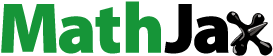
Abstract
Introduction
The accidental ingestion of diluted household descaling products by infants is a phenomenon that poison control centers regularly encounter. Feeding infants with baby milk prepared with water from electric kettles still containing descaler is a common way of exposure. This study aimed to determine the risks related to ingestion of (diluted) descalers by infants.
Methods
pH measurements were performed using acetic acid and three different commercially available electric kettle descalers. The pH of different dilutions was measured in the absence or presence of baby milk powder. In addition, an overview was made of pH values of different electric kettle descalers as given by the product information of the manufacturer. Finally, a simple pharmacokinetic (PK) model was used to predict changes in blood pH in infants after ingestion of acetic acid, which is the most commonly used descaler.
Results
Several commercially available electric kettle descalers have a pH <2. Even after diluting such descalers up to 10 times the pH can remain low. The addition of milk powder increases the pH of descalers containing weaker acids, with a pH >1.5, while descalers with stronger acids and pH <1 show little pH increase after the addition of milk powder. Finally, a simple PBPK model for the ingestion of acetic acid predicted that the ingestion of larger amounts of acetic acid (>1000 mg) by an infant could result in relevant changes in blood pH.
Conclusions
Commercially available electric kettle descaling products may pose a health risk to infants in case of accidental ingestion since the pH of some of these products can be very low, even when diluted 10-times or in the presence of baby milk powder. Oral exposure of infants to the common descaler acetic acid, after accidental preparation of baby milk with cleaning vinegar, will probably not result in serious local effects, but changes in blood pH cannot be excluded when larger amounts of acetic acid are ingested.
Introduction
Accidental exposure of infants to electric kettle descalers, for instance due to the preparation of baby milk with water still containing descaler, is often reported to the Dutch Poisons Information Center (DPIC). Household kettle descalers usually contain weak acids, including both organic and inorganic acids, like acetic acid, citric acid, phosphoric acid or sulfamic acid. The DPIC is consulted >100 times per year about exposures of infants to descalers used to descale kettles and coffee machines. In most of these cases reported to the DPIC vinegar was used as descaler, although descalers containing stronger acids were also involved (Supplementary data). In ∼70% of the vinegar exposures cleaning vinegar was involved, the other cases involved cooking vinegar. Both types of vinegar contain at least 4% vinegar, but cleaning vinegar is usually more concentrated with possible concentrations up to 10%. The UK National Poisons Information Service (NPIS) received 230 inquires relating to kettle descaler exposures of children (<2 years) in 2009–2013, the Czech Toxicological Information Center received 14 inquires in 2009 about descalers diluted in milk drinks (children <3 years) [Citation1,Citation2], indicating that the exposure of infants to kettle descalers is, at least in Europe, a widespread phenomenon. The important question is whether such exposures can result in adverse health effects.
Local effects of acids are well known and described [Citation3]. The severity of tissue damage due to acids depends on pH, the amount and the contact time of the acidic solution with tissue. The severity of injury can also be predicted by the titratable acid/alkaline reserve, i.e. the buffer capacity of an acidic solution [Citation4]. In general, acid solutions with a pH of 2 or lower are considered to be strong corrosives, and exposure to these chemicals can result in severe burns, lesions and coagulation necrosis [Citation5]. This latter effect reduces tissue penetration by acids, usually resulting in less tissue damage compared to caustic agents like alkaline chemicals [Citation3]. Ingestion of acids may induce burns throughout the gastrointestinal tract, mainly esophageal, gastric and/or duodenal lesions. Eventually, esophageal stricture formation may occur due to scarring. In case of oral exposure to weak acids as found in household descaling products, it is not clear whether local tissue damage is likely to occur, especially when descalers are diluted and/or supplemented with baby milk powder.
Moreover, it is difficult to predict the occurrence of systemic effects after such exposures. In case of a functional intestinal barrier, the rate of acid absorption, metabolism and excretion determines the acid load. At a steady state, blood pH is tightly regulated and buffered, since protein function is strongly dependent on pH. Mechanisms for pH auto-regulation include the carbonic acid–bicarbonate buffer system, renal bicarbonate homeostasis, renal excretion of ammonium ions, respiratory control of the partial pressure of carbon dioxide and buffering by bone [Citation6,Citation7]. Systemic effects may occur when acids enter the blood circulation after ingestion of large amounts. Damage to the gastrointestinal barrier promotes the absorption of larger amounts of acids. In some cases, the resulting acidemia (lowering of blood pH) cannot be corrected sufficiently and symptoms like hemolysis, thrombocytopenia, clotting disorders, changes in respiratory rate, Kussmaul respiration, kidney disorders, nausea and vomiting can be observed [Citation8].
This study was conducted to gain more insight into the risk of developing local effects after accidental ingestion of weak acids as found in descaling household products by infants. This question was addressed by analyzing the pH of commercially available electric kettle descalers, both in undiluted and diluted form, and in the absence and presence of milk powder. Moreover, the possibility of the development of systemic effecters after ingestion of acetic acid was further addressed using simple PK modeling.
Materials and methods
pH of descalers – product information
We made an inventory of safety data sheets of 30 different descalers available in The Netherlands (in supermarkets and online), including the acidic component of the product and the pH as indicated by the manufacturers. Only descalers used for descaling kitchen equipment (electric kettles, coffee machines) were selected.
pH of descalers – measurements
We performed pH measurements on three descalers and cleaning vinegar. Different solutions of descalers were prepared, in the presence or absence of milk powder. For each product the following dilutions were prepared using tap water: 1:2, 1:5 and 1:10. The volume of each sample was 30 mL. The pH was measured using a Microprocessor Bench-top pH Meter (Hanna Instruments HI8520) and a glass body, refillable, double junction pH electrode (Hanna Instruments HI1131). After measuring each sample, 3.33 grams of milk powder (standard dose recommended by the manufacturer, Nutrilon Standard 1, Nutricia) was added, vortexed for 1 min and measured again. The pH was measured at room temperature (20 °C). The pH of the undiluted descaler sample containing milk powder was measured at 37 °C as well. Based on the measured pH values actual acid concentrations in the undiluted and diluted sample were calculated using weak acid-base equilibria equations:
(1)
(1)
(2)
(2)
Calculation of blood pH after ingestion of acetic acid by infants
The expected blood pH of infants after ingestion of acetic acid was calculated, using several assumptions: the bioavailability of acetic acid (AA) was assumed to be 100%. This value was higher compared to in vivo data of Sugiyama et al. [Citation9]. A maximal bioavailability was used in the approach since there were no data available for the bioavailability of acetic acid in infants. The partial pressure of carbon dioxide (pCO2) was assumed to be 40 mmHg (and stable during acid absorption).
We used the Simcyp® simulator [Citation10] to predict the increase in plasma concentration of acetic acid after ingestion of different oral doses in infants aged 0.06 year (22 weeks) and ∼3.7 kg body weight. Additional methodological information is given in the Supplementary data. The acquired model was used to simulate a plasma concentration–time course of acetic acid for doses ranging between 250 mg and 4000 mg (corresponding to the ingestion of a 100 mL 0.25–4% (m/V) acetic acid solution). The obtained maximal plasma concentrations (Cmax) were further used in the Henderson Hasselbalch equation in order to calculate the expected plasma pH at Cmax.
(3)
(3)
The expected pH was determined by calculating the concentration of HCO3− as the difference between the normal concentration of HCO3− (24 mM) and the plasma concentration of absorbed acetic acid. For hydroxyacetic acid, phosphoric acid and sulfamic acid no in vivo pharmacokinetic data, as was found for acetic acid, were available. For instance, there were no data available on the course of acid concentrations in blood plasma after ingestion of the respective acids. Such data are required in order to estimate the parameters for absorption and elimination.
Results
pH of descalers
The characteristics of 30 descalers available in supermarkets or online in the Netherlands are shown in . According to the manufacturer most of these descalers have a pH lower than 2. Sulfamic acid, citric acid and phosphoric acid were the acids most commonly used, in 13, 8 and 7 of 30 descalers, respectively. For further in vitro analyses we used cleaning vinegar, and three electric kettle descaling products which can be bought in supermarkets or on the internet (further indicated as descaler 1, 2 and 3, see ).
Table 1. Commercially available descalers and their acidic components and pH as indicated by the manufacturer.
Table 2. Product information of the electric kettle descalers used for pH measurements.
As shown in , cleaning vinegar has a pH >2, even in undiluted form, and the pH clearly increased after addition of milk powder. Based on the pH it was calculated that the concentration acetic acid in this product was 5.7% (m/V), although the product sheet indicated a concentration of 8%. Descaler 1 is largely composed of hydroxyacetic acid, with the addition of some D-gluconic acid. Both acids are stronger than acetic acid and the undiluted descaler 1 has a pH <2. Descaler 1 also showed an increase in pH following addition of milk powder. Descaler 2, containing the even stronger phosphoric acid, has a low pH (<2, even in a 1:10 dilution), and the effect of milk powder on the pH was minimal. Based on the pH, it was estimated that the concentration of phosphoric acid in this product was 22% (m/V), which was higher than the concentration given by the manufacturer (7–10%). The last descaler, descaler 3, contains the strongest acid, sulfamic acid, and both undiluted and diluted solutions have a pH ≪ 2. The product information stated that the pH was 1.5, while pH measurements indicated a pH of 0.41. This low pH is in accