ABSTRACT
Clearance of misfolded proteins from the secretory pathway often occurs soon after their biosynthesis by endoplasmic reticulum (ER)-associated protein degradation (ERAD). However, certain types of misfolded proteins are not ERAD substrates and exit the ER. They are then scrutinized by ill-defined post-ER quality control (post-ERQC) mechanisms and are frequently routed to the vacuole/lysosome for degradation. Glycosylphosphatidylinositol-anchored proteins (GPI-APs) constitute a class of proteins of the secretory pathway that mostly depends on post-ERQC. How misfolded GPI-APs are detected, transported to the vacuole/lysosome and taken up by this organelle was poorly defined. Originating from the intriguing observation that several misfolded GPI-APs accumulate in the yeast vacuolar membrane in the absence of the major vacuolar protease Pep4, we designed an unbiased genome-wide screen in yeast and followed the trafficking of the misfolded fluorescent GPI-AP Gas1* from the ER to the vacuolar lumen. Our results reveal that post-ERQC of GPI-APs is linked with a novel type of microautophagy.
Glycosylphosphatidylinositol-anchored proteins (GPI-APs) are abundant membrane-conjugated cell-surface proteins, playing key structural, developmental and signaling roles in eukaryotic cells. Initially, GPI-APs are synthetized with a C-terminal transmembrane domain (TMD) and soon after they have been translocated into the endoplasmic reticulum (ER), their TMD is replaced by a membrane-embedded pre-assembled GPI anchor. As a result, GPI-APs are attached to the lumenal/extracellular leaflet of the membrane bilayer, being deprived of cytosolic tails or TMDs. Following attachment, the GPI anchor undergoes several modifications on both glycan and lipid moieties that are crucial for protein export from the ER and trafficking to its destination, the plasma membrane.
If newly synthesized GPI-APs misfold, the modified (remodeled) GPI anchor often causes overriding both ERQC and retention mechanisms, resulting in their premature ER exit. However, these species are then detected by post-ERQC mechanisms and will eventually be targeted to the vacuole/lysosome for degradation. Thus, misfolded GPI-APs are ideal to study post-ERQC and degradation. Moreover, although membrane-bound, the lack of cytosolic tails and TMDs makes GPI-APs unique substrates because they are invisible to known ubiquitination-dependent processes for “conventional” protein tagging and invagination at endosomes en route to the vacuole/lysosome.
In our recent work [Citation1], we combined fluorescence live-cell imaging with genome-wide screening under physiological conditions to uncover mechanisms linked to post-ERQC and degradation of newly synthesized misfolded GPI-APs. We used the budding yeast Saccharomyces cerevisiae as a model organism in combination with Gas1*, a misfolded mutant version of the yeast GPI-AP Gas1. Wild-type cells expressing GFP-Gas1* reveal most of the fluorescent signal inside the vacuolar lumen, as expected. Intriguingly, when we imaged pep4∆ cells, which have strongly reduced hydrolytic activity inside the vacuole and are often used as control for vacuolar protein degradation, GFP-Gas1* and other misfolded GPI-APs accumulate in the vacuolar membrane.
GFP-Gas1* in the vacuolar membrane could not represent an intermediate of macroautophagy or the canonical multivesicular body (MVB) pathway because in both cases substrates are released into the vacuolar lumen as part of internal membranes such as autophagic bodies (from autophagosomes) or intralumenal vesicles/ILVs (from MVBs). Thus, vacuolar degradation of GFP-Gas1* shows Pep4-dependent features that are indicative of an unknown post-ER degradation pathway.
To identify cellular components involved in this pathway, we collaborated with the group of Maya Schuldiner and performed a genome-wide high-throughput fluorescence microscopy screen. The complete yeast deletion and Decreased Abundance by mRNA Perturbation (DAmP) libraries were crossed with pep4∆ cells expressing GFP-Gas1*. Imaging of more than 5000 viable double mutants yielded 54 hits, with essentially two distinct phenotypes. One phenotype (43 hits) constitutes an enhanced ER signal and/or intracellular accumulation and/or no signal in the vacuolar membrane or lumen, compatible with a transport block to the vacuole. The other phenotype (11 hits) constitutes a suppressor phenotype with no visible accumulation in the vacuolar membrane and a strong lumenal vacuolar signal, which is compatible with affecting the vacuolar uptake of GFP-Gas1*. Sorting of hits into functional categories according to their annotations provides a layout of the trafficking pathway, and selected hits were further characterized for their roles in Gas1* transport and degradation .
