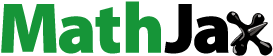
Abstract
Introduction: Breath analyzers are commonly used to test for alcohol intoxication, i.e., elevated systemic levels of ethanol, at workplaces and among vehicle drivers. However, local low-dose exposure to ethanol in the mouth or airways may temporarily increase the breath-alcohol concentration (BrAC) without the systemic ethanol level being affected, leading to false positive test results. The aim of this study was to assess the impact of local ethanol exposure on the BrAC.
Methods: Eleven healthy adults (six women) were exposed to on average 856 mg/m3 ethanol vapor for 15 min, followed by repeat collection of exhaled breath in Tedlar bags. One hour later, the subjects washed their mouth for 30 s with a typical mouthwash containing 22% ethanol and post-exposure breaths were again collected repeatedly. Negligible systemic uptake of ethanol was confirmed by analysis of blood sampled before, between and after the exposures. Ethanol in breath and blood was analyzed by gas chromatography.
Results: No or very low levels (less than 0.002 mg/g) of ethanol were detected in blood at any time point, indicating negligible systemic uptake. The decline in breath was mono-exponential after both exposures with average half times of 0.4 (range 0.3–0.8) min after inhalation exposure and 1.9 (1.1–3.0) min after mouthwash. BrAC levels in the first sample, collected a few seconds after exposure, were 0.14 (0.07–0.13) mg/L after inhalation and 4.4 (2.7–6.0) mg/L after mouth wash. On average, it took 0.5 (0.06–0.7) min and 11 (6–15) min, respectively, for the BrAC to fall below the Swedish statutory limit of 0.1 mg/L air.
Conclusion: In practice, use of breath analysis should not be a problem even if the subject inhaled ethanol vapors before the test. In contrast, use of ethanol-containing mouthwash results in a false positive test if sampling is done within 15 min.
Introduction
Breath analyzers are commonly used to test for alcohol intoxication, i.e., as an indication of elevated systemic levels of ethanol for various purposes including regulatory such as testing for drink-driving. There are several advantages using breath analyzers, including non-invasive sampling and a simple sample matrix (air) which allows for uncomplicated analytical methods and low cost, light-weight, easy to use, direct reading instruments. The use of breath analyzers is based on a well-established, stable partitioning of ethanol between blood and exhaled air, with a blood:breath ratio of 2100 [Citation1] although the ratio varies somewhat with time, with dose, within and between individuals and differs between countries [Citation1–3]. When it comes to drink-driving, countries differ with respect to punishable blood-alcohol concentration (BAC) and breath-alcohol concentration (BrAC) limits. The relation between the BAC and BrAC limits (i.e., the applied BAC:BrAC ratio) also differs somewhat between countries. In Sweden, the statutory BAC and BrAC limits are 0.20 mg/g blood and 0.10 mg/l air, respectively, accounting for a blood/breath ratio (2100) and the density of blood (1.055 g/ml) [Citation4].
When breath analyses are used for regulatory purposes, it is important that they correctly reflect the alcohol intake. Two important potential confounders in this respect are washing the mouth (without swallowing) with ethanol containing products and inhalation of ethanol vapors. For example, people sometimes use mouthwash as an explanation for a positive breath test [Citation5]. Other examples of potential confounders are E-cigarette smoking (as the E-liquid may contain ethanol) [Citation6,Citation7], use of alcohol-based hand rubs, disinfectants or cleaners [Citation8–10] and, oddly, alcohol spill in the car [Citation11].
Confounding of false-high BACs by use of ethanol-containing mouthwash product has been reported by several investigators [Citation5,Citation12–17]. These give a more or less consistent picture of the post-exposure decline in breath, although many of the studies do not report half times and/or limits of detection for breath ethanol (see Discussion for more details).
During inhalation, ethanol (like other polar solvent vapors) is partially dissolved in the mucous surface of the airway epithelium. During exhalation, some of the chemical in the mucous epithelium is released back to air. This so called “washin-washout effect” reduces the systemic uptake and leads to a delay in the post-exposure decline of polar chemical vapor [Citation18]. However, the magnitude of the reduced uptake and delayed release is not well known and has not previously been studied with ethanol. We found only two studies addressing ethanol breath in humans after inhaling ethanol vapor. Tardif and coworkers measured ethanol and acetaldehyde in end-exhaled air during exposure to up to 990 ppm (1870 mg/m3) ethanol [Citation19,Citation20]. However, they did not follow the post-exposure decline in breath and did not relate their results to alcohol breath tests. Some research groups studied dermal and inhalation exposure to ethanol vapors during use of alcohol-based hand rubs [Citation8–10,Citation21,Citation22]. Again, the decline of ethanol in breath was not studied.
The aim of this study was to assess the impact of local ethanol exposure on the BrAC and to what extent this would give a false positive breath test result, i.e., that the BrAC is above the statutory limit although the BAC is negligible. We thus investigated the time course of breath ethanol levels following inhalation of ethanol vapors and mouth rinsing with a typical ethanol-containing mouthwash. Potential systemic exposure was checked by analysis of ethanol in blood.
Materials and methods
Subjects
The study involved 11 healthy subjects, 6 women and 5 men, with a mean age of 28 years (range 21–36) and body mass indices (BMIs) between 17–26 and 20–28 for males and females, respectively. All the volunteers were students recruited by advertisement at a webpage at Karolinska Institutet. Prior to exposure, they underwent a brief medical examination and answered a questionnaire with questions about alcohol consumption, use of medication and medical history. Exposures were performed only after informed written and oral consent. The female volunteers performed a pregnancy test (Colibri Medical AB, Helsingborg, Sweden) immediately before the exposure in order to avoid any unintended fetal exposure. A 24-h abstinence from alcohol consumption before the day of the experiment was requested and confirmed in retrospect by analysis of blood samples collected before the first exposure. The study was approved by the Regional Ethical Review Board in Stockholm.
Chemicals
Ethanol (95%, Kemetyl AB, Haninge, Sweden or 96%, Histolab Products AB, Gothenburg, Sweden) was used to prepare standard curves for air, breath, and blood and to generate ethanol vapor for the chamber exposures. Listerine® (Johnson & Johnson, Maidenhead, UK) containing 21.6% ethanol was used for mouthwash experiments.
Inhalation exposure
Before the beginning of the inhalation exposure, a blank breath sample was collected in breath-gas analysis bags (Tedlar, 1 L, SKC, Inc., Eighty Four, PA) from each subject.
The subjects were exposed seated at resting conditions to ethanol vapors in a 20 m3 dynamic exposure chamber. The ambient air in the chamber had a controlled climate with an average temperature of 24 °C and an average relative humidity of 32%. Ethanol vapors were generated by spraying neat ethanol into a preheated tube connected to the inlet air of the exposure chamber. The vapors, following the inlet air stream, were dispersed throughout the chamber via the ceiling (more details about the exposure chamber are given in [Citation23]). Samples of air in the chamber were collected by an air pump from the upper central part of the exposure chamber and reached a gas chromatograph (GC) through a Teflon®-coated tube for analysis. The average concentration of ethanol during the 15 min exposure was 856 mg/m3 (455 ppm, range 763–950 mg/m3). For comparison, the Swedish 8-h occupational exposure limit (OEL) is 1000 mg/m3 (500 ppm), the 15-min short-term exposure limit (STEL) being 1900 mg/m3 (2000 ppm).
For each subject, a total of ten breath samples (one exhalation per sample) were collected in 1 L Tedlar bags after the 15 min exposure in the chamber was completed. The first breath sample was collected after 15 s and the remaining nine in intervals of 10 s. The subjects were instructed to push hard to fill the bag completely using the same breathing technique as for the breath analyzers. Cleaning was done by filling the bag with clean air, heating it with an electric hair dryer and then completely evacuating it by means of an air pump. The procedure was repeated at least 3 times. Complete decontamination was checked by GC of bag air samples.
