate age-related deterioration of oocyte quality.
Abbreviations: ATG9: autophagy related 9A; ATP: adenosine triphosphate; CALCOCO2/NDP52: calcium binding and coiled-coil domain 2; CCCP: carbonyl cyanide 3-chlorophenylhydrazone; CCZ1: CCZ1 vacuolar protein trafficking and biogenesis associated; GAPDH: glyceraldehyde-3-phosphate dehydrogenase; GAPs: GTPase-activating proteins; GEF: guanine nucleotide exchange factor; GV: germinal vesicle; GVBD: germinal vesicle breakdown; LAMP1: lysosomal-associated membrane protein 1; MI: metaphase I stage of meiosis; MII: metaphase II stage of meiosis; Mito: MitoTracker; mtDNA: mitochondrial DNA; MON1: MON1 homolog, secretory trafficking associated; OPTN: optineurin; PINK1: PTEN induced putative kinase 1; PRKN: parkin RBR E3 ubiquitin protein ligase; RAB7: RAB7, member RAS oncogene family; ROS: reactive oxygen species; TEM: transmission electron microscopy; TOMM20/TOM20: translocase of outer mitochondrial membrane 20; TUBB: tubulin, beta; UB: ubiquitin.
Introduction
In recent years, the incidence of aging-related female infertility has increased, especially in industrialized countries, due to the postponement of marriage and childbearing in women of reproductive age [Citation1]. Although female fertility peaks at approximately 25 years of age, the decrease in female fecundity starts as early as 30 years of age [Citation2]. The parallel decline in both the quantity and quality of oocytes has been shown to contribute to the gradual age-related decline in fertility [Citation3,Citation4]. In aged females, it is believed that the decrease in oocyte quality is related to the increase in chromosomal aberrations and the deterioration of the cytoplasmic quality in oocytes [Citation4]. Approximately 50–70% of mature oocytes from a 40-year-old woman were found to be affected by chromosomal abnormalities [Citation5]. The aneuploidy observed in aged oocytes was implicated in a lower fertility rate, poor embryonic development, and birth defects [Citation6,Citation7]. In addition, cellular organelle dysfunctions, especially abnormalities in mitochondrial function, are also among the most frequently cited causes of the deterioration of aged oocyte quality [Citation8].
Mitochondria are multifunctional organelles that play dominant roles in a series of cellular processes, such as energy production, the apoptosis cascade and calcium balance [Citation9]. The oocyte has notably more mitochondria and mitochondrial DNA (mtDNA) copies than any other cell type in the body (approximately 105–106 copies in a mature oocyte) [Citation10]. This dramatic increase in mitochondrial mass mainly occurs at the later stage of oocyte development, which is consistent with the high bioenergetic demands of the processes of meiosis completion, fertilization and early embryonic development. Mitochondria are the major determinants of oocyte quality, and mitochondrial dysfunction is indeed correlated with ovarian aging. The dysfunction in one or more aspects of mitochondrial biology, for example, decreased intracellular ATP content, decreased mtDNA numbers and accumulated mtDNA point mutants, or altered membrane potential, have been extensively reported to contribute to the deterioration of oocyte quality [Citation8]. Mitochondrial function is also closely associated with the maintenance of genomic stability in oocytes. In various species, an increase in reactive oxygen species (ROS) caused or was caused by mitochondrial dysfunction, and ATP production failure has been shown to induce chromosomal nondisjunction, spindle instability, telomere shortening, and reduced developmental competence of aged oocytes [Citation11]. Moreover, the importance of the mitochondrial threshold in oocyte quality control has also been reflected in aged animals after mitochondrial replacement therapy, antioxidant treatment or calorie restriction [Citation12,Citation13].
Mitochondria are highly dynamic organelles that continuously move, fuse and divide in response to variations in cellular energy demands. Mitochondrial quality is controlled by mitophagy, which detects and repairs damaged mitochondria through selective autophagy to maintain cell activity and function. Altered mitophagy has been increasingly linked to aging and age-predisposing disorders. Many studies have described the involvement of mitophagy defects in metabolic disease, neurodegeneration and cardiac aging [Citation14,Citation15]. Whether mitophagy functions in oocyte or oocyte aging, similar to somatic tissue, remains an interesting area to explore. A previous study showed that obesity-exposed oocytes do not activate mitophagy in response to mitochondrial damage, which results in vast numbers of poised mitochondria inherited by offspring [Citation16]. However, in postovulatory aged oocytes, coenzyme Q10 and resveratrol showed delays in oocyte aging by activating mitophagy [Citation17,Citation18]. If oocytes maintain inactive mitophagy during oocyte maturation, what is the role of mitophagy in oocyte aging, and what is the underlying molecular mechanism?
The most well-studied pathway of mitophagy involves the stabilization of the mitochondrial kinase PINK1 (PTEN induced putative kinase 1) on depolarized mitochondrial membranes. In higher eukaryotes, including humans and insects, PINK1 senses and signals damage to cytosolic E3 ligase PRKN (parkin RBR E3 ubiquitin protein ligase). By avoiding mitochondrial import, PINK1 accumulates on impaired mitochondria with the kinase domain facing the cytosol, where it phosphorylates ubiquitin attached to mitochondrial outer membrane proteins. These phospho-ubiquitin chains recruit PRKN to mitochondria and activate its latent E3 ubiquitin ligase activity. PRKN further ubiquitinates mitochondrial outer membrane proteins and then recruits receptors such as OPTN (optineurin) or CALCOCO2/NDP52 (calcium binding and coiled-coil domain 2) to complete autophagosome assembly proximal to a damaged individual mitochondrion [Citation19,Citation20]. The small GTPase RAB7 (RAB7, member RAS oncogene family) belongs to the RAB family and is known as a key regulator of the late endosomal/lysosomal network. The regulation of RAB7 GDP/GTP binding status has been shown to play roles in diverse functions, such as lysosomal biogenesis, late endosome-lysosome fusion and autophagosome maturation [Citation21]. Recent findings suggest regulatory effects of RAB7 on PINK1-PRKN-mediated mitophagy [Citation22]. RAB7 can be targeted to damaged mitochondria upon mitophagy induction and this recruitment depends on an intact RAB cycling process mediated by the RAB7 guanine nucleotide exchange factor (GEF), MON1 (MON1 homolog, secretory trafficking associated)-CCZ1 (CCZ1 vacuolar protein trafficking and biogenesis associated) complex and GTPase-activating proteins (GAPs). Control of RAB7’s GDP-GTP exchange, as well as its subsequent GTP hydrolysis is crucial for the efficient initiation of mitophagy. In addition, RAB7 also functions in autophagosome formation by correctly targeting ATG9A-bearing vesicles to the area near damaged mitochondria [Citation23]. RAB5 and RAB7 are two key regulators of endosomal trafficking, and their sequential activation of endocytosis has been demonstrated in Xenopus oocytes [Citation24]. In mammals, the function of RAB5 in meiosis has been addressed by controlling proper spindle length and kinetochore-microtubule attachment in meiotic oocytes, and RAB7 participates in meiotic spindle migration in mouse oocytes [Citation25,Citation26]. If RAB7 plays an important role in oocyte meiosis, what is its role in the regulation of mitophagy during oocyte maturation and, furthermore, in oocyte aging?
In this study, when mitochondrial damage was induced in oocytes by CCCP (carbonyl cyanide-m-chlorophenylhydrazone) treatment, the PRKN-mediated mitophagy pathway was activated, and meiotic progression was blocked at the metaphase I (MI) stage. Further study revealed that the cause of these outcomes was related to the decreased activity of RAB7. In oocytes harvested from aged mice, we found age-related accumulations of PINK1 and PRKN proteins accompanied with a significant decreased expression of RAB7. Our results also demonstrated the rescuing effect on CCCP-treated or aged oocytes after increasing the RAB7 activity by microinjection of GTP-bound Rab7Q67L or by the treatment of the RAB7 activator ML098.
Results
Inactive PRKN-mediated mitophagy pathway during oocyte meiosis
To determine whether the mitophagy pathway functions in oocytes, we microinjected mitochondrial-targeted form of Keima (mt-Keima) into GV (germinal vesicle) oocytes to assess mitophagic flux during oocyte maturation. The pH-dependent fluorescent properties of Keima allow rapid determination as to whether the protein is in the mitochondria (pH ~ 8.0, green signals) or the lysosome (pH ~ 4.5, red signals) [Citation27]. As shown in , the overlapped mt-Keima signals in cytoplasm demonstrated the active mitophagic flux in GV oocytes. Following the oocyte entering into meiosis, the overlapped signals were found to be around the chromosomes of pro-MI oocytes; however, in MI and mature metaphase II (MII) oocytes, the lysosome signals of mt-Keima (red) were hardly detected. We then determined the expression patternsof the mitophagy-related protein PRKN during oocyte maturation. Immunostaining showed the uniform distribution of PRKN in the cytoplasm of immature GV oocytes, and following meiosis, the cytoplasmic expression of PRKN decreased gradually, whereas weak signals were detected in the nuclei of Pro-MI oocytes and the spindles of MI and MII oocytes (). Because of the critical role of RAB7 in PRKN-dependent mitophagosome formation during mitochondrial damage, we also checked its distribution in meiotic oocytes. As a marker of late endosomes, RAB7 was identified as a dot or RAB7-positive vesicle and was scattered in the cytoplasm of oocytes. After oocytes enter into meiosis, RAB7 vesicles gradually increased and were mainly located in the cortex and around the nucleus or spindle of Pro-MI and MI oocytes. The largest number of RAB7 vesicles was observed in MII oocytes, with an increased density of dots in the whole cell (). Western blot analysis further demonstrated the opposite expression patterns of PRKN and RAB7 and showed a slight increase in another mitophagy-related protein, PINK1, in meiotic oocytes (). From the mt-Keima labeling and the dynamic expression of mitophagy related proteins, the results imply the inactive mitophagy during oocyte meiosis.
Figure 1. Evaluation of mitophagy activity in oocytes during maturation. P23 female mice were injected with PMSG for 48 h and fully grown oocytes (GV stage) were collected for IVM. Oocytes were harvested at different meiotic stages: 0 h (GV), 3 h (GVBD), 8 h (Pro-MI or MI) and 12 h (MII). (A) Mitophagic flux reflected by mt-Keima labeling. GV oocytes were microinjected with mt-Keima mRNAs and hold in 2 μM milrinone for 8–10 h. Images of oocytes at different meiotic stages were collected at fluorescent emission 550 nm (red) and 440 nm (green), respectively. Red means the active mitophagic flux as the fusion of mitophagosome with lysosome. (B) Immunofluorescence of PRKN in oocytes at each meiotic stage. TUBB was used to label meiotic spindle formation and DNA was stained with Hoechst 33342. Red, PRKN; Green, TUBB; Pink, DNA. (C) Immunofluorescence of RAB7 in oocytes at each meiotic stage. Red, RAB7. Bars: 20 μm. (D) Western blots of RAB7, PRKN and PINK1 proteins in oocytes during meiotic progression. The expression of TUBB was used as internal control. (E) Relative intensity of RAB7, PINK1 and PRKN proteins as compared with TUBB. *, P < 0.05.
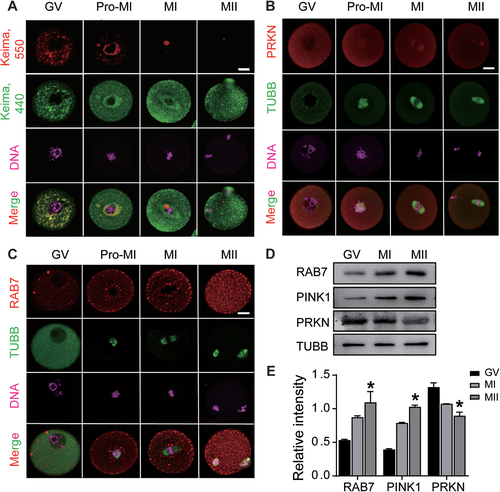
Mitophagy induced by CCCP affects oocyte meiosis
Considering the critical role of mitochondrial functions in meiosis, we next evaluated whether the abnormal activation of mitophagy affects meiotic progression. GV oocytes from young mice (3–4 W) were first treated with the mitochondrial damage inducer CCCP to determine its effects on meiosis. We counted GVBD (germinal vesicle breakdown) rate and first polar body (PB) extrusion rate after 3 h and 12 h of in vitro culture. Compared with GVBD in the control oocytes, the rate of which was greater than 80%, GVBD was blocked in the CCCP-treated oocytes after 3 h of culture. Long-term CCCP treatment showed cytotoxicity, as oocytes failed to extrude the first polar body, and most degenerated after 12 h of incubation (). We then microinjected mCherry-labeled Prkn mRNA into GV oocytes and followed with CCCP treatment for different duration to monitor mitophagy dynamics. Oocytes were collected at 0.5 h, 1 h and 3 h later for MitoTracker staining. As shown in , in control oocytes without CCCP treatment, PRKN proteins were distributed evenly in the cytoplasm without any overlap with mitochondria. CCCP treatment induced juxtanuclear mitochondrial aggregation, and PRKN was found to gradually translocate onto mitochondria after 0.5 h of incubation. We next evaluated the morphology of the mitochondria in CCCP-treated oocytes by TEM (transmission electron microscopy) analysis. CCCP-treated 293 T cells were harvested in parallel for comparison with treated oocytes. Compared with the 293 T cells, the oocytes showed a very quick response to mitochondrial damage, exhibiting ruptured membranes, cristae disappearance and vacuolization shortly after 0.5 h of CCCP treatment, whereas in 293 T cells such mitochondria damage was obvious after 3 h of CCCP treatment (). Mitochondria damage revealed by vacuolated mitochondria counting revealed difference between the oocytes and 293 T cells (the vacuolation rates were 83.67% ±1.52% in oocytes vs. 55%±1.73% in 293 T cells after 1 h of CCCP treatment) (). Western blots further demonstrated a significant increase in PRKN protein levels in the oocytes after CCCP treatment for 1 h, accompanied by dramatically decreased RAB7 expression (). The protein levels of another endosome-related protein, RAB5, however, remained unchanged during CCCP treatment (). The essential role of RAB7 in meiotic maturation has recently been reported through its regulation of actin dynamics and mitochondrial migration [Citation26]. Herein, the significant decrease in RAB7 after the activation of mitophagy may be related to the blockage of oocyte meiosis after CCCP treatment.
Figure 2. Block of meiosis in CCCP treated oocytes. GV oocytes were collected and treated with or without 1 µM CCCP to follow the meiotic progression. (A) Decreased GVBD and first polar body extrusion (PB) rates after CCCP treatment. The GVBD rate was monitored in the first 3 hours and the PB rate was calculated from 8 h-12 h after CCCP treatment. n = 60 oocytes in each group. (B) Activation of mitophagy after CCCP treatment. 10 pl of 500 ng/ml mCherry-Prkn mRNAs were microinjected into GV oocytes and oocytes were hold in 2 μM milrinone for 2 h before treated with CCCP. Oocytes were collected at 0 h, 0.5 h, 1 h and 3 h for MitoTracker staining. Hoechst 33342 was used to label DNA. Red, PRKN; Green, mitochondria; Blue, Hoechst 33342. Bar: 20 µm. (C) CCCP induced mitochondria damage in oocytes and 293 T cell by TEM. Oocytes were collected at 0 h, 0.5 h and 1 h and 293 T cells were harvested at 0.5 h, 1 h and 3 h after treatment. Some oocytes treated with CCCP and RAB7 activator ML098 (1 µM) for 0.5 h were also collected for TEM analysis. Insets, representative good or damaged mitochondria. White arrows, mitochondria with good morphology; black arrows, vacuolated mitochondria. Bars: 500 nm. (D) Accumulation of mitochondria damage in oocytes or 293 T cells. Under TEM images, percentages of damaged mitochondria per area (500 nmX500 nm) were shown. At least three visions were chosen and mitochondria were counted by two individuals. (E) Western blots of RAB5, RAB7, PRKN and PINK1 in oocytes after CCCP treatment. (F) Relative intensity of proteins detected with western blots. TUBB was used as internal control. *, P < 0.05; **, P < 0.01 as compared with controls.
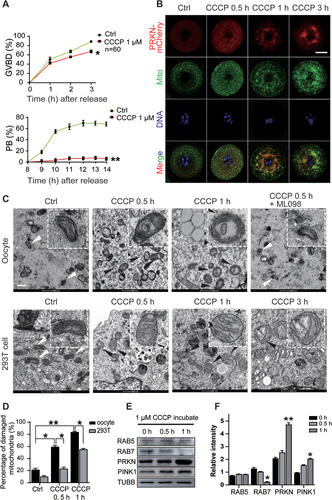
Depletion of RAB7 affects meiosis progression and mitophagy in oocytes
As the only lysosomal RAB protein in the RAB GTPase superfamily, RAB7 regulates transport from late endosomes to lysosomes and plays unique roles in autophagy, especially in the promotion of autophagosome-lysosome fusion. Recent studies on PRKN-mediated mitophagy revealed the necessity of RAB7 for the clearance of damaged mitochondria by regulating mitophagosome formation [Citation28]. To determine whether the downregulated expression of RAB7 is related to the accumulation of damaged mitochondria in oocytes, we depleted the expression of RAB7 in GV oocytes by Rab7 siRNA (siRab7) microinjections and evaluated their efficiencies on oocyte maturation. Accordingly, siRab7-2 (siRab7) was chosen for the following experiments and the specificity of siRab7 treatment was manifested by microinjection of Rab7 mRNA together (). A significant decrease in RAB7 protein levels was manifested in the siRab7 injected oocytes (), which resulted in dramatically decreased GVBD (siCtrl, 92%±1.2% vs. siRab7, 76.5%±2.1%) and PB extrusion rates after in vitro maturation (siCtrl, 69.4%±0.89% vs. siRab7, 16.4%±0.08%) (). MI oocytes injected with control siRNA or siRab7 were then collected for TUBB staining to evaluate spindle morphology, and chromosomes were labeled with Hoechst 33,342. Compared with the siCtrl-injected oocytes, RAB7 depletion led to a shortened spindle with decreased spindle length (siCtrl, 17.88 μM±1.02 μM vs. siRab7, 15.71 μM±1.14 μM) and increased spindle width (siCtrl, 12.14 μM±0.55 μM vs. siRab7, 12.9 μM±0.41 μM) and the ratio of spindle length to width decreased from 1.42 ± 0.06 μM (siCtrl) to 1.19 ± 0.03 μM (siRab7). Moreover, RAB7 depletion induced chromosome displacement with an increased ratio of chromosome displacement to spindle length (siCtrl, 0.33 ± 0.06 μM vs. siRab7, 0.57 ± 0.09 μM) (). Chromosomal spread of MII oocytes revealed increased aneuploidy rates in siRAB7-treated oocytes (siCtrl, 6.3%±0.89% vs. siRab7, 38.9%±4.32%) (). Similar to previous reports, the results showed defects in spindle assembly after RAB7 was knocked down in GV oocytes.
Figure 3. RAB7 knockdown leads to oocyte maturation disorder and abnormal mitophagy. GV oocytes were microinjected with 10 pl Rab7 siRNA (siRab7-1 and siRab7-2) or control siRNA (siCtrl) (500 ng/µl) and arrested at the GV stage for 24 h in M16 medium containing 2 μM milrinone. (A) Decreased GVBD and PB rates after Rab7 siRNA treatment. The GVBD rate was monitored in the first 3 hours and the PB rate was counted from 8 h-12 h after releasing from milrinone. n = 150 oocytes in each group. (B) Abnormal spindle morphology in oocytes after siRab7 treatment. Oocytes in control and treated group were collected at MI stage for immunofluorescence with TUBB antibody. Green, TUBB; Blue, DNA labeled with Hoechst 33342. The length and width of each spindle and the width of chromosome were measured (µm) and then they were shown as the ratio of length to width (length:width) and ratio of chromosome disperse to length (chromosome width:length). n = 20 oocytes in each group. Bar: 10 μm. (C) Chromosome spread in siCtrl and siRab7 treated oocytes. Chromosome was labeled with Hoechst 33342 (blue) and centrosome was labeled with SS18L1/CREST staining (red). Chromosome was counted with two individuals and aneuploidy rates were evaluated. n = 20 oocytes in each group. Bar: 10 μm. (D) Co-staining of ATG9 and LAMP1 in oocytes of siCtrl and siRab7 treated groups by immunofluorescence. Red, ATG9; Green, LAMP1; Pink, DNA labeled with Hoechst 33342. The association of ATG9-positive vesicles with LAMP1 labeled lysosomes was evaluated in control and treated oocytes. n = 20 oocytes in each group. Bar: 20 μm. (E) Immunofluorescence of PRKN in siCtrl and siRab7 treated oocytes. Double staining with TOMM20, the mitochondria outer membrane protein was performed to reveal the translocation of PRKN proteins on mitochondria in siRab7 treated oocytes. Red, PRKN; Green, TOMM20; Pink, DNA was labeled with Hoechst 33342. Oocytes with PRKN translocations were compared between control and treated group. n = 20 oocytes in each group. Bar: 20 μm. (F) The blockage of mitophagic flux after siRab7 treatment. mt-Keima mRNAs were microinjected with siCtrl or siRab7. Images of oocytes were collected at fluorescent emission 550 nm (red) and 440 nm (green), respectively. The ratio (550;440 per oocyte) of fluorescent density was used as an index of mitophagic activity. n = 15 oocytes in each group. Bar: 20 μm. (G) Western Blots of RAB7, LAMP1, PRKN and PINK1 in siCtrl and siRab7 treated groups. The expression of TUBB was used as internal control. The relative intensity of each protein was shown as compared to the expression of TUBB. *, P < 0.05; **, P < 0.01 compared to the control group.