Figure 1. A yeast post-ER degradation pathway links to Pep4-dependent microautophagy. GPI-APs are synthesized at the ER. They are devoid of cytosolic tails and TMDs and known to associate with sphingolipids and sterols in specific membrane domains (rafts). After ER export, correctly folded GPI-APs (blue) are routed to the plasma membrane (PM) via the secretory pathway. Misfolded GPI-APs (red) are diverted from the secretory pathway at the Golgi and are routed to endosomes by a process involving Pep1/Vps10. Unlike membrane-bound cargos that contain cytosolic tails and become ubiquitinated for ESCRT-mediated internalization from endosomes, forming multi-vesicular bodies (MVBs), misfolded GPI-APs fail to internalize at this stage. Instead, after endosome-vacuole fusion, they internalize from the vacuolar membrane into intravacuolar vesicles (IVVs). This microautophagic process is Pep4-dependent and negatively regulated by VTCs; it might be aided by raft-like properties in the vacuolar membrane. Subsequent degradation of IVVs depends on the vacuolar lipase Atg15.
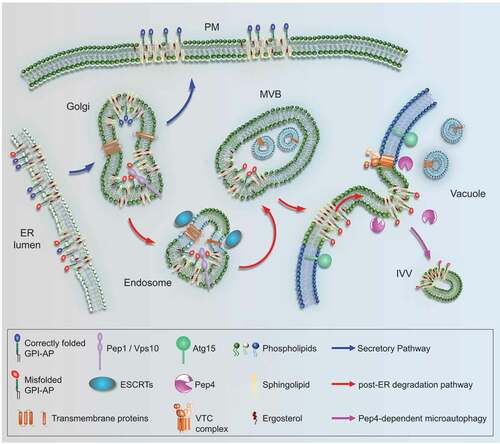
Notably, the Golgi-localized sorting cargo receptor Pep1/Vps10 plays a role in diverting Gas1* from the secretory pathway to endosomes, an early but decisive transport step to the vacuole. Other notable hits include members of the ESCRT machinery with known functions in protein transport between endosomes and the vacuole. Although the best-studied cellular function of ESCRTs concerns protein internalization from endosomes, we find that misfolded GPI-APs are not internalized at this stage. The same observation also illustrates that this post-ER degradation pathway is devoid of cellular components that can compensate for the lack of cytosolic tails on GPI-APs, such as suitable adaptor proteins. Perhaps the need for ESCRTs in this pathway adds to previous reports about their role in fusing endosomes with the vacuole.
Most remarkably, several members of the family of vacuolar transporter chaperones (VTCs), previously known for having roles in microautophagy, were identified as suppressors of the pep4∆ phenotype. This finding provided crucial insight into the fate of misfolded GPI-APs once targeted to the vacuolar membrane. Whereas we initially considered that GPI-APs are degraded directly or indirectly by Pep4 from the lumenal side of the vacuolar membrane, the functional link of this process to the VTCs suggests a new hypothesis, involving protein internalization prior to degradation. Hence, the GPI anchor itself will also be amenable to degradation. We finally validated this hypothesis by using time-lapse confocal fluorescence microscopy under conditions where intravacuolar structures are preserved.
In addition to providing unprecedented insight into the post-ERQC and degradation of a particular class of proteins of the secretory pathway, this study opens a new avenue to investigate features of a novel type of microautophagy. Interestingly, one hallmark of this type of microautophagy, the dependency upon Pep4 activity, has previously been observed for certain types of lipophagy. It will be interesting to reveal how Pep4 is regulating these types of microautophagy, whether and how VTCs are involved in membrane deformation, and how VTCs and Pep4 are mechanistically connected.
Disclosure statement
No potential conflict of interest was reported by the author(s).
Additional information
Funding
Reference
- Lemus L, Matić Z, Gal L, et al. Post-ER degradation of misfolded GPI-anchored proteins is linked with microautophagy. Curr Biol. 2021 Jul 21: S0960-9822(21)00907–6.0. PMID: 34314677. DOI:https://doi.org/10.1016/j.cub.2021.06.078