Mouthwash exposure
The mouthwash experiment started approximately 1 h after the inhalation experiment.
The subject rinsed the mouth with 20 ml Listerine®, containing 21.6 wt% ethanol, for 0.5 min, in accordance with the product’s instruction. The mouthwash was then discarded and the first breath sample was collected in a Tedlar bag 0.5 min later. Nine additional breath samples were collected with 2 min intervals. The subject was instructed to breath normally with the mouth closed between samplings.
Blood sampling
Before beginning the inhalation exposure, 200 µl capillary blood was sampled from the fingertip. Blood samples were also collected after completion of the breath sampling, i.e., 2 min and 19 min after the inhalation exposure and mouthwash, respectively. The fingers were cleaned with pure water (no alcohol) prior to blood sampling.
Analysis of ethanol
Ethanol levels in exposure chamber air and breath samples were analyzed by GC. The GC system (Clarus 580, Perkin-Elmer) was equipped with a flame ionization detector (FID), a 2 mL volume sample loop and a capillary column (CP-Wax 58, 25 m × 0.32 mm i.d. × 0.02 μm film thickness, Varian). The oven temperature was kept isothermal at 80 °C. The total run time was 1.10 min while the retention time of ethanol was 0.90 min. The calibration curve (six concentrations, 0–660 ppm, R2 = 0.99) was based on standards prepared in Tedlar sample bags (10 L, SKC, Inc., Eighty Four, PA). The limit of detection (LOD) was 0.0007 mg/L air. The sample bags were warmed with a hair dryer to ensure that no condensation of water vapor took place. The bag was then connected to the sample loop in the GC via a short, narrow stainless steel tube and the sample from the bag was pumped into the GC for analysis.
Ethanol in the blood samples was analyzed by headspace GC. The GC system (Clarus 500, Perkin-Elmer) was coupled to a TurboMatrix 40 Trap Headspace (HS) autosampler. The GC was equipped with a FID and a capillary column (PoraPLOT Q, 25 m × 0.53 mm i.d. × 20 μm film thickness, Chrompack) operating at a temperature of 130 °C for 5.0 min. The retention time of ethanol was 3.7 min. Prior to injection, the vial with 200 µl blood was preheated at 60 °C for 25 min in an auto-sampler connected to the HS-GC. Calibration standards were prepared by spiking 200 µl blood with known amounts of ethanol diluted in water. The LOD of the method was 0.00005 mg/g blood.
Kinetic calculations
The decline in breath ethanol (Ct) was fitted to the equation:
(1)
(1)
where t is the time from end of exposure, C0 is the concentration at the start of exposure, Cb is the background (pre-exposure) concentration and k is the elimination rate constant. The three parameters (C0, Cb, k) were estimated by weighted least square fitting using the Solver add-in in Microsoft Excel. The half time was then calculated according to EquationEq. (2)
(2)
(2) :
(2)
(2)
Statistical analysis
Data from the two exposure conditions were compared by Wilcoxon matched pairs test, gender differences were analyzed by Mann-Whitney and Spearman rank correlation was used for analyzes of correlations to BMI. Friedman test was used to check for differences between the three time-points of the blood samples. Statistica v.13, TIBCO Software Inc. was used for all analyzes.
Results
Ethanol in blood
Before the first exposure session, one exhaled air sample was collected from every subject. The average BrAC value was 0.0019 ± 0.006 mg/L. In addition, in the blood samples taken before the first exposure, the average ethanol concentration was 0.00024 mg/g blood. These low values confirm that none of the volunteers had ingested alcohol containing beverages prior to the experiment. The individual pre-exposure BrAC values were used as background concentration (Cb) in the kinetic analyses.
The average BAC values of all volunteers were 0.00073 mg/g and 0.0.00043 mg/g at 3 min after inhalation exposure and 19 min after mouthwash respectively (), indicating negligible systemic uptake of ethanol.
Table 1. The test subjects’ breath (BrAC) and blood (BAC) alcohol concentrations before, between and after inhalation and mouthwash exposure to ethanol.
Inhalation exposure
In all test subjects, ethanol in breath showed a mono-exponential decrease following inhalation exposure ( and Supplementary Figures 1–11). The average first BrAC in the first sample was 0.091 ± 0.016 mg/L (range 0.067–0.126 mg/L). Using a semi-log plot (), the decline of ethanol is in an almost straight line indicating a single half time.
Figure 1. (A) Decline in breath alcohol concentrations (BrAC) and corresponding blood alcohol concentrations (BAC) in one subject after inhalation exposure to 906 mg/m3 ethanol for 15 min. (B) Semi-log plot of A.
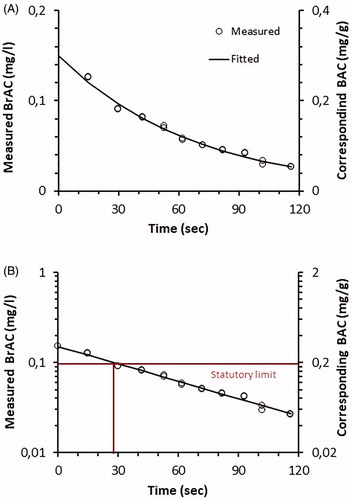
The half time of ethanol after inhalation exposure was 0.42 min and varied among the volunteers (range 0.26–0.78 min, ). The elimination rate constant k ranged between 0.015 and 0.045 min−1 ().
Table 2. Fitted kinetic parameters describing the decrease in breath ethanol after 15 min of inhalation exposure to 856 mg/m3 ethanol.
The first breath sample exceeded the Swedish statutory limit of 0.1 mg/L in two of the 11 test subjects (). Thus, breath sampling shortly after inhalation of ethanol vapor might theoretically result in a false positive test. However, it took less than a minute (up to 0.66 min, or 39 s) for the BrAC to fall below 0.1 mg/L. Thus, in practice it is unlikely that even a relatively heavy exposure to ethanol vapor would result in a positive test result.
All calculations were corrected for the individual exposure concentration.
None of the kinetic parameters differed between genders and none correlated with the BMI.
Mouthwash
The same pattern of a mono-exponential decrease was seen after mouthwash () as after ethanol inhalation (). However, as described further below, the initial BrAC was markedly higher and the half time much longer after mouthwash than after inhalation exposure. In fact, in all subjects the BrAC values attained after mouthwash were so high that they would have given a false positive response in a breath test. On average, it took 10.6 min (range 5.7–15.2 min) for the BrAC to fall below the Swedish statutory limit of 0.1 mg/L ().
Figure 2. (A) Decline in breath alcohol concentrations (BrAC) and corresponding blood alcohol concentrations (BAC) in one subject after rinsing with ethanol containing mouthwash for 0.5 min. (B) Semi-log plot of A.
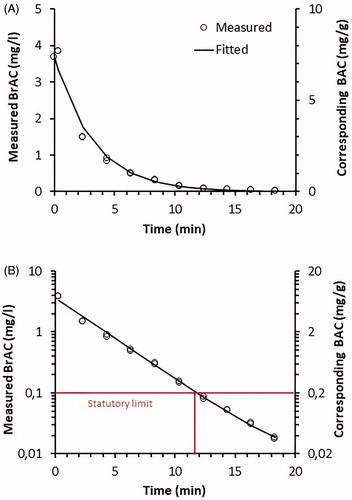
Table 3. Fitted kinetic parameters describing the decrease in breath ethanol after rinsing with ethanol containing mouthwash containing 21% ethanol for 0.5 min.
