Abstract
Background
The clinical characteristics following self-poisoning with organophosphorus (OP) insecticides differs according to the insecticide ingested. Phenthoate is a dimethoxy WHO Hazard Class II OP pesticide with limited literature on its clinical characteristics and outcome. We aimed to better understand its clinical characteristics by studying patients with phenthoate self-poisoning in Sri Lanka.
Methods
We conducted a prospective cohort study of patients presenting with phenthoate self-poisoning to eight hospitals in Sri Lanka between 2002 and 2018. Clinical outcomes were recorded for each patient. Blood samples for measuring plasma phenthoate concentration, cholinesterase activity, and response to oximes were available for a very small number of patients recruited to a clinical trial.
Results
Two hundred and ninety-two patients who ingested agricultural phenthoate formulations were included in the study. Median time to admission was 3.9 (IQR 2.4 - 6.8) h. Forty-two (14.4%) patients were intubated, mostly (30/37, 81%) within 24 h of ingestion (median time to intubation 7.2 [IQR 2.6–20.9] h). Median duration of intubation was 74.8 (IQR 26.8–232.5) h; the longest duration in a survivor was 592 h. Nineteen died (case fatality 6.5%, 95% CI 4.0–10.0); median time to death was 37 (IQR 16 - 101.7) h. Median plasma phenthoate concentration in patients with samples (n = 81) was 135 (IQR 62.7–356.5) ng/mL (0.42 µmol/mL [0.2 to 1.1 µmol/mL]). Five of six patients receiving pralidoxime chloride 2 g showed an initial increase in AChE and BuChE activity that was not sustained despite an infusion of pralidoxime.
Conclusion
Phenthoate self-poisoning has a 6.5% case fatality rate. Most patients who experience respiratory failure undergo early intubation; most deaths occurred among those patients who were intubated less than 24 h after ingestion. There was a non-sustained increase in cholinesterase activity with pralidoxime, but further studies are required to analyse the extent to which oximes are clinically effective in phenthoate self-poisoning.
Introduction
Organophosphorus (OP) insecticide self-poisoning results in an estimated 100,000 deaths per year [Citation1]. OP compounds inhibit acetylcholinesterase (AChE, EC 3.1.1.7) – an enzyme that catalyses the breakdown of acetylcholine into choline and acetic acid – resulting in excessive accumulation of acetylcholine at cholinergic synapses, producing an acute cholinergic syndrome [Citation2,Citation3]. Over 100 OP compounds have been used in agriculture; most are derivatives of phosphoric, phosphonic or phosphinic acid [Citation4]. However, there is marked variation in the poisoning that occurs with different OP insecticides [Citation5,Citation6]. The chemical structure of OPs affect their lethality, potency as cholinesterase inhibitors, duration of action, and responsiveness to treatment with oximes [Citation5,Citation7–9]. In particular, phosphorothioate (P = S) OP insecticides have little to no inhibitory activity themselves and require metabolism to the oxon (P = O) before they inhibit AChE [Citation4].
OP insecticide poisoning results in respiratory failure, through initial loss of central respiratory drive and lung fluid overload, causing hypoxia, followed later by neuromuscular junction failure [Citation10,Citation11]. Despite improvement in the medical management of acute OP poisoning, case fatality for individual pesticides has shown only a minor decrease over time [Citation12]. The lack of clinical protocols specific to poisoning by particular OP insecticides has been proposed as a factor leading to poor outcome [Citation5,Citation13].
The WHO hazard classification of pesticides from Class Ia (extremely hazardous) to Class IV (unlikely to present acute hazard) is based on the rat oral LD50 for the active insecticide ingredient [Citation14]. Class Ia and Ib pesticides are increasingly being banned from agriculture worldwide, making WHO Class II (moderately hazardous) OP pesticides more commonly used [Citation5,Citation15,Citation16]. However, within WHO Class II itself, there is much variation in lethality, responsiveness to oximes, and clinical characteristics, including ventilatory requirement and duration of ventilation [Citation5,Citation8,Citation17].