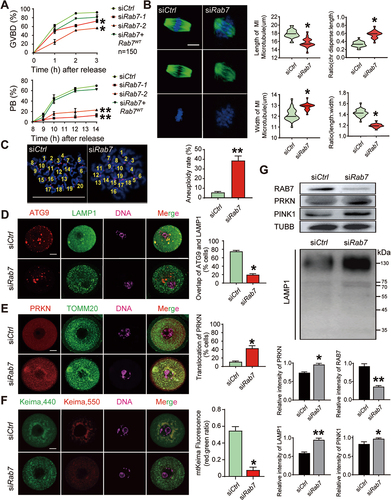
We next checked autophagosome/mitophagosome formation in control and RAB7-depleted oocytes by double-staining ATG9 and LAMP1, a lysosome marker. Among all the essential autophagy proteins, ATG9 is the only known membrane-spanning protein, and it is important for autophagosome/mitophagosome formation as regulated by RAB7 [Citation23,Citation28]. In the control siRNA-treated oocytes, ATG9 was apparent in several stained spherical vesicles scattered in the cytoplasm around nuclei, and LAMP1 was found to be colocalized on ATG9-positive vesicles. However, this colocalization disappeared in RAB7-depleted oocytes, the LAMP1 signals became stronger and appeared as smaller dots scattered throughout the cytoplasm. The dissociation of ATG9 and LAMP1 means defects in membrane fusion during autolysosome biogenesis (). The effects of RAB7 depletion on mitochondria were also examined by double staining PRKN and the mitochondrial protein TOMM20 (Translocase of Outer Mitochondrial Membrane 20). As shown in , PRKN showed basal expression in the cytoplasm of control oocytes, and no overlap was observed with TOMM20-labeled mitochondria. RAB7 knockdown resulted in the aggregation of mitochondria around the nucleus, and PRKN was found to translocate to mitochondria as the overlap of the two staining signals (siCtrl, 11.2% ±0.81% vs. siRab7, 41.7% ±8.52%). Mt-Keima labeling also revealed the blockage of the mitophagic flux as shown by the significant decreased lysosome-Keima in siRab7 treated oocytes (). The increased expression of PRKN and LAMP1 in RAB7-depleted oocytes was also demonstrated by western blots (). Thus, RAB7 depletion not only can inhibit the meiotic progression but can also completely block autophagic flux, leading to the accumulation of damaged mitochondria in oocytes.
RAB7 activity is required to balance mitophagy and meiosis during oocyte maturation
Next, to verify the regulatory effect of RAB7 on CCCP-induced mitochondrial damage and defects in meiosis, Rab7 mRNA (Rab7WT), its dominant-active mutant or its dominant-negative mutant Rab7T22N were injected into CCCP-treated oocytes. RAB7Q67L and RAB7T22N represent the GTP- and GDP-binding status of RAB7, respectively. To our surprise, in all three groups injected with Rab7 variants, only active Rab7Q67L showed rescue effects on the meiotic defects induced by CCCP treatment. showed comparable percentages of GVBD (ctrl, 76.3% ±2.01% vs. CCCP, 41.6% ±16.07% vs. CCCP+RAB7T22N, 31.6% ±5.8% vs. CCCP+Rab7Q67L, 61.1% ±6.55% vs. CCCP+Rab7WT, 24.6% ±8.9%) and PB rates in Rab7Q67L-treated oocytes and control oocytes (ctrl, 64.3% ±3.02% vs. CCCP, 5.5% ±0.09% vs. CCCP+Rab7T22N, 0 vs. CCCP+Rab7Q67L, 45.0% ±1.0% vs. CCCP+Rab7WT, 0). The effects of active RAB7 on CCCP-induced mitochondrial damage were then evaluated by PRKN labeling (mCherry-Prkn mRNA microinjection) and MitoTracker staining. The staining resulted showed the dissociation of PRKN from mitochondria and the decreased density of mitochondria in Rab7Q67L CCCP-treated oocytes (). ATG9 and LAMP1 co-staining also demonstrated the formation of mitophagosome () and western blots revealed significant decreases in PRKN and LAMP1 protein levels ( and Figure S1A) after Rab7 microinjection of mRNA encoding RAB7Q67L. To further delineate the necessity of RAB7 activity in regulating meiosis and mitophagy, the RAB7 activator ML098 was applied with CCCP to determine its effect on oocyte meiosis. As shown in , compared with the complete degeneration of CCCP-treated oocytes, significant increases in oocyte survival (degeneration rates: ctrl, 0 vs. CCCP, 72.3% ±17.5% vs. 0.1 µM ML098, 20.76% ±14.4% vs. 0.5 µM ML098, 21.12% ±9.37% vs. 1 µM ML098, 11.1% ±6.1%) and maturity rate were observed when ML098 was added after CCCP treatment (maturity rates: ctrl, 60.75% ±8.73% vs. CCCP, 0.75% ±0.95% vs. 0.1 µM ML098, 24.5% ±7.3% vs. 0.5 µM ML098, 37% ±6.05% vs. 1.0 µM ML098, 28.75% ±5.79%). The mitochondrial membrane potential was then evaluated by JC-1 staining, and the results verified the recovery of mitochondrial function in the ML098-treated oocytes (). TEM result further demonstrated the rescue effect of ML098 on mitochondria damage induced by CCCP (). Thus, CCCP-induced damage to mitochondria and meiosis can be effectively rescued by increasing the level of active RAB7-GTP in oocytes.
Figure 4. The rescue effect of active RAB7 on CCCP-treated oocytes. GV oocytes were pretreated with 1 µM CCCP for 0.5 h, then wild-type Rab7, GTP-bound active.
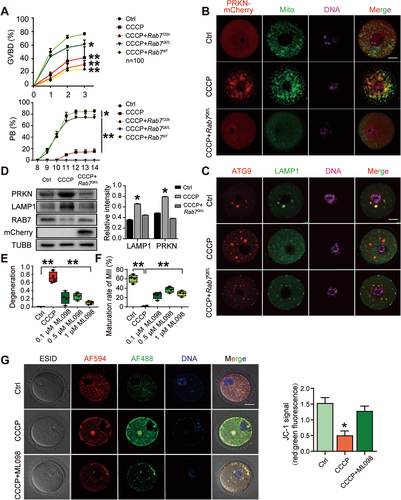
PRKN ubiquitinates RAB7 and RAB7 GEF after being activated by mitochondrial damage
Previous findings indicate that RAB7 can be targeted to damaged mitochondria during mitophagy induction and that recruitment depends on the RAB7 GEF MON1-CCZ1 complex. Similar to other GEFs, the heterodimeric MON1-CCZ1 complex activates RAB7 by catalyzing GDP-GTP exchange, and this process is critical for the efficient initiation of mitophagy [Citation28]. Since PRKN has been reported to regulate the levels and activity of RAB7 by promoting its ubiquitination, considering the negative correlation between RAB7 and the PINK1-PRKN mitophagy pathway in oocytes, we next investigated whether PRKN functions as an E3 ubiquitin ligase in regulating RAB7 degradation [Citation29]. Additionally, because only active RAB7 showed rescue effects on CCCP-induced damage to mitochondria and meiosis in oocytes, we also tested whether the dysregulation of the MON1-CCZ1 complex was induced after CCCP treatment. Western blot analysis demonstrated significant decreases in RAB7, CCZ1 and MON1 protein levels in oocytes after CCCP treatment, whereas the decreased expression of these three proteins was blocked by the addition of MG132, a proteasome inhibitor (). Immunoprecipitation experiments were then performed on RAB7, MON1 and CCZ1 and the results demonstrated a significant increase in ubiquitination of each tested protein after detection with the UB (ubiquitin) antibody (). Since even higher accumulation of ubiquitinated proteins was observed in the MG132-cotreated oocytes, it suggests the involvement of the ubiquitin-proteasome pathway in regulating the degradation of these proteins. We next constructed Rab7-FLAG- and Prkn-HA-expressing vectors and co-transfected them into 293 T cells to check the interactions between PRKN and RAB7. Immunoprecipitation assays with FLAG-tagged and HA-tagged antibodies first verified the interactions between RAB7 and PRKN (Figure S1B). The transfected cells were then treated with or without CCCP for 2 h to examine endogenous MON1 and CCZ1 protein levels. As shown in Figure S1C, the exogenous overexpression of PRKN and RAB7 did not lead to changes in the protein levels of endogenous MON1 or CCZ1. However, short-term (2 h) CCCP treatment induced not only dramatic decrease of exogenous RAB7 but also significant reductions of endogenous MON1 and CCZ1 in a PRKN dose-dependent manner. Similarly, a PRKN dose-dependent increase of total ubiquitin levels was also observed only after CCCP treatment. We then performed in vitro ubiquitination assay to check out whether PRKN mediated the ubiquitination of RAB7, MON1 and CCZ1 by exogenous expression RAB7, MON1 and CCZ1 respectively with ubiquitin and different does of PRKN (). The results again showed only CCCP treatment could induce ubiquitination of exogenous RAB7, MON1 and CCZ1 in a PRKN-does dependent manner. Taken together, these findings indicate the PRKN mediated unbiquitin-proteasome system in the degradation of RAB7 and its GEF MON1-CCZ1, causing a significant decrease in RAB7 activity. This process depends on the activation of CCCP treatment and a significant increase of cellular PRKN levels.
Figure 5. CCCP treatment induced PRKN-dependent ubiquitination of RAB7 and MON1-CCZ1 complex in oocytes and 293 T cells. (A-E) Increased ubiquitination of RAB7, CCZ1 and MON1 after CCCP treatment. GV oocytes (n = 800) were treated with 1 µM CCCP for 0.5 h with or without the co-treatment of the proteasome inhibitor, MG132 10 µM. Input shows the protein levels of PRKN, MON1, CCZ1, RAB7 and UB in each group and TUBB was used as the internal control (A and B). The proteins were then immunoprecipitated with RAB7 (C), MON1 (D) and CCZ1 (E) respectively. Their ubiquitin levels were checked by the UB antibody. (F-K) In vitro ubiquitination assay of RAB7, CCZ1 and MON1 in 293 T cells. Plasmids of GFP-Rab7-FLAG, GFP-Mon1-FLAG, GFP-Ccz1-FLAG (2 µg/105 cells) were transfected into 293 T cells with Ub-MYC (2 µg/105 cells) and different does of mCherry-Prkn-HA (2 µg or 4 µg/105 cells) plasmids, respectively. The 293 T cells were pre-treated with 10 μM MG132 for 6 h. After 48 h of transfection, the transfected 293 T cells were then treated with or without CCCP for 2 h. Western blots of inputs showed the protein levels of exogenous UB, PRKN, RAB7, MON1 and CCZ1 with MYC, HA and FLAG antibodies. CCCP treatment induced significant decrease of RAB7 (F), CCZ1 (G) and MON1 (H) expressions in a PRKN-does dependent manner. Proteins in each group were immunoprecipitated with FLAG antibodies respectively and their ubiquitin levels were checked with MYC antibody. PRKN-dependent ubiquitination of RAB7 (I), CCZ1 (J) and MON1 (K) was only seen after CCCP treatment.
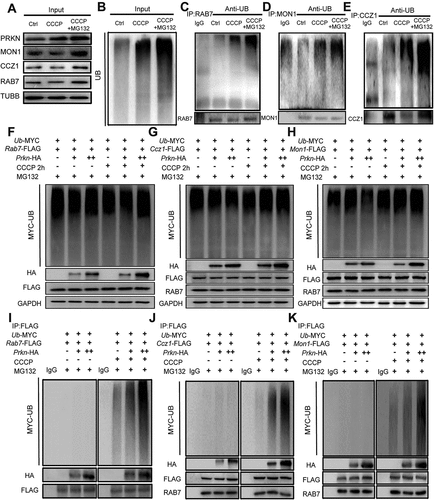
The necessity of RAB7 activity in keeping oocyte quality during ovarian aging
Because of the close connection between mitochondrial dysfunction and oocyte aging, we collected GV oocytes from mice at different ages (3–4 W, 6 M, 8 M and 12 M) to determine the dynamics of mitophagy-related proteins. Western blot analysis showed a significant reduction in RAB7 proteins and a gradual elevation in PRKN and PINK1 expression after 6 months of age (). The co-immunostaining of PRKN and RAB7 in in vitro matured oocytes from young (3–4 W) and old (12 M) mice revealed different expression patterns for the two proteins (). In GV oocytes from aged mice, PRKN aggregates were observed around the nuclei, and because of the high levels of PRKN in the cytoplasm, the locations of PRKN in the meiotic chromosome were not as obvious as those in young oocytes. However, the number of RAB7-positive vesicles decreased significantly in the aged oocytes at all meiotic stages. The results suggest interrelationships of PRKN and RAB7 during oocyte aging. The accumulation of mitochondria damage in oocytes has been widely reported during ovarian aging [Citation30]. By JC-1 staining on GV oocytes from young and aged mice, our result also demonstrated a dramatic reduction of mitochondria membrane potential (ΔΨm) in aged oocytes (Figure S1D). Because RAB7 is activated and translocated to damaged mitochondria during mitophagy, we next injected GTP-bound Rab7Q67L mRNA into young and aged GV oocytes to determine their locations via MitoTracker dye. As shown in , active RAB7 was evenly distributed in the cytoplasm of the young oocytes and was seldom overlapped with mitochondria; however, it was found to form aggregates in the perinuclear area and colocalized with mitochondria in more than 60% of the aged oocytes. The mitophagic flux in young and aged GV oocytes was then evaluated by mt-Keima labeling and the result again indicated the defects of mitophagosome formation in aged oocytes (). Thus, the increased translocation of active RAB7 and PRKN to damaged mitochondria may inhibit the RAB7 mediated mitophagosome formation which results in the blockage of mitophagic flux and the accumulation of damaged mitochondria in aged oocytes.
Figure 6. The activation of PRKN-mediated mitophagy pathway and the blockage of mitophagic flux in aged GV oocytes. GV oocytes were collected from PMSG primed mice at 4 W, 6 M, 8 M and 12 M. (A) Western blots of RAB7, PRKN and PINK1 protein levels in oocytes collected from mice at different ages. The expression of TUBB was used as the internal control. Relative density of each protein was shown as compared with TUBB. (B) Co-staining of PRKN and RAB7 in oocytes at different meiotic stages. Green, RAB7; Red, PRKN; Pink, DNA labeled with Hochest 33342. (C) Increased translocation of active RAB7 on mitochondria in aged oocytes. GV oocytes were collected from 4 W and 12 M mice and microinjected with mCherry-Rab7Q67L mRNA (red) in M2 medium containing 2 μM milrinone for 2 h. Mitochondria was labeled with MitoTracker staining (green) and DNA was counterstained with Hochest 33342 (blue). (D) Oocytes with translocation of active RAB7 on mitochondria were counted and shown as percentage of accumulation. n = 20 oocytes in each group. (E) Decreased mitophagy activity in aged GV oocytes. GV oocytes collected from 4 W and 12 M mice were microinjected with mt-Keima mRNAs and hold in 2 μM milrinone for 8–10 h. Images of oocytes were collected at fluorescent emission 550 nm (red) and 440 nm (green), respectively. (F) The ratio of 550:440 per oocyte was used as an index to reflect mitophagic activity. n = 15 oocytes in each group. (G) ATP levels in oocytes collected from different ages. Increased ATP levels was observed when 12 M oocytes were incubated with 1 µM ML098 for 0.5 h. Bars: 20 µm. *, P < 0.05; **, P < 0.01.
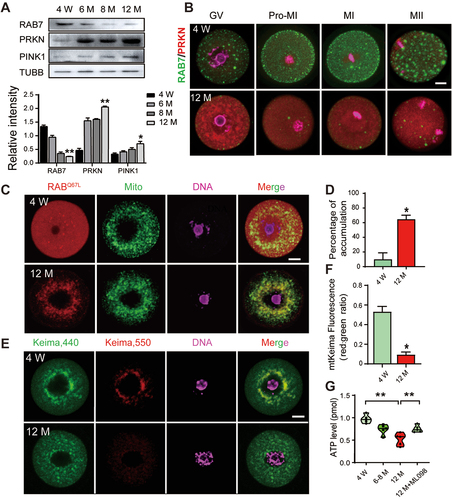
Because of the rescuing effect of the RAB7 activator ML098 on CCCP-induced mitochondrial damage and defects in oocyte meiosis, GV oocytes were harvested from 12 M mice and treated with ML098 for 0.5 h. The decreased ATP levels in aged oocytes appeared first, compared with their appearance in the GV oocytes collected from 4 W or 6–8 M adult mice; however, these ATP levels showed a significant increase after ML098 treatment ().
To evaluate the effects of increased RAB7 activity on oocyte quality, mice at 10 M of age were injected with ML098 every other day for one month. After the protocol was finished, some mice were induced to superovulate, and mature oocytes were collected for IVF, and some mice were mated with fertile adult male mice for the fertility test. ROS staining was first performed to analyze mitochondria functions between the two groups and a dramatic decrease of ROS levels was observed in ML098 treated oocytes (). Oocytes collected from control and ML098 treated mice were then for IVF and increased percentages of 2-cell, 4-cell, morula and blastocyst development were observed in the treated group ( and Figure S1E). After ending the embryonic culture, we harvested blastocysts and performed TUNEL and POU5F1 staining respectively to determine apoptosis and cell numbers between the two groups. The results showed decreased TUNEL positive apoptotic cells and increased total and inner cell mass (ICM, POU5F1-positive cells) cell numbers in blastocysts of ML098 treated group (). Those mice for fertility test also revealed an increase in live births after ML098 treatment (ctrl group, 5.3 ± 0.81 pups/mouse vs. ML098 group, 6.5 ± 1.06 pups/mouse) (). In general, the blockage of mitophagic flux in aged GV oocytes is related with increased translocation of PRKN and active RAB7 on damaged mitochondria; treatment with the RAB7 activator ML098 can ameliorate mitochondrial function and improve oocyte quality in aged mice.
Figure 7. In vivo treatment of ML098 mitigated age-related mitochondria damage and improved oocyte quality. Female mice at 10 M of age were i.p. injected 200 µl RAB7 activator ML098 (1 µM) every other day for one month. Mice with saline injection were served as the control group (Ctrl). (A) ROS staining of MII oocytes collected from control and ML098 treated mice after superovulation. ROS levels were reflected by fluorescence intensity in each group. n = 10 oocytes in each group. (B) Early embryonic development after IVF. Mature oocytes were collected from control (n = 44) and ML098 (n = 52) treated mice after superovulation. Early embryonic development was evaluated at 24 h, 48 h, 72 h and 96 h which represented 2-cell, 4-cell, morula and blastocyst stage respectively. (C) TUNEL and POU5F1 staining in blastocysts collected from control and ML098 treated groups. Left panel, TUNEL staining. Right panel, POU5F1 staining to label inner cell mass of the blastocyst. Blue, DNA labeled with Hochest 33342. (D) TUNEL-positive cells (green) in each blastocyst were counted. (E) Inner cell numbers and total cell numbers were counted in each blastocyst. n = 10 blastocysts in each group. (F) Fertility test after in vivo ML098 treatment. Mice (n = 8) in control and ML098 treated mice were mated with fertile male mice to follow the first delivery of pups. Average pups delivered per female were shown. All bars: 20 µm. *, P < 0.05; **, P < 0.01. (G) A schematic diagram depicting the role of RAB7 in regulating PRKN-mediated mitophagy pathway in oocyte meiosis and oocyte quality control during aging. Left panel showed RAB7 activity is required to maintain mitophagy in an inactive state for the progression of meiosis. RAB7 activity is also essential for the auto/mitophagosome formation in oocytes. Right panel demonstrates the induction of PRKN-mediated mitophagy pathway by the accumulation of mitochondria damage in oocytes during aging. The activation of PRKN-mediated mitophagy pathway in aged oocytes is manifested by increased PRKN protein levels and translocations of PRKN on mitochondria outer membrane. PRKN then recruits RAB7 and its GEF complex MON1-CCZ1 to mitochondria outer membrane and ubiquitinates them for degradation through the ubiquitin-proteasome system. The significant decrease of RAB7 activity further induces the failure of auto/mitophagosome formation and the accumulation of damaged mitochondria in cytoplasm which in turn results in meiotic abnormalities and the deterioration of oocyte quality.