Comparison between inhalation exposure and mouthwash
The BrAC value in the first breath sample sampled after mouthwash was markedly higher than after inhalation exposure (4.3 vs. 0.14 mg/L, p = 0.0004, Wilcoxon matched pairs test). The half time was also significantly longer after mouthwash (1.9 min vs. 0.42 min, p = 0.003). A comparison between the time to fall below the statutory limit after the two exposures (range 0.06–0.66 min after inhalation exposure and 5.7–15.2 min after mouthwash) yielded a significant difference (p = 0.003, Wilcoxon matched pairs test).
Discussion
We measured the elimination of breath ethanol in volunteers after local exposure by inhalation and via mouthwash. Fingertip capillary blood samples collected after the exposures confirmed that the systemic uptake of ethanol was negligible. The decrease in BrAC was mono-exponential after both exposures, but with a five-fold slower decline after mouthwash (average half times of 1.9 versus 0.42 min). In addition, the intial BrAC was – 30-fold higher after mouthwash (4.3 versus 0.14 mg/L). The exposure levels in our study (763–950 mg/m3) were similar to the Swedish 8-h OEL of 1000 mg/m3 (500 ppm), the 15-min STEL being 1900 mg/m3 (1000 ppm). The OELs and STEL differ somewhat between countries, e.g., in Germany they are 380 mg/m3 (200 ppm) and 1520 mg/m3 (800 ppm), while the American Conference of Industrial Governmental Hygienists (ACGIH) recommends a STEL of 1880 mg/m3 (1000 ppm). The ethanol toxicokinetics are first-order at these low concentrations, therefore a doubling of the exposure level would result in double BrAC levels at any time point, with no change in half time. Thus, the time for BrAC to fall below the statutory limit would take one half time longer. Likewise, the BrAC levels after mouthwash will depend on the ethanol percentage in the mouthwash (as well as volume and duration). In our study, we chose to use the product with the highest ethanol content available on the Swedish market, i.e., with 22%. In other studies, mouthwash with ethanol content between 4 and 30% has been used [Citation5,Citation13].
In the calculation of individual kinetic data, constant background BrAC levels over time were assumed, using the individual pre-experiment BrAC value from each subject. This may introduce a small error in the estimates of the kinetic parameters, should the background BrAc change during the course of the experiment.
We found no reports on the breath kinetics following inhalation exposure to known concentrations of ethanol. Two studies have measured BrAC in humans during, but not after, exposure to ethanol [Citation19,Citation20]. Several investigators have studied BrAC after use of ethanol containing hand sanitizers [Citation8,Citation9,Citation21], see also review by McClean et al. [Citation10]. Although exposure via hand sanitizers is realistic from a practical point of view, the BrAC data are difficult to interpret as the inhaled concentration of ethanol were unknown.
The effect of mouthwash on BrAC has been studied by several investigators. Modell et al. [Citation5] had 10 healthy subjects rinse the mouth for 15 s with three brands of mouthwash containing 6–27% ethanol. Measurements with a breath analyzer indicated a monoexponential decrease and all BrAC values were below 0.1 mg/L after 15 min for all three brands. Foglio-Bonda et al. [Citation13] measured breath ethanol in 30 volunteers after mouth rinsing with 21.6% ethanol. All estimated BAC levels were below 0.5 g/L blood, corresponding to 0.22 mg/L in breath (the authors used a partition coefficient of 2300) after 10 min. After 20 min, ethanol could not be detected in the breath from any of the subjects.
Other studies report that BrAC was back to baseline levels 13 min after mouthwash with 16–18% [Citation17] and 21.6% ethanol [Citation12], respectively. In a study by Gullberg [Citation15], three volunteers rinsed their mouth with 40% ethanol for 10 s. BrAC was practically zero after 8–14 min. Half times were not given but we estimate them to 1.1–2.1 min based on the slope constants. Wigmore and Leslie [Citation16] measured BrAC at 5 min and 10 min after rinsing with 10 ml of 20% ethanol for 10 s. Half time data were not presented, but two thirds had BrAC below the statutory limit (0.01 g/210 L) after 10 min.