Phenthoate is a dimethoxy phosphorothioate (‘thion’) WHO Hazard Class II OP insecticide with a rat oral LD50 of around 400 mg/kg (). It is metabolised by cytochrome P450 systems to the inhibiting agent phenthoate oxon, as well as demethyl phenthoate oxon acid, demethyl phenthoate acid, O,O- dimethyl phosphorothioic acid, and phosphorothioic acid metabolites in mammals [Citation18,Citation19]. There have been few reports of poisoning, with only two fatal cases and two case series (totalling 6 patients) reported in the literature [Citation20–22]. However, as more highly hazardous pesticides are withdrawn from agriculture, phenthoate may become more important. To better understand the clinical characteristics of acute phenthoate poisoning, we analysed data on phenthoate self-poisoned patients recruited to a prospective cohort study in eight Sri Lankan secondary hospitals between 2002 and 2018. A small number of these patients were recruited to a clinical trial of pralidoxime [Citation23], offering very preliminary information on cholinesterase inhibition and response to oximes.
Table 1. Phenthoate properties.
Methods
Patients
Patients were recruited from eight different secondary hospitals in Sri Lanka between the years 2002 and 2018 as previously published [Citation5,Citation8,Citation9]. Patients were included based on their history of phenthoate ingestion as described by themselves, their family members, notes from the transferring doctor, or the presence of a pesticide bottle. All patients who reported ingestion/co-ingestion of a pesticide other than phenthoate and those who co-ingested substances other than alcohol were excluded from the study.
Clinical data from patients was obtained regularly by research staff as described previously [Citation5,Citation8,Citation9]. All patients were seen regularly by full time study doctors at least twice each day and more according to clinical need. Significant events (intubation, seizures, and deaths) were recorded at the time of events. Patients were seen at the medical ward first and seriously ill patients were transferred to the intensive care unit (ICU) based on glasgow coma score (GCS) and cardiorespiratory function as beds became available. Criteria for intubation were tidal volume <180 mL/breath using a Wright’s respirometer, respiratory rate <10 breaths/min, or failure of a Guedel airway to preserve airway patency. Arterial blood gases were not available to help guide therapy. Hypotensive patients who did not respond to 50–100 mg total loading doses of atropine and fluid resuscitation (with 2 L of normal saline) were treated with dopamine plus dobutamine (both started at 5–10 μg kg−1 min−1 and increased as necessary) by infusion pump as per standard local practice. Norepinephrine and epinephrine infusions were not used; bolus epinephrine (1–3 mg intravenously) was administered for cardiac arrests as per standard Advanced Life Support guidelines.
Ethics approval for this data collection was obtained from the research ethics committees of University of Colombo, Sri Lankan Medical Association, University of Peradeniya, Australian National University, University of New South Wales, University of Sydney, and Oxford Tropical Medicine Ethics Committee.
Toxicological analysis
Blood samples were taken from a subset of phenthoate-poisoned patients in 2004–2006 as part of an RCT of pralidoxime or an RCT of activated charcoal [Citation23], to test the accuracy of the history of phenthoate ingestion and measure AChE/butyrylcholinesterase (BuChE, EC 3.1.1.8) reactivation. As previously reported, for red cell AChE measurement (normal value 600–700 mU/mmol Hb [Citation23,Citation24]), 0.2 mL of EDTA blood was diluted at the bedside into 4 mL of cooled saline and rapidly frozen to −20 °C. Plasma was then separated from a second EDTA blood sample for BuChE analysis (normal range 3000–6000 mU/ml) [Citation25,Citation26] and frozen at −20 °C. All analyses were done in Munich. AChE activity was assayed according to a modified Ellman method, using ethopropazine to inhibit butyrylcholinesterase (BuChE) activity [Citation25]. Re-activatability of AChE (ageing) and BuChE were assessed as described [Citation5,Citation27]. Phenthoate was quantified in additional samples as described in a previously published study [Citation9]. Plasma samples were assayed in Adelaide, South Australia by liquid chromatography mass spectrometry using an AB Sciex 6500+ triple-quadrupole mass spectrometer with Ion Drive™ Turbo V ion source (Sciex, Canada) using the electrospray ionization probe in positive mode and with a lower limit of quantitation of 1 ng/mL.