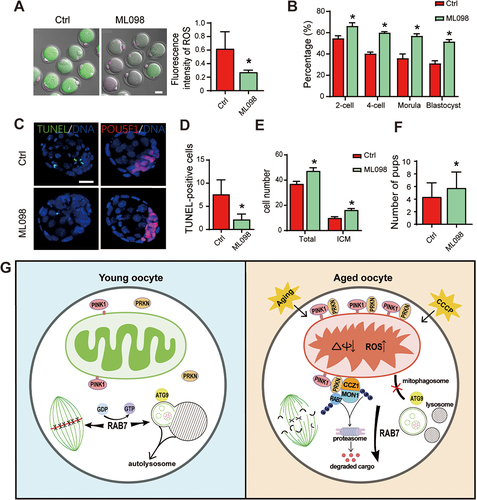
Discussion
Cells can utilize mitophagy, a subset of the macroautophagy pathway, to selectively eliminate damaged mitochondria to maintain cell homeostasis. It has been established that a decline in mitochondrial quality and activity might contribute to normal aging and correlate with a wide range of age-related processes [Citation14,Citation31]. As the unique resource of all the mitochondria for an embryo, oocytes undergo massive increases in mitochondrial biogenesis during follicle development, which results in more mitochondria (105–106) in a mature oocyte than in all types of somatic cells. Since mitochondrial biogenesis reinitiates only at the blastocyst stage, oocyte-containing mitochondria must be maintained within a specific range for bioenergetics in fertilization and early embryonic development [Citation32]. A previous study demonstrated the inability of oocytes to eliminate damaged mitochondria in high-fat high-sugar-exposed female mice2 [Citation16]. This result suggests inactive mitophagy in oocytes. In this study, by mt-Kemia labeling and the dynamic expressions of the mitophagy-related proteins PRKN, PINK1 and RAB7 in oocytes during in vitro maturation, we also observed inactive mitophagy in oocyte meiosis. When CCCP was used to depolarize mitochondria and trigger mitophagy, we found not only the accumulation of damaged mitochondria in the cytoplasm but also the blocked meiosis at the MI stage. Further study revealed that these abnormalities in oocytes were related to a significant decrease in RAB7 activity and were effectively rescued by microinjection of active GTP-bound Rab7Q67L or treatment of the RAB7 activator ML098. According to our results, oocytes do not seem to require mitophagy to clear damaged mitochondria during oocyte maturation or fertilization. Instead, oocytes require the activity of RAB7 in meiotic progression by maintaining mitophagy in an inactive state. This outcome is in accordance with autophagy being unnecessary for oocyte maturation, as shown by oocyte-specific atg5 knockout mice [Citation33]. Moreover, our study demonstrated the decreased mitophagic flux in GV oocytes along with ovarian aging, and in vivo treatment with ML098 ameliorated the deterioration of oocyte quality in old mice. The relationship between chromosomal abnormalities and mitochondrial dysfunctions was established in previous decades [Citation34]. Here, our study revealed that inactive mitophagy in oocytes was the premise for the normal progression of meiosis; however, ovarian aging was accompanied by the failure of mitophagy to eliminate damaged mitochondria in oocytes. In humans, loss-of-function mutations in either PINK1 or PRKN lead to early onset of Parkinson’s disease, and mitophagy rates decrease in the hippocampal dentate gyrus with age and upon human huntingtin overexpression [Citation14,Citation35]. In Drosophila, loss of PRKN expression decreases the fly lifespan, whereas PRKN overexpression fosters fly longevity [Citation36,Citation37]. However, PRKN protein expression was negligibly detected in young hearts and was much higher in aged cardiomyocytes [Citation38,Citation39]. Thus, our data further indicate the tissue- and/or cell-specific regulation of mitophagy and reveal, for the first time, the relationship between mitophagy and chromosomal stability in oocyte meiosis and its dysfunction in oocyte aging.
RAB GTPases are key regulators of membrane trafficking and serve as scaffolds to integrate both membrane trafficking and intracellular signaling in a temporally and spatially sensitive manner [Citation40]. Many RAB family members, including RAB5, RAB6A, RAB11, RAB23, RAB35 and RAB8A, have been reported to function in oocyte meiosis [Citation25,Citation41–45]. RAB7, the key organizer of the late endosomal/lysosomal network, has recently been shown to regulate actin dynamics for DRP1-mediated mitochondrial function and spindle migration in mouse oocyte meiosis [Citation26]. In this study, after activating the PRKN-mediated mitophagy pathway by CCCP treatment, we found meiosis to be blocked at the MI stage, which was accompanied by a significant decrease in the protein levels of RAB7. We reasoned that RAB7 is involved in CCCP-induced meiosis blockade. After RAB7 was knocked down in oocytes, our results also demonstrated defects in meiosis at the MI stage with abnormal spindle morphology and increased aneuploidy. Recent studies suggested a fairly novel idea: RAB7 may have a regulatory role in PINK1-PRKN-mediated mitophagy [Citation22]. In addition to its role in autophagosome-lysosome fusion, RAB7 may drive mitophagosome formation through the transport of ATG9-containing membrane to the forming and expanding phagophore [Citation46]. Actually, the deletion of RAB7 in oocytes increased mitophagy levels by elevating the protein expression of PRKN and PINK1 and the translocation of PRKN to mitochondria. Moreover, the dissociation of ATG9 vesicles from LAMP1-marked lysosomes indicates defects in mito/autophagosome formation. Thus, our study suggests a crucial role of RAB7 in the structure of mito/autophagosomes and its negative regulation of mitophagy in oocytes.
In PINK1-PRKN-mediated mitophagy, RAB7 was targeted to damaged mitochondria, and the process was dependent on RAB7 guanine nucleotide cycling between GDP/GTP-bound states. Similar to that of all the other RAB GTPases, RAB7 activation is promoted by its GEF, MON1-CCZ1 complex, while its inactivation is regulated by enhanced intrinsic GTPase activity through GTPase-activating proteins (GAPs). In valinomycin-induced mitophagy, RAB7 is recruited to damaged mitochondria by the RAB7 GEF MON1-CCZ1 complex [Citation47]. The activity of RAB7 was inhibited by RAB-GAPs, TBC1D15-TBC1D17, and their depletion appeared to result in the excessive proliferation of autophagosomal structures around damaged mitochondria [Citation48]. These results suggest that RAB7 activity is important for mitophagosome formation or biogenesis, not than its destruction. In oocytes, our study revealed a requirement for active RAB7 in not only mitophagosome formation but also in the mitophagy-induced blockade of meiosis. When mRNAs of Rab7, Rab7Q67L and Rab7T22N was microinjected into CCCP-treated oocytes, only the Rab7Q67L rescued all the observed defects. Moreover, the RAB7 activator ML098 also functioned in CCCP-treated oocytes and improved oocyte quality in aged mice after in vivo long-term treatment. An increase in RAB7 availability has been shown to correct mitotic spindle misorientation in cells lacking MYO5B expression [Citation49]. Another RAB GTPase, RAB23, whose GTP form was reported to be essential for spindle organization, drives the motor protein Kif17 [Citation42]. Herein, our results suggest a newly discovered mechanism for the detrimental effects of mitochondrial damage on meiotic progression, and it is related to a significant decrease in RAB7 activity in oocytes.
The ubiquitin-proteasome system is a selective proteolytic system in which substrates are recognized and tagged with ubiquitin for processive degradation by the proteasome. As a multifunctional E3 Ub ligase, PRKN has been implicated in regulating the mitophagy process through the polyubiquitination of outer mitochondrial membrane proteins. For instance, mitofusins MFN1 and MFN2 were reported to be ubiquitinated in a PINK1- and PRKN-dependent manner upon the induction of mitophagy [Citation50]. VDAC1 (voltage-dependent anion channel 1) was identified as a target for PRKN-mediated Lys27 polyubiquitylation and mitophagy [Citation51]. Another study identified hundreds of dynamically regulated ubiquitylation sites in dozens of proteins that were specific PRKN-dependent target modifications generated in response to mitochondrial depolarization [Citation52]. Recently, PRKN has been shown to regulate the levels and activity of RAB7 by promoting its ubiquitination in HEK293 cells [Citation29]. In contrast to the opinion stated by the authors regarding the PRKN-mediated ubiquitination as a means of maintaining RAB7 activity, our results demonstrated the degradation of RAB7 through the ubiquitin-proteasome pathway, which was manifested by the accumulation of ubiquitinated RAB7 in CCCP-treated oocytes and by the increased expression of RAB7 when oocytes were co-treated with the proteasome inhibitor MG132. Since the defects observed on meiosis or mitochondria induced by CCCP treatment in our study could not be rescued by wild-type RAB7, other regulatory mechanisms must block the RAB7 switch from the GDP-bound to the GTP-bound state. In CCCP-treated oocytes, we found the ubiquitination of the RAB7 GEF complex proteins CCZ1 and MON1. Thus, our study revealed the role of the PRKN-dependent ubiquitin-proteasome pathway in the mediation of RAB7 activity in oocytes after mitochondrial damage.
To date, most of our knowledge about mitophagy is based on the overexpression of related proteins or the activation of one or more of these pathways as observed upon the use of the uncoupling agents CCCP or FCCP to elicit mitochondrial damage. However, whether endogenous PRKN can induce mitophagy remains debatable. In human primary fibroblasts and induced pluripotent stem-derived neurons, endogenous levels of PRKN were not sufficient to initiate mitophagy upon the loss of mitochondrial membrane potential. However, upon the overexpression of PRKN in human primary fibroblasts, a series of events indicated mitophagy had been initiated [Citation53]. This finding suggests that the levels of PRKN are the rate-limiting factor in this pathway. In this study, overexpressing PRKN and RAB7 in 293 T cells did not lead to the degradation of endogenous CCZ1 and MON1, but CCCP treatment induced significant decrease of RAB7, CCZ1 and MON1 in a PRKN does-dependent manner. In vitro ubiquitination assay further demonstrated the PRKN does-dependent ubiquitination of the three proteins after CCCP treatment. Since the same doses of PRKN plasmids were transfected, an increase in endogenous PRKN expression, which we observed in CCCP-treated oocytes, is one possible explanation. This suggests that RAB7 and its GEF complex are degraded through the ubiquitin-proteasome pathway only when PRKN levels increase beyond a threshold value. In contrast to our observation with 293 T cells, oocytes seemed more sensitive to CCCP-induced mitochondrial damage, which was reflected by the lower dose and shorter duration time needed to exert an effect and the rapid accumulation of damaged mitochondria, as shown by TEM analysis. Thus, oocytes may represent a unique model to explain the accumulation of mitochondrial damage along with aging, which is related to the positive feedback of PRKN on RAB7 activity. In young oocytes with little mitochondrial damage, PRKN remains at a very low level, and RAB7 activity is required to maintain meiotic progression; when mitochondrial damage accumulates in aged oocytes, the significantly increased level of PRKN and its translocation to mitochondria lead to RAB7 recruitment, which is affected by the RAB7 GEF MON1-CCZ1 complex. The overactivation of PRKN ubiquitinates RAB7 and MON1-CCZ1 and promotes their degradation through the ubiquitin-proteasome system. The dramatically decreased RAB7 activity then induces the blockage of meiosis and the accumulation of damaged mitochondria due to the failure of mito/autophagosome formation ().
Female fertility is one of the first body functions to be affected by aging, which starts to decline in women older than their mid-30s [Citation54]. Decreased oocyte quality is widely acknowledged as the main factor culminating in age-related deterioration of reproductive capacity. Altered mitochondrial function, including decreased energy production, accumulation of mtDNA deletions/mutations or reduced oocyte mitochondrial membrane potential, has been implicated in the predisposition to chromosomal nondisjunction during first and second meiotic division and mitotic errors in embryos and leads to reduced quality and developmental potential in aged oocytes and embryos [Citation55]. The primary cause of the observed mitochondrial dysfunction derives from age-related ROS accumulation and subsequent oxidative damage generated by mitochondria themselves during daily biological metabolism [Citation56]. Many antioxidants, such as melatonin and coenzyme Q10, have been reported to have antiaging effects because they regulate mitochondrial functions and ROS levels in old oocytes [Citation57–59]. A study recently revealed that ROS may act as triggers for the induction of PINK1-PRKN-dependent mitophagy [Citation60]. It is possible that age-related ROS accumulation may be the cause of increased mitophagy levels in old eggs. Based on our findings about the central role of RAB7 in balancing mitochondrial functions and chromosomal stability, the regulation of RAB7 activity may represent a good candidate for oocyte rejuvenation. The rescue effects of the RAB7 activator ML098 on CCCP-treated oocytes and the promotional effect on female fertility in aged mice after long-term ML098 treatment further support our hypothesis. Although IVF has been regarded as a revolutionary technique in infertility treatment, it cannot compensate for the natural decline in infertility with aging. Furthermore, external factors, such as visible light, nonideal pH, temperature, or gamete and embryo manipulations, contribute to higher oxidative stress (OS) [Citation61]. Therefore, supplementation of IVF or IVM media with ML098 may be an effective way to increase oocyte maturation or fertilization rates through a decrease in oxidative damage during oocyte manipulation. ML098 may also be a good drug candidate to improve oocyte quality in women of advanced maternal age in the future.
In conclusion, our study revealed inactive mitophagy in oocytes during meiotic progression. External stimuli such as CCCP treatment or the aging process induce mitochondrial damage and activate the PRKN-mediated mitophagy pathway, which results in defects in meiosis and the accumulation of damaged mitochondria in oocytes. Considering the crucial role of RAB7 activity in maintaining the balance between mitophagy and chromosome stability, we propose the RAB7 activator ML098 as a good candidate in IVF clinics to improve oocyte quality for women of advanced maternal age.
Materials and methods
Mice
ICR mice were purchased from the animal core facility of Nanjing medical university. Mice were maintained under a 12/12 h dark-light cycle at 22°C with free access to food and water. Animal experiments were approved by the Animal Care and Use Committee of Nanjing Medical University and were performed in accordance with institutional guidelines. Oocytes for in vitro maturation were collected from 3 week ICR female mice. Female ICR mice at 10-months old were used for RAB7 activator injection and fertility test was done with the same strain of adult male mice (12 weeks old).
Oocyte collection, culture and treatment
Female mice at P23 were injected with 10 IU PMSG (Ningbo second hormone factory, 11 C25) and GV oocytes were collected 48 h later in M16 medium under mineral oil (Sigma-Aldrich, M8410) at 37°C in 5% CO2. After 3 h and 12 h of incubation, the rates of GVBD and polar body extrusion (PB) were evaluated. GV oocytes were first treated with different does of CCCP (1 µM, 3 µM, 5 µM and 10 µM, Med Chem Express, HY-100,941) and oocyte meiosis was followed at different time points (3 h and 12 h). CCCP was then used at 1 µM to treat oocytes for 0.5 h to see the effect of mitochondria damage on the meiotic progression. In other experiments, RAB7 activator ML098 (0.1 μM, 0.5 μM and 1 μM; Med Chem Express, HY-19,800) or proteasome inhibitor 10 μM MG132 (Sigma-Aldrich, M8699) were added together with CCCP for further analysis.
Plasmid construction, mRNA and siRNA synthesis
mCherry-pBSK or pBSK vectors encoding PRKN, RAB7, RAB7Q67L, RAB7T22N, mt-Keima for mRNA synthesis and plasmids (Ub-MYC, GFP-Rab7-FLAG, GFP-Mon1-FLAG, GFP-Ccz1-FLAG and mCherry-Prkn-HA cloned in pcDNA3.1 vectors) for transfection of 293 T cells were synthesized by Tsingke Biological Technology (Beijing, China). The cDNA sequences used to express GTP- or GDP-bound RAB7 (RAB7Q67L and RAB7T22N) and mt-Keima were referenced from previous reports [Citation62,Citation63]. For mRNA synthesis, plasmids were first linearized by NotI. Then mRNAs were created and tailed using HiScribe T7 ARCA mRNA Kit (NEB, E2065S), purified by a RNA Clean &Concentrator-25 Kit (Zymo Research, R1017) and stored at −80°C for future use. Rab7 siRNA were synthesized by RIOBIO (Guangzhou, China) and the sequence of siRNA is as follows: siCtrl#F, 5ʹ-UUCUCCGAACGUGUCACGUTT-3ʹ, 5ʹ-ACGUGACACGUUCGGAGAATT-3ʹ; siRab7-1#F, 5ʹ-GAACACACGUAGGCCUUCATT-3ʹ, 5ʹ-UGAAGGCCUACGUGUGUUCTT-3ʹ; siRab7-2#F, 5ʹ-GGAUGACCUCUAGGAAGAATT-3ʹ, 5ʹ-UUCUUCCUAGAGGUCAUCCTT-3ʹ. The siRNA powder was then diluted with DEPC water to a storage concentration of 20 µM and stored at −80°C for use.
Microinjection
siRNA for knockdown and mRNA for overexpression were injected into oocytes by microinjector (Lecia, Germany). Ten pl of 500 ng/µl mRNA or siRNA were injected into oocytes. The injected oocytes were arrested at the GV stage for 2–10 h in M2 medium (Sigma-Aldrich, M7167) containing 2 μM milrinone (Sigma-Aldrich, 78,415–72-2) after mRNA injection or arrested at the GV stage for 24 h in M16 medium (Sigma-Aldrich, M7292) containing 2 μM milrinone after siRNA injection. Then the oocytes were washed for 5 times and moved to M16 culture medium without milrinone for subsequent experiments.
Immunofluorescence
Oocytes or blastocysts were fixed with 4% paraformaldehyde and 0.5% Triton X-100 (Sigma-Aldrich, X100) in PBS (Sangon Biotech, E607008, pH 7.4) for 30 min at room temperature. After washing three times with PBS, they were moved to PBS with 1% BSA (Sigma-Aldrich, A1933) and incubated at 4°C for 12 h. Primary antibodies were then incubated at 4°C for 4–6 h and followed with secondary antibodies for 1 h at room temperature after three washes with PBS with 1% BSA. Nuclei were counterstained with Hoechst 33,342 (Life Technologies, H3570) at room temperature for 30 min and immunofluorescence signals were observed using a confocal laser scanning microscope (LSM 710; Zeiss, Germany). Primary and secondary antibodies were obtained from the following commercial sources: anti-RAB7A antibody (Cell Signaling Technology, 95,746), anti-PRKN antibody (ZEN BIO, 381,626), anti-ATG9A antibody (ZEN BIO, 381,260), anti-LAMP1 antibody (DSHB, 1D4B), anti-POU5F1/OCT4 antibody (Abcam, ab25630) and anti-TOMM20 antibody (Santa Cruz Biotechnology, sc-17764), anti-TUBB (Cell Signaling Technology, 2146). All secondary antibodies, Donkey anti-Rabbit IgG (Invitrogen, A-21207), Donkey anti-Mouse IgG (Invitrogen, A-21202) and Donkey anti-Rat IgG (Invitrogen, A-11007) were from Invitrogen. For TUNEL staining, blastocysts were labeled with TUNEL Apoptosis Detection Kit (YEASEN, 40307ES60) and the TUNEL-positive cells were counted in each blastocyst by two individuals.
Quantification of mitophagy
After mt-Keima injection, oocytes collected from young mice (4 W) for different treatments and old mice (12 M) were observed under the confocal laser scanning microscope with fluorescence emission at 550 nm and 440 nm, respectively. Images of Keima at 550 nm and 440 nm fluorescence emissions were created and analyzed by using Zeiss ZEN imaging software. The whole-cell region was delineated manually to facilitate area calculation. The ratio (550:440 per oocyte) of fluorescent density was used as an index of mitophagic activity.
Western blots
Oocytes or 293 T cells were lysed with RIPA lysate (Solarbio, R0010) containing protease inhibitor (Solarbio, K106993P) and placed on ice for 5 min. Then protein loading buffer (Beyotime, P0015) was added and denatured at 95°C water for 5–10 min. A total of 20 μg proteins in each sample were loaded and separated by electrophoresis (Bio-Rad, USA). After electronic transfer (Bio-Rad, USA), the PVDF membranes (ThermoFisher, 88,518) were blocked in 20 ml 5% milk for at least 1 h and then incubated overnight at 4°C with specific antibodies. The membranes were then washed three times with TBST (Beyotime, ST677) and incubated with secondary antibodies for 1 h at room temperature. Finally, the protein bands were visualized using an ECL (Beyotime, China) and Western Blotting Detection System (GE Healthcare, USA). Primary and secondary antibodies were obtained from the following commercial sources: anti-RAB5A antibody and anti-MYC antibody (Proteintech, 11,947-1-AP and 16,286-1-AP), anti-mCherry antibody (Abcam, ab213511),anti-GADPH antibody (Abcam, ab8245), anti-FLAG antibody (ZEN BIO, 390,002). Anti-HA antibody, anti-UB antibody, anti-CCZ1 antibody and anti-PINK1 antibody were purchased from Santa Cruz Biotechnology (sc-7392, sc-8017, sc-514,290 and sc-517,353). All secondary antibodies, Goat Anti-mouse IgG H&L (HRP), Goat Anti-Rabbit IgG H&L (HRP), Rabbit Anti-Goat IgG H&L (HRP), Goat Anti-Rat IgG H&L (HRP) were from YIFEIXUE Biotechnology (YFSA02, YFSA01, YFSA03 andYFSA04).
Transmission electron microscopy (TEM)
oocytes and 293 T cells were fixed under different conditions. Oocytes were fixed with 2.5% glutaraldehyde for 2 h and stained with eosin (Bio-Channel, BC-DL-043) for 1 s. Then the oocytes were embedded in 1.5% agarose(BIOFROXX, 1110GR100) and the agarose gel cube (2 × 2 × 2 mm) containing oocytes were fixed overnight in 2.5% glutaraldehyde. 293 T cells were centrifuged at 2500 g for 5 min and fixed with 2.5% glutaraldehyde for 24 h. Then, oocytes and 293 T cells were processed and wrapped in epoxypropane resin following standard TEM procedures. Finally, cells were observed by transmission electron microscopy (Tecnai G2 Spirit BioTwin, FEI, USA).
Chromosome spread
MII oocytes were briefly (30 s) incubated in Tyrode’s buffer (Sigma-Aldrich,T1788,pH 2.5) at 37°C to remove the zona pellucida. Then oocytes were cultured in M2 medium for 10 min and treated with 1% sodium citrate for 60 s. The oocytes were then fixed on a glass slide in a drop of 1% PFA that containing 0.15% TritonX-100 and air-dried at room temperature. The samples were washed three times with PBS with 1% BSA and blocked at room temperature for 1 h. Oocytes were incubated with SS18L1/CREST antibody (Abcam, ab227535) overnight at 4°C and chromosomes were stained with Hoechst 33,342. Chromosomes were examined under a laser scanning confocal microscope.
Cell culture, transfection and treatment
293 T cells were cultured in DMEM media (Sigma-Aldrich, D0819) with 10% FBS (SORFA, SX1111) and cultured at 37.0°C, 5% O2, 5% CO2 in humidified atmosphere. For 293 T cell transfection, plasmids were mixed with lipofectamine 2000 (Invitrogen, 11,668,019) in DMEM medium and incubated at room temperature for 5 min. The ratio of DNA to lipofectamine was calculated according to the producer’s instruction. The mixture was then added into cell culture and incubated at 37°C for 6 h in FBS free DMEM medium. After being changed to the medium containing 10% FBS, cells were further cultured for 48 h and samples were collected for western blots or immunoprecipitations. To induce mitochondrial damage, cells were treated with 10 μM CCCP for 2 h before harvesting.
Immunoprecipitation
800 oocytes or 1 × 106293 T cells were lysed with RIPA lysate containing protease inhibitor and placed on ice for 20 min. Total 3 µl antibodies (0.2 µg/µl) were added to each protein sample and incubated at 4°C in a rotating wheel overnight. Then 30 µl protein-A/G beads (Sigma-Aldrich, LSKMAGAG) were added to each sample and incubated at 4°C for 3–5 h. After centrifugation at 2000 g for 20 s, the supernatants were removed and the beads were washed with PBS twice. The beads were then added with protein loading buffer and denatured at 95°C for 5 min. After a brief centrifugation, the collected supernatants were for western blots with specific antibodies.
Mitochondrial function assessment
We evaluated the mitochondrial function in oocytes through the detection of ATP content, mitochondria distribution by MitoTracker (Yeasen, 40742ES50) staining, and measurement of mitochondrial membrane potential and ROS level. For ATP detection, five oocytes in each group were washed three times in PBS, and then transferred into 50 µl ultra-pure water and stored at −20°C overnight. Oocyte ATP levels were then decided according to the manual of ATP bioluminescent somatic cell assay kit (Sigma-Aldrich, FLASC). A six-point standard curve (0, 1.0, 2.5, 5.0, 7.5, and 10 pmol of ATP) was generated in each assay, and ATP levels were calculated using the formula derived from the linear regression of the standard curve. For MitoTracker, JC-1 and ROS staining, all the reagents MitoTracker, JC-1 (200X, Beyotime, C2006) and CellROX Orange Reagent (Yeasen, 50101ES01) were first diluted with M2 medium to make culture drops and placed in an incubator at 37°C to balance for 20 min. Oocytes were then transferred in the culture drops and incubated at 37°C for 30 min. After counterstaining with Hoechst 33,342, oocytes were moved to a glass bottom culture dish and examined under a laser scanning confocal microscope. Mitochondria distribution was reflected by MitoTracker staining. Mitochondria membrane potential was determined by JC-1 staining. JC-1 exhibited potential-dependent accumulation in mitochondria, indicated by a fluorescence emission shift from green (~529 nm) to red (~590 nm). Consequently, mitochondrial depolarization was indicated by a decrease in the red/greenfluorescence intensity ratio. Oocyte ROS level was reflected by the density of fluorescent signals of ROS staining.
In vitro ubiquitination assay
GFP-Rab7-FLAG, GFP-Mon1-FLAG, GFP-Ccz1-FLAG were transfected into 293 T cells with Ub-MYC and different does of mCherry-Prkn-HA, respectively. 293 T cells were treated with 10 μM MG132 for 6 h in advance. Forty-eight h after transfection, protein extraction was performed with NP-40 lysis buffer and the whole-cell lysates were incubated in rotation with anti-FLAG antibody or rabbit IgG isotype at 4°C overnight. Precipitation of the antibody-bound proteins were performed by adding protein-A/G beads (ThermoFisher, 78,609) and incubated at 4°C for 3–5 h. After a brief centrifuge and washing with washing buffer (50 mM Tris pH 8.5, 1 Mm), the precipitates were for immunoblotting analysis with specific antibodies.
In vivo treatment of RAB7 activator ML098 in old mice
Twenty female mice at 10 M of age were randomly divided into control and treated groups. In the treated group, mice were intraperitoneally injected with 0.2 ml RAB7 activator ML098 (1 μM) every other day, whereas mice injected with the same does of saline were served as controls. One month later, some mice in the two groups were supperovulated with PMSG and hCG and mature oocytes were collected for IVF or oocyte quality evaluation by ROS staining; some mice were mated with fertile male mice for fertility test.
In vitro fertilization (IVF)
Fourteen mice were first injected with 15 IU PMSG and followed with a single injection of 10 IU hCG (Ningbo second hormone factory, 200,929) 48 h later. Expanded cumulus-oocyte complexes were collected from Fallopian tubes after 14–16 h of hCG injection and placed in M2 medium containing 0.3 mg/ml hyaluronidase (Sigma-Aldrich, H3506) to remove cumulus cells. After several washes, some MII oocytes were picked up for ROS staining and others were incubated in 200 µl HTF medium (Sigma-Aldrich, MR-070) with capacitated sperm for in vitro fertilization. Sperm were collected from epididymis of male mice at 8–10 W and incubated in HTF medium under oil for 1 h at 37°C in a 5% CO2 and 95% air for capacitation. After 6 h of incubation, fertilized oocytes were moved to KSOM culture medium (Sigma-Aldrich, MR-106) and incubated at 37°C to follow embryonic development at 24 h, 48 h, 72 h and 96 h, respectively. Embryonic development was evaluated as the ratio of 2-cell/MII, 4-cell/MII, morula/MII and blastocyst/MII. Blastocysts in each group were then fixed in 4% PFA for TUNEL and POU5F1 staining to evaluate apoptosis and cell numbers of each embryo.
Fertility test
Eight old female mice injected with saline and eight old female mice injected with ML098 were mated with 3-month-old male ICR mice. After finishing the ML098 injection protocol, mice from control and treated groups were monitored for estrous cycles and only mice in estrous were chosen to mate with males of proven fertility. The mating was confirmed by the formation of the fertilization plug (within 3–5 days). Females were then separated from males and pregnancy was allowed to progress until delivery. The mating rates and numbers of live pups were determined.
Statistical analysis
Each experiment was repeated at least three times. Student’s t test or one-way ANOVA were used to compare the statistical differences between experimental groups. Image J (NIH, USA) was used to analyze western blot protein bands and confocal images. All data are presented as mean ± SEM. P < 0.05 considered to indicate a significant result.
Supplemental Material
Download Zip (2.8 MB)Acknowledgments
J.L. thanks the supports from the National Key Research and Development Program of China (2018YFC1004203, 2018YFC1003703) and the National Natural Science Foundation of China (31871513, 31671202).
Disclosure statement
No potential conflict of interest was reported by the author(s).
Supplementary material
Supplemental data for this article can be accessed here
Additional information
Funding
References
- Qiao J, Wang ZB, Feng HL, et al. The root of reduced fertility in aged women and possible therapentic options: current status and future perspects. Mol Aspects Med. 2014;38:54–85.
- Broekmans FJ, Soules MR, Fauser BC. Ovarian aging: mechanisms and clinical consequences. Endocr Rev. 2009;30:465–493.
- Nelson SM, Telfer EE, Anderson RA. The ageing ovary and uterus: new biological insights. Hum Reprod Update. 2013;19:67–83.
- Djahanbakhch O, Ezzati M, Zosmer A. Reproductive ageing in women. J Pathol. 2007;211:219–231.
- Yoldemir T. Fertility in midlife women. Climacteric. 2016;19:240–246.
- Herbert M, Kalleas D, Cooney D, et al. Meiosis and maternal aging: insights from aneuploid oocytes and trisomy births. Cold Spring Harb Perspect Biol. 2015;7:a017970.
- Mikwar M, MacFarlane AJ, Marchetti F. Mechanisms of oocyte aneuploidy associated with advanced maternal age. Mutat Res. 2020;785:108320.
- May-Panloup P, Boucret L, Chao de la Barca JM, et al. Ovarian ageing: the role of mitochondria in oocytes and follicles. Hum Reprod Update. 2016;22:725–743.
- O’Sullivan D, Sanin DE, Pearce EJ, et al. Metabolic interventions in the immune response to cancer. Nat Rev Immunol. 2019;19:324–335.
- Shoubridge EA, Wai T. Mitochondrial DNA and the mammalian oocyte. Curr Top Dev Biol. 2007;77:87–111.
- Guo Z, Yu Q. Role of mTOR signaling in female reproduction. Front Endocrinol (Lausanne). 2019;10:692.
- Chiang JL, Shukla P, Pagidas K, et al. Mitochondria in ovarian aging and reproductive longevity. Ageing Res Rev. 2020;63:101168.
- Dou X, Sun Y, Li J, et al. Short-term rapamycin treatment increases ovarian lifespan in young and middle-aged female mice. Aging Cell. 2017;16:825–836.
- Jang JY, Blum A, Liu J, et al. The role of mitochondria in aging. J Clin Invest. 2018;128:3662–3670.
- Srivastava S. The mitochondrial basis of aging and age-related disorders. Genes (Basel). 2017;8:398.
- Boudoures AL, Saben J, Drury A, et al. Obesity-exposed oocytes accumulate and transmit damaged mitochondria due to an inability to activate mitophagy. Dev Biol. 2017;426:126–138.
- Niu YJ, Zhou W, Nie ZW, et al. Ubiquinol-10 delays postovulatory oocyte aging by improving mitochondrial renewal in pigs. Aging (Albany NY). 2020;12:1256–1271.
- Zhou J, Xue Z, He HN, et al. Resveratrol delays postovulatory aging of mouse oocytes through activating mitophagy. Aging (Albany NY). 2019;11:11504–11519.
- Roberts RF, Tang MY, Fon EA, et al. Defending the mitochondria: the pathways of mitophagy and mitochondrial-derived vesicles. Int J Biochem Cell Biol. 2016;79:427–436.
- Ashrafi G, Schwarz TL. The pathways of mitophagy for quality control and clearance of mitochondria. Cell Death Differ. 2013;20:31–42.
- Guerra F, Bucci C. Multiple roles of the small GTPase RAB7. Cells. 2016;5(3):34.
- Tan EHN, Tang BL. RAB7a and mitophagosome formation. Cells. 2019;8(3):224.
- Zheng H, Miao P, Lin X, et al. Small GTPase RAB7-mediated FgAtg9 trafficking is essential for autophagy-dependent development and pathogenicity in Fusarium graminearum. PLoS Genet. 2018;14(7):e1007546.
- Mukhopadhyay A, Barbieri AM, Funato K, et al. Sequential actions of Rab5 and RAB7 regulate endocytosis in the Xenopus oocyte. J Cell Biol. 1997;136:1227–1237.
- Ma R, Hou X, Zhang L, et al. Rab5a is required for spindle length control and kinetochore-microtubule attachment during meiosis in oocytes. FASEB J. 2014;28:4026–4035.
- Pan ZN, Pan MH, Sun MH, et al. RAB7 GTPase regulates actin dynamics for DRP1-mediated mitochondria function and spindle migration in mouse oocyte meiosis. FASEB J. 2020;34:9615–9627.
- Katayama H, Kogure T, Mizushima N, et al. A sensitive and quantitative technique for detecting autophagic events based on lysosomal delivery. Chem Biol. 2011;18:1042–1052.
- Yamano K, Wang C, Sarraf SA, et al. Endosomal Rab cycles regulate Parkin-mediated mitophagy. Elife. 2018;7. DOI:10.7554/eLife.31326
- Song P, Trajkovic K, Tsunemi T, et al. Parkin modulates endosomal organization and function of the endo-lysosomal pathway. J Neurosci. 2016;36:2425–2437.
- Miao Y, Cui Z, Gao Q, et al. Nicotinamide mononucleotide supplementation reverses the declining quality of maternally aged oocytes. Cell Rep. 2020;32:107987.
- Sun N, Youle RJ, Finkel T. The mitochondrial basis of aging. Mol Cell. 2016;61:654–666.
- Cummins JM. The role of mitochondria in the establishment of oocyte functional competence. Eur J Obstet Gynecol Reprod Biol. 2004;115(Suppl 1):S23–9.
- Tsukamoto S, Kuma A, Murakami M, et al. Autophagy is essential for preimplantation development of mouse embryos. Science. 2008;321:117–120.
- Van Blerkom J, Runner MN. Mitochondrial reorganization during resumption of arrested meiosis in the mouse oocyte. Am J Anat. 1984;171:335–355.
- Sun N, Yun J, Liu J, et al. Measuring in vivo mitophagy. Mol Cell. 2015;60:685–696.
- Greene JC, Whitworth AJ, Kuo I, et al. Mitochondrial pathology and apoptotic muscle degeneration in Drosophila parkin mutants. Proc Natl Acad Sci U S A. 2003;100:4078–4083.
- Rana A, Rera M, Walker DW. Parkin overexpression during aging reduces proteotoxicity, alters mitochondrial dynamics, and extends lifespan. Proc Natl Acad Sci U S A. 2013;110:8638–8643.
- Song M, Gong G, Burelle Y, et al. Interdependence of parkin-mediated mitophagy and mitochondrial fission in adult mouse hearts. Circ Res. 2015;117:346–351.
- Thai PN, Seidlmayer LK, Miller C, et al. Mitochondrial quality control in aging and heart failure: influence of ketone bodies and mitofusin-stabilizing peptides. Front Physiol. 2019;10:382.
- Takai Y, Sasaki T, Matozaki T. Small GTP-binding proteins. Physiol Rev. 2001;81:153–208.
- Hou X, Zhang J, Li L, et al. Rab6a is a novel regulator of meiotic apparatus and maturational progression in mouse oocytes. Sci Rep. 2016;6:22209.
- Cheng H, Govindan JA, Greenstein D. Regulated trafficking of the MSP/Eph receptor during oocyte meiotic maturation in C. elegans. Curr Biol. 2008;18:705–714.
- Wang HH, Zhang Y, Tang F, et al. Rab23/Kif17 regulate meiotic progression in oocytes by modulating tubulin acetylation and actin dynamics. Development. 2019;146. DOI:10.1242/dev.171280
- Zhang Y, Wan X, Wang HH, et al. RAB35 depletion affects spindle formation and actin-based spindle migration in mouse oocyte meiosis. Mol Hum Reprod. 2019;25:359–372.
- Pan ZN, Lu Y, Tang F, et al. RAB8A GTPase regulates spindle migration and Golgi apparatus distribution via ROCK-mediated actin assembly in mouse oocyte meiosisdagger. Biol Reprod. 2019;100:711–720.
- Jimenez-Orgaz A, Kvainickas A, Nagele H, et al. Control of RAB7 activity and localization through the retromer-TBC1D5 complex enables RAB7-dependent mitophagy. EMBO J. 2018;37:235–254.
- Vaites LP, Paulo JA, Huttlin EL, et al. Systematic analysis of human cells lacking ATG8 proteins uncovers roles for GABARAPs and the CCZ1/MON1 regulator C18orf8/RMC1 in macroautophagic and selective autophagic flux. Mol Cell Biol. 2018;38. DOI:10.1128/MCB.00392-17
- Yamano K, Fogel AI, Wang C, et al. Mitochondrial Rab GAPs govern autophagosome biogenesis during mitophagy. Elife. 2014;3:e01612.
- Leng C, Overeem AW, Carton-Garcia F, et al. Loss of MYO5B expression deregulates late endosome size which hinders mitotic spindle orientation. PLoS Biol. 2019;17:e3000531.
- Gegg ME, Cooper JM, Chau KY, et al. Mitofusin 1 and mitofusin 2 are ubiquitinated in a PINK1/parkin-dependent manner upon induction of mitophagy. Hum Mol Genet. 2010;19:4861–4870.
- Geisler S, Holmstrom KM, Skujat D, et al. PINK1/Parkin-mediated mitophagy is dependent on VDAC1 and p62/SQSTM1. Nat Cell Biol. 2010;12:119–131.
- Sarraf SA, Raman M, Guarani-Pereira V, et al. Landscape of the PARKIN-dependent ubiquitylome in response to mitochondrial depolarization. Nature. 2013;496:372–376.
- Rakovic A, Shurkewitsch K, Seibler P, et al. Phosphatase and tensin homolog (PTEN)-induced putative kinase 1 (PINK1)-dependent ubiquitination of endogenous Parkin attenuates mitophagy: study in human primary fibroblasts and induced pluripotent stem cell-derived neurons. J Biol Chem. 2013;288:2223–2237.
- Cecchino GN, Seli E, Alves da Motta EL, et al. The role of mitochondrial activity in female fertility and assisted reproductive technologies: overview and current insights. Reprod Biomed Online. 2018;36:686–697.
- Eichenlaub-Ritter U, Wieczorek M, Luke S, et al. Age related changes in mitochondrial function and new approaches to study redox regulation in mammalian oocytes in response to age or maturation conditions. Mitochondrion. 2011;11:783–796.
- Sasaki H, Hamatani T, Kamijo S, et al. Impact of oxidative stress on age-associated decline in oocyte developmental competence. Front Endocrinol (Lausanne). 2019;10:811.
- Zhang L, Zhang Z, Wang J, et al. Melatonin regulates the activities of ovary and delays the fertility decline in female animals via MT1/AMPK pathway. J Pineal Res. 2019;66(3):e12550.
- Ben-Meir A, Burstein E, Borrego-Alvarez A, et al. Coenzyme Q10 restores oocyte mitochondrial function and fertility during reproductive aging. Aging Cell. 2015;14:887–895.
- Tamura H, Kawamoto M, Sato S, et al. Long-term melatonin treatment delays ovarian aging. J Pineal Res. 2017;62(2):e12381.
- Xiao B, Goh JY, Xiao L, et al. Reactive oxygen species trigger Parkin/PINK1 pathway-dependent mitophagy by inducing mitochondrial recruitment of Parkin. J Biol Chem. 2017;292:16697–16708.
- Agarwal A, Durairajanayagam D, Du Plessis SS. Utility of antioxidants during assisted reproductive techniques: an evidence based review. Reprod Biol Endocrinol. 2014;12:112.
- Kawakami A, Sakane F, Imai S, et al. RAB7 regulates maturation of melanosomal matrix protein gp100/Pmel17/Silv. J Invest Dermatol. 2008;128:143–150.
- Kogure T, Karasawa S, Araki T, et al. A fluorescent variant of a protein from the stony coral Montipora facilitates dual-color single-laser fluorescence cross-correlation spectroscopy. Nat Biotechnol. 2006;24:577–581.