In a study by Franklin and Stevens [Citation14], subjects rinsed their mouth with wine containing 13% ethanol. BrAC was back to zero after 15 min (only measured at 15 min). Dubowski [Citation24] followed BrAC after ethanol ingestion in 55 subjects. They retained the 11.4% ethanol for 1 min in the mouth. Repeat minute by minute measure showed a decline in BrAC but only mean data and no half times or slope constants were given. Zero BrAC was achieved after 9 min (mean value). Judged by the semilog plot the halftime was approximately 1.5 min.
The referred studies had different designs with respect to, e.g., ethanol percentage, volume and duration of mouthwash, frequency and duration of breath sampling and analytical methods. Furthermore, limits of detection were not stated, therefore statements on “zero” or “practically zero” levels could not be evaluated with exactness. Nevertheless, all the studies are seemingly in good agreement with our study, wherein it took 6–16 min for the BrAC to fall below 0.1 mg/L.
As expected, the post-exposure BrAC was markedly higher (higher concentration in first breath and estimated at time zero) and decreased slower (longer half time) after mouthwash than after inhalation exposure ( and ). The difference is mainly explained by massive differences in dose. Thus, the total amount of ethanol inhaled during 15 min of exposure at 1000 mg/m3 is roughly 150 mg (assuming a lung ventilation of 10 L/min), of which a small and unknown fraction is absorbed in the linings of the airways and desorbed during exhalation. In comparison, the amount of ethanol used (of which an unknown fraction dissolves in the oral epithelium and is released back during exhalation) in the rinsing procedure with 20 mL mouthwash is 4320 mg. The 29-fold difference in amounts fits remarkably well with the 31-fold difference in BrAC values immediately after exposure. In spite of the apparent similarities after adjustment for “dose”, other factors such as differences in site and duration of absorption may also explain the differences. One may speculate that the inhaled ethanol vapor is spread and absorbed over large areas with rich blood supply and relatively thin epithelial linings (tissue thickness 30–160 μm, down to 0.1 μm in the alveoli [Citation25]). This allows for rapid washout via blood as well as via exhalation.
Zang and Kleinstreuer [Citation26] showed that inhaled ethanol vapors deposit substantially in the upper airways by modelling transport and uptake of ethanol vapors. This so called “washin-washout effect” was also shown by Johanson [Citation18]. In contrast, during mouth rinsing the ethanol is spread over and absorbed by a small surface area (oral cavity only) with comparatively poor blood supply and thick epithelium (99–294 μm, depending on location [Citation25]. Thus, the washout from the oral cavity via blood and exhalation is expected to be less effective, resulting in a slower decrease and longer half time in expired breath compared to the lower airways.
Previously, the breath kinetics of another water soluble substance, hydrogen cyanide, has been studied after 10 min of low-level exposure (10 ppm) [Citation27]. The experiment gave an average half time in breath of 0.27 (range 0.17–0.40) min, i.e., approximately half of that found for ethanol in the present study. The contribution from washin-washout from the airways was negligible [Citation27]. Disregarding toxic potencies and looking at breath kinetics only, the results from the two studies support one another, as the shorter half time of hydrogen cyanide can be explained by its higher volatility (gas vs. liquid at room temperature) and lesser polarity (water:air partition coefficient 320 [Citation27] versus 2133 [Citation28]), compared to ethanol).
It is not surprising that low levels of ethanol were detected prior to the exposures. Various alcohols, including ethanol, are generated endogenously via various metabolic procedures, predominantly by microbial fermentation of ingested sugars in the gut [Citation28], although other organs like the gastrointestinal tract and the liver are capable of alcohol synthesis as well [Citation29]. Ostrovsky [Citation30] reported endogenous blood concentrations of 0.0001–0.0003 mg/ml, in agreement with our finding of 0.0001–0.0005 mg/g.
Only 11 subjects were included in our study. However, the washout kinetics of ethanol depend on physicochemical processes – mass transfer due to bulk flow, diffusion and dissolution – which in turn depend on air flow velocity (breathing pattern, lung ventilation rate), temperature, geometry of the airways, and epithelial and mucosal thickness. Out of these, air flow velocity is expected to vary significantly between adults. For example, hypo- and hyperventilation would result in slower and faster decrease in breath ethanol, respectively. The subjects did not receive any instruction on how to breathe. Nevertheless, there were no signs of abnormal breathing in any of them. Thus, we believe these results are generally applicable to the adult population.
Conclusions
This study shows that, in practice, inhalation of ethanol, even if carried out immediately before the breath alcohol test, does not result in an overestimate of the true BrAC value. In contrast, use of mouthwash might overestimate BrAC. People suspected of drunken driving, sometimes use mouthwash as an excuse when found positive in a breath alcohol test. However, in our study the BrAC fell below the Swedish statutory limit (0.10 mg/L) in less than 16 min in all 11 subjects. This suggests that the use of mouthwash does not explain a positive alcohol breath test as, in practice, it takes longer to get the car started, driving, be stopped by the police and carry out the breath test. In any case, a positive alcohol breath tests can easily be verified by repeating the test after 15 min or using a 15-min period of observation before testing, as already applied in many countries (e.g., California, Canada). Thus, after a positive breath test in Sweden, the driver is offered to breathe in an evidence instrument (Evidenzer, infrared technique) twice with 6–9 min in between or to take a venous blood sample. In California, a 15 min observation period is required before performing evidential roadside screening breath test [Citation12]. Likewise, two breath tests are performed with 15 min in between for legally purposes in Canada [Citation31].
Supplemental Material
Download MS Power Point (132.1 KB)Disclosure statement
No potential conflict of interest was reported by the authors.
References
- Jones AW. Evidence-based survey of the elimination rates of ethanol from blood with applications in forensic casework. Forensic Sci Int. 2010;200:1–20.
- Jaffe DH, Siman-Tov M, Gopher A, et al. Variability in the blood/breath alcohol ratio and implications for evidentiary purposes. J Forensic Sci. 2013;58:1233–1237.
- Haffner HT, Graw M, Dettling A, et al. Concentration dependency of the BAC/BrAC (blood alcohol concentration/breath alcohol concentration) conversion factor during the linear elimination phase. Int J Legal Med. 2003;117:276–281.
- Jones AW, The relationship between blood alcohol concentration (BAC) and breath alcohol concentration (BrAC): a review of the evidence. London (UK): Road Safety Web Publication; 2010. p. 1–43.
- Modell JG, Taylor JP, Lee JY. Breath alcohol values following mouthwash use. JAMA 1993;270:2955–2956.
- Valentine GW, Jatlow PI, Coffman M, et al. The effects of alcohol-containing e-cigarettes on young adult smokers. Drug Alcohol Depend. 2016;159:272–276.
- Poklis JL, Wolf CE, Peace MR. Ethanol concentration in 56 refillable electronic cigarettes liquid formulations determined by headspace gas chromatography with flame ionization detector (HS-GC-FID). Drug Test Analysis. 2017;9:1637–1640.
- Emerson BL, Whitfill T, Baum CR, et al. Effects of alcohol-based hand hygiene solutions on breath alcohol detection in the emergency department. Am J Infect Control. 2016;44:1672–1674.
- Strawsine E, Lutmer B. The effect of alcohol-based hand sanitizer vapors on evidential breath alcohol test results. J Forensic Sci. 2018;63:1284–1290.
- MacLean RR, Valentine GW, Jatlow PI, et al. Inhalation of alcohol vapor: measurement and implications. Alcohol Clin Exp Res. 2017;41:238–250.
- Straka L, Novomesky F, Marcinkova M, et al. An unusual case of highly false-positive breath-alcohol test in a motor vehicle driver. RJLM. 2017;25:293–296.
- Fessler CC, Tulleners FA, Howitt DG, et al. Determination of mouth alcohol using the dräger evidential portable alcohol system. Sci Justice. 2008;48:16–23.
- Foglio-Bonda PL, Poggia F, Foglio-Bonda A, et al. Determination of breath alcohol value after using mouthwashes containing ethanol in healthy young adults. Eur Rev Med Pharmacol Sci 2015;19:2562–2566.
- Franklin SD, Stephens A. Can wine tasting be used as a defence to a charge of excess alcohol?. Sci Justice. 2000;40:39–40.
- Gullberg RG. The elimination rate of mouth alcohol: mathematical modeling and implications in breath alcohol analysis. J Forensic Sci. 1992;37:1363–1372.
- Wigmore JG, Leslie GM. The effect of swallowing or rinsing alcohol solution on the mouth alcohol effect and slope detection of the intoxilyzer 5000. J Anal Toxicol. 2001;25:112–114.
- Worner TM, Prabakaran J. The accuracy of breath alcohol analysis using the breathalyzer. Alcohol Alcohol. 1985;20:349–350.
- Johanson G. Modelling of respiratory exchange of polar solvents. Ann Occup Hyg. 1991;35:323–339.
- Tardif R, Liu L, Raizenne M. Exhaled ethanol and acetaldehyde in human subjects exposed to low levels of ethanol. Inhal Toxicol. 2004;16:203–207.
- Dumas-Campagna J, Tardif R, Charest-Tardif G, et al. Ethanol toxicokinetics resulting from inhalation exposure in human volunteers and toxicokinetic modeling. Inhal Toxicol. 2014;26:59–69.
- Ahmed-Lecheheb D, Cunat L, Hartemann P, et al. Dermal and pulmonary absorption of ethanol from alcohol-based hand rub. J Hosp Infect. 2012;81:31–35.
- Hautemaniere A, Cunat L, Ahmed-Lecheheb D, et al. Assessment of exposure to ethanol vapors released during use of Alcohol-Based Hand Rubs by healthcare workers. J Infect Public Health. 2013;6:16–26.
- Ernstgård L, Lind B, Johanson G. Acute effects of exposure to vapours of standard and dearomatized white spirits in humans. 1. Dose-finding study. J Appl Toxicol. 2009;29:255–262.
- Dubowski KM. Studies in breath-alcohol analysis: biological factors. Z Rechtsmed. 1975;76:93–117.
- Prestin S, Rothschild SI, Betz CS, et al. Measurement of epithelial thickness within the oral cavity using optical coherence tomography. Head Neck. 2012;34:1777–1781.
- Zhang Z, Kleinstreuer C, Kim CS. Transport and uptake of MTBE and ethanol vapors in a human upper airway model. Inhal Toxicol. 2006;18:169–184.
- Stamyr K, Nord P, Johanson G. Washout kinetics of inhaled hydrogen cyanide in breath. Toxicol Lett. 2008;179:59–62.
- Jones AW. Determination of liquid/air partition coefficients for dilute solutions of ethanol in water, whole blood, and plasma. J Anal Toxicol. 1983;7:193–197.
- de Lacy Costello B, Amann A, Al-Kateb H, et al. A review of the volatiles from the healthy human body. J Breath Res. 2014;8:014001
- Ostrovsky YM. Endogenous ethanol–its metabolic, behavioral and biomedical significance. Alcohol 1986;3:239–247.
- Martin TL, Solbeck PA, Mayers DJ, et al. A review of alcohol-impaired driving: the role of blood alcohol concentration and complexity of the driving task. J Forensic Sci. 2013;58:1238–1250.