Data analysis
Data were analysed using MS Excel and Graphpad Prism (version 9). Descriptive statistics were used to summarise categorical variables. Median and inter-quartile ranges (IQR) were used for analysing data that did not fit a normal distribution. We used a two sample t-test to test to compare the difference in mean phenthoate concentrations in 61 patients with GCS >13 versus GCS ≤13. A p-value < 0.05 was considered significant. Figures were made using Graphpad Prism and Adobe Illustrator CS4.
Results
We identified two hundred and ninety-two patients who had ingested agricultural formulations of phenthoate between the years 2002 and 2018 of which 19 died (case fatality 6.5%, 95% CI 4.0–10.0). Most patients were male (218, 74.7%). The median age was 30 (IQR 23 − 42) years () and time to secondary hospital admission was 3.9 (2.4 − 6.8) h post-ingestion. Most patients were conscious at the time of presentation with a high median GCS of 15/15; 22 (7.5%) patients had GCS < 10 at presentation while 17 patients (5.8%) were comatose (GCS 3/15).
Table 2. Patient outcomes.
Respiratory failure and mortality
Of the 292 patients, 42 (14.4%, 95%CI 10.8–18.9) were intubated, of whom 28 (66.7%) survived and 14 (33.3%) died. Five patients were intubated on more than one occasion.
The median time to first intubation from poisoning (available for 37/42 patients) was 7.2 (IQR 2.6 - 20.9) h post-ingestion (). Most (30/37, 81%) were intubated after less than 24 h, while seven (18.9%) were intubated after 24 h. The median duration of ventilation was 74.8 (IQR 26.8 - 232.5) h (). The longest duration of ventilation in a survivor was 592 h.
Figure 1. Box violin plots showing distribution of patients’ time from ingestion to first intubation in graph A (n = 37), duration of ventilation in graph B (n = 41), and time to death from ingestion in graph C (n = 19).
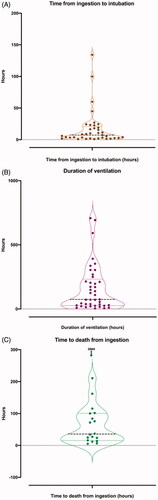
Nineteen patients (6.5%, 95% CI 4.0–10.0) died, five without being intubated. Median time to death was 37 h (IQR 16 − 101.7) h (). Twelve (63%) patients presented with reduced consciousness (GCS ≤13). Median GCS at presentation of patients who died was 11 (IQR 3 − 15) and patients who survived was 15 (IQR 15 − 15).
Early and late intubations
The median time to respiratory failure in the seven patients who were intubated late was 44.8 (IQR 24.5 − 99.9) h. The duration of ventilation in survivors was modestly longer for patients intubated early versus those intubated after 24 h (median [IQR] 172.6 [74.8–303.7] h vs 107.1 [24.5–222.9] h). Three patients who were intubated early and two patients who were intubated late required intubation more than once.
Fourteen (33.3%) patients who were intubated died. Of those for whom a time to intubation was known (n = 12), all (except one intubated at 24.5 h) were intubated before 24 h post-ingestion. The median time to death among the twelve intubated patients was 54.3 (IQR 24 − 96.1) h. The maximum duration of mechanical ventilation of a patient who was intubated early and did not survive was 72 h.
Phenthoate blood concentrations
Median plasma concentration of phenthoate (available for 81 patients) was 135 (IQR 62.7–356.5) ng/mL (0.42 µmol/mL [0.2 to 1.1 µmol/mL]). Overall phenthoate concentration at admission was higher in early presentations than late presentations to the hospital (). Initial median phenthoate concentration was 134.8 (IQR 68.2 − 340) ng/mL for presentations under 12 h post-ingestion and a median of 26 (IQR 19.2 − 80.8) ng/mL/mL for presentations after 12 h. There was no statistically significant difference (p = 0.94) in mean phenthoate concentration among patients with a GCS ≤13 compared to those with GCS > 13. Three out of eighty-one patients died, with variable admission plasma phenthoate concentration levels of 34.9, 132, and 309 ng/mL. Breast milk samples taken from three patients showed low phenthoate concentrations, of between 10.0 and 32.0 ng/mL.
Figure 2. Relationship between time since poisoning and initial plasma phenthoate concentration (ng/ml) for 59 patients. Three outliers [(106, 17), (1.6, 2601) and (1.5, 5257)] are represented with arrows. Patients present with variable amounts of phenthoate concentration in their blood, with an apparent trend towards lower phenthoate concentrations in late presentations.
![Figure 2. Relationship between time since poisoning and initial plasma phenthoate concentration (ng/ml) for 59 patients. Three outliers [(106, 17), (1.6, 2601) and (1.5, 5257)] are represented with arrows. Patients present with variable amounts of phenthoate concentration in their blood, with an apparent trend towards lower phenthoate concentrations in late presentations.](/cms/asset/c79406f0-929b-419d-a027-5653593e5c42/ictx_a_1917596_f0002_b.jpg)
Cholinesterase inhibition and activation
Cholinesterase inhibition assays were performed in a small sample of eight patients recruited to an RCT of pralidoxime 2 g over 20 min, followed by an infusion of 0.5 g/h [Citation23]. Six patients were randomised to receive pralidoxime and two to placebo.
Median red cell AChE at admission (median 8.3 [IQR 2.9–16.2] h post-ingestion) was 31.5 mU/µmol Hb (IQR 3.75–146, range 0–255, ), far below the lower limit of normal (600 mU/µmol Hb). However, although all were symptomatic, only three had GCS ≤13, associated with admission AChE activities of 3, 6, and 173 mU/µmol Hb. The patients who were fully conscious had admission AChE activities of 12, 51, 65, and 255 mU/µmol Hb. Median BuChE concentration was 1022 mU/ml (IQR 133.8–2,031, range 1–4013, ), showing less severe inhibition of BuChE compared to AChE. Cholinesterase inhibition varied markedly at baseline as expected for such a small sample.
Figure 3. AChE and BuChE activity in 8 patients. Red symbols represent patients receiving pralidoxime. Blue symbols represent patients receiving placebo. Each symbol shape represents a different patient. The graph shows a modest increase in cholinesterase activity in the first hour among 5 out of 6 patients who received pralidoxime.
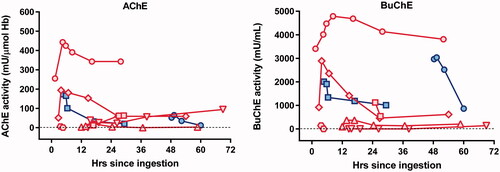
Six patients received pralidoxime, of which five showed modestly increased AChE activity after treatment. For four patients with detectable AChE activity at baseline (), in vivo AChE activity increased in the first hour by a median of 50 (IQR 22 − 165.5, range 5–188) mU/µmol Hb. The fifth who presented after 14.6 h showed an increase from zero to 39 mU/µmol Hb in the first hour after administration; the sixth who presented after 3.6 h showed no increase (6 mU/µmol Hb to zero). This rapid effect (too fast for ageing) may be due to changes to the phenthoate in the bottle, before ingestion. Such reactions have been noted with dimethoate, forming isodimethoate, which can result in intractable AChE inhibition [Citation28]. AChE reactivation was poorly maintained at 12 h. There was a modest increase in BuChE activity (median increase of 272 [IQR 8.1–1519] mU/ml) in five of six patients receiving pralidoxime (). The AChE activity in those who didn’t received pralidoxime decreased by 8 mU/µmol Hb (4.6% from baseline) in the first patient, and increased by 13 mU/µmol Hb (25.7% from baseline) in the second patient.
Discussion
In this large case series of 292 patients with phenthoate self-poisoning, we found phenthoate to cause a less severe poisoning than some other similar WHO hazard Class II OP insecticides. It is associated with a moderate case fatality, a shorter duration of ventilation than other dimethoxy OPs studied, and modest short-lived reactivation of AChE following oxime administration.
The case fatality of phenthoate (6.5%) is in the mid-range of case fatality for OP insecticide self-poisoning [Citation29]. Within the spectrum for lethality of WHO Class II OPs, only diazinon is known to be less lethal than phenthoate in humans [Citation29]. However, many other WHO hazard class II insecticides – for example neonicotinoids, fipronil – have lower human toxicity after ingestion [Citation29]. The case fatalities of chlorpyrifos (8%), fenthion (16.2%), and dimethoate (23.1%) are all higher than phenthoate with minor variations between different studies [Citation5,Citation29]. An exception to this is a study that included a smaller number of patients with Class II OP poisoning, where the mortality from chlorpyrifos (5.3%), dimethoate (4.8%), and quinalphos (4.3%) poisoning appears to be lower than the expected standard for class II OPs as suggested by larger cohort studies [Citation30]. Analysis of phenthoate case fatality over time showed no effect between 2002 and 2019, despite improvements in medical management and a steady decline in overall case fatality of pesticide poisoning [Citation12].
A majority of patients (73.7%) who required intubation died, indicating a high mortality rate among those who required intubation. This could be due to inadequate resources such as lack of arterial blood gases, norephinephrine and epinephrine infusions in the intensive care unit, or due to the mechanisms of respiratory failure in phenthoate poisoning itself. The majority of phenthoate poisoned patients with respiratory failure required intubation soon after poisoning, rather than after 24 h [Citation5,Citation10]. Early respiratory failure in OP poisoning is generally associated with shorter duration of ventilation and a better prognosis overall [Citation10,Citation31]. However, in patients poisoned with phenthoate, nearly all the intubated patients who died were intubated early and had a shorter duration of mechanical ventilation compared to those who were intubated late. This suggests that delayed respiratory failure/intermediate syndrome is not a major problem with phenthoate poisoning.
Most patients with phenthoate self-poisoning presented fully alert (GCS 15/15), with only 7.5% presenting with impaired consciousness (GCS < 10). The majority of patients (63%) who died had reduced GCS (≤13) on presentation, similar to dimethoate but quite different to slow-acting fenthion [Citation5,Citation32]. For fenthion, severe poisoning symptoms are usually delayed due to slow inhibition of AChE (probably due to slow conversion from thion to oxon and large volume of distribution). Phenthoate is of modest lipophilicity (logKow 3.69 compared to 4.3 and 5.05 for fenthion and chlorpyrifos, respectively). Unlike fenthion, delayed toxicity and intubation were uncommon after phenthoate poisoning.
The plasma phenthoate concentration was lower than dimethoate but higher than fenthion, profenofos, and chlorpyrifos [Citation5,Citation8], suggesting a smaller volume of distribution consistent with the modestly lower logKow. Red cell AChE activity was severely inhibited while BuChE inhibition was relatively modest. Median AChE activity on admission for phenthoate for the few patients studied was substantially lower than median AChE activity in larger cohorts of fenthion, profenofos, dimethoate and chlorpyrifos self-poisoning [Citation5,Citation8]. Median BuChE activity in phenthoate at admission was similar to dimethoate poisoning and far less inhibited than median BuChE in patients who ingested chlorpyrifos, quinalphos and fenthion [Citation27,Citation33].
The evidence for the benefit of oximes in acute OP poisoning is inconclusive [Citation34,Citation35]. Modest and poorly sustained reactivation of AChE and BuChE was noted in five out of six patients with ChE assays performed around pralidoxime administration, perhaps associated with their relative late presentation (median 8 h). Reactivation of AChE and BuChE in the first hour was smaller than reactivation after pralidoxime in chlorpyrifos, quinalphos, dimethoate, and fenthion poisoned patients [Citation33]. Red cell AChE inhibition is thought to correlate with clinical severity of poisoning [Citation36], except for S-alkyl OPs [Citation8,Citation37]. Although the level of consciousness is a good marker of severity and outcome generally for OP insecticide poisoning [Citation32], there is insufficient evidence to study the relationship between AChE inhibition and severity of unconsciousness in these phenthoate poisoned patients.
Analysis of phenthoate metabolites in the urine and blood of a human phenthoate fatality failed to identify the active phenthoate oxon, suggesting that it may rapidly bind to AChE and break down, similar to chlorpyrifos oxon [Citation22,Citation38,Citation39].
Limitations
This was a large prospective case series of phenthoate patients; however, it lacked information on co-ingestion of alcohol, which is common amongst poisoned men [Citation40]. We have previously noted that alcohol ingestion itself did not affect outcome in dimethoate OP poisoning, although it did affect the quantity of pesticide ingested [Citation26]. Another limitation of the study was the lack of plasma phenthoate concentration data for patients whose breast milk were analysed for phenthoate concentration, which would have been useful to gain a better understanding of phenthoate properties. We did not have access to information on solvents for phenthoate sold in Sri Lanka as this is not publicly available data, which prevented analysis of formulations. Furthermore, exploratory analysis on factors associated with a poor outcome was limited by the availability of data on non-respiratory organ dysfunction. The number of patients for which AChE and BuChE analysis was available was too small for its findings to be generalised; however, it was modestly useful for drawing comparisons between AChE and BuChE activity post-pralidoxime treatment versus other OP insecticide self-poisonings. More information on efficacy of oximes in phenthoate poisoning would be useful.
Conclusion
As highly hazardous WHO Class II insecticides such as dimethoate and fenthion are banned globally, other OP insecticides will likely replace them and become more commonly used. The evidence reported here suggests that phenthoate is in the mid-range of toxicity of insecticides of WHO hazard class II. However, the case fatality is high compared to poisoning with other Class II insecticide chemical classes that are much less toxic for humans, such as neonicotinoids, pyrethroids and other non-cholinesterase pesticides [Citation29].
Acknowledgements
This study was conducted by the South Asian Clinical Toxicology Research Collaboration within a long running cohort study. The major sources of funding for the pesticide poisoning cohort were provided by the Wellcome Trust (GR063560MA, GR071669MA) and the National Health and Medical Research Council of Australia (268053, 1030069, 1055176). We thank the physicians, directors, and medical and nursing staff of the study hospitals, as well as the South Asian Clinical Toxicology Research Collaboration staff for their assistance and support in running this cohort. We thank the laboratory staff who did the assays. We also thank all the patients who took part in the study. The Centre for Pesticide Suicide Prevention is funded by an Incubator Grant from the Open Philanthropy Project Fund, an advised fund of Silicon Valley Community Foundation, on the recommendation of GiveWell, USA.
Disclosure statement
No potential conflict of interest was reported by the author(s).
Additional information
Funding
References
- Mew EJ, Padmanathan P, Konradsen F, et al. The global burden of fatal self-poisoning with pesticides 2006–15: systematic review. J Affect Disord. 2017;219:93–104.
- Vale A, Lotti M. Organophosphorus and carbamate insecticide poisoning. Handb Clin Neurol. 2015;131:149–168.
- Eddleston M. Insecticides: organic phosphorus compounds and carbamates. In: Nelson L, editors. Goldfrank’s toxicologic emergencies. 11th ed. McGraw-Hill: New York; 2019. p. 1486–1502.
- Gupta RC. CHAPTER 2 - classification and uses of organophosphates and carbamates. In: Gupta RC, editor. Toxicology of organophosphate & carbamate compounds. Burlington: Academic Press; 2006. p. 5–24.
- Eddleston M, Eyer P, Worek F, et al. Differences between organophosphorus insecticides in human self-poisoning: a prospective cohort study. Lancet. 2005;366(9495):1452–1459.
- Wadia R, Bhirud RH, Gulavani AV, et al. Neurological manifestations of three organophosphate poisons. Indian J Med Res. 1977;66:460–468.
- Eddleston M, Buckley NA, Eyer P, et al. Management of acute organophosphorus pesticide poisoning. Lancet. 2008;371(9612):597–607.
- Eddleston M, Worek F, Eyer P, et al. Poisoning with the S-Alkyl organophosphorus insecticides profenofos and prothiofos. QJM. 2009;102:785–792.
- Lamb T, Selvarajah LR, Mohamed F, et al. High lethality and minimal variation after acute self-poisoning with carbamate insecticides in Sri Lanka – implications for global suicide prevention. Clin Toxicol. 2016;54:624–631.
- Eddleston M, Mohamed F, Davies JO, et al. Respiratory failure in acute organophosphorus pesticide self-poisoning. QJM. 2006;99:513–522.
- Jayawardane P, Senanayake N, Buckley NA, et al. Electrophysiological correlates of respiratory failure in acute organophosphate poisoning: evidence for differential roles of muscarinic and nicotinic stimulation. Clin Toxicol (Phila). 2012;50:250–253.
- Buckley NA, Mohamed F, Raubenheimer J, et al. Case fatality of agricultural pesticides after self-poisoning in Sri Lanka: a prospective cohort study. Lancet Global Health. 2021, in press.
- Eddleston M, Dawson A, Karalliedde L, et al. Early management after self-poisoning with an organophosphorus or carbamate pesticide - a treatment protocol for junior doctors. Crit Care. 2004;8:R391–R397.
- World Health Organization. The WHO recommended classification of pesticides by hazard and guidelines to classification: 2019 edition. Geneva: WHO; 2020.
- Alahakoon C, Dassanayake TL, Gawarammana IB, et al. Differences between organophosphates in respiratory failure and lethality with poisoning post the 2011 bans in Sri Lanka. Clinical Toxicology. 2020;58:466–470.
- Rathish D, Agampodi S, Jayasumana C. Acetylcholinesterase inhibitor insecticides related acute poisoning, availability and sales: trends during the post-insecticide-ban period of Anuradhapura, Sri Lanka. Environ Health Prev Med. 2018;23( 1):27.
- Peter JV, Jerobin J, Nair A, et al. Clinical profile and outcome of patients hospitalized with dimethyl and diethyl organophosphate poisoning. Clin Toxicol. 2010;48:916–923.
- Paranjape K, Gowariker V, Krishnamurthy VN, et al. The pesticide encyclopedia. United Kingdom: CABI; 2014. ISBN-13: 9781780640143
- Information NCfB. Phenthoate, CID = 17435. PubChem Database. 2021.
- Kang E-J, Seok S-J, Lee K-H, et al. Factors for determining survival in acute organophosphate poisoning. Korean J Intern Med, 4. 2009;24:362.
- Nara A, Yamada C, Kodama T, et al. Fatal poisoning with both dichlorvos and phenthoate. J Forensic Sci. 2018;63:1928–1931.
- Kawabata S, Hayasaka M, Hayashi H, et al. Phenthoate metabolites in human poisoning. J Toxicol Clin Toxicol. 1994;32:49–60.
- Eddleston M, Eyer P, Worek F, et al. Pralidoxime in acute organophosphorus insecticide poisoning–a randomised controlled trial. PLoS Med. 2009;6:e1000104-e1000104.
- Thiermann H, Szinicz L, Eyer P, et al. Correlation between red blood cell acetylcholinesterase activity and neuromuscular transmission in organophosphate poisoning. Chem-Biol Interact. 2005;157–158:345–347.
- Worek F, Mast U, Kiderlen D, et al. Improved determination of acetylcholinesterase activity in human whole blood. Clin Chim Acta. 1999;288( 1–2):73–90.
- Eddleston M, Gunnell D, von Meyer L, et al. Relationship between blood alcohol concentration on admission and outcome in dimethoate organophosphorus self-poisoning. Brit J Clin Pharmacol. 2009;68( 6):916–919.
- Eddleston M, Eyer P, Worek F, et al. Predicting outcome using butyrylcholinesterase activity in organophosphorus pesticide self-poisoning. QJM. 2008;101( 6):467–474.
- Eyer P, Radtke M, Worek F. Reactions of isodimethoate with human red cell acetylcholinesterase. Biochem Pharmacol. 2008;75:2045–2053.
- Dawson AH, Eddleston M, Senarathna L, et al. Acute human lethal toxicity of agricultural pesticides: a prospective cohort study. PLoS Med. 2010;7:e1000357.
- Peter JV, Jerobin J, Nair A, et al. Is there a relationship between the WHO hazard classification of organophosphate pesticide and outcomes in suicidal human poisoning with commercial organophosphate formulations? Regul Toxicol Pharmacol. 2010;57( 1):99–102.
- Giyanwani PR, Zubair U, Salam O, et al. Respiratory failure following organophosphate poisoning: a literature review. Cureus. 2017;9:e1651.
- Davies JOJ, Eddleston M, Buckley NA. Predicting outcome in organophosphorus poisoning with a poison severity score or Glasgow Coma Scale. Q J Med. 2008;101( 5):371–379.
- Konickx LA, Worek F, Jayamanne S, et al. Reactivation of plasma butyrylcholinesterase by pralidoxime chloride in patients poisoned by WHO class II toxicity organophosphorus insecticides. Toxicol Sci. 2013;136:274–283.
- Eddleston M, Szinicz L, Eyer P, et al. Oximes in acute organophosphorus pesticide poisoning: a systematic review of clinical trials. QJM. 2002;95:275–283.
- Buckley NA, Eddleston M, Li Y, et al. Oximes for acute organophosphate pesticide poisoning. Cochrane Database Syst Rev. 2011;CD005085.
- Lotti M. Clinical toxicology of anticholinesterase agents in humans. In: Krieger RI, Doull J, editors. Handbook of pesticide toxicology Volume 2 Agents. San Diego (CA): Academic Press; 2001. p. 1043–1085.
- Eyer P, Worek F, Thiermann H, et al. Paradox findings may challenge orthodox reasoning in acute organophosphate poisoning. Chem-Biol Interact. 2010;187:270–278.
- Eyer F, Roberts DM, Buckley NA, et al. Extreme variability in the formation of chlorpyrifos oxon (CPO) in patients poisoned by chlorpyrifos (CPF). Biochem Pharmacol. 2009;78:531–537.
- Takade DY, Allsup T, Khasawinah A, et al. Metabolism of O, O-dimethyl S-[α-carboethoxy) benzyl] phosphorodithioate (phenthoate) in the white mouse and house flies. Pest Biochem Physiol. 1976;6( 4):367–376.
- Konradsen F, van der Hoek W, Peiris P. Reaching for the bottle of pesticide – a cry for help. Self-inflicted poisonings in Sri Lanka. Soc Sci Med. 2006;62:1710–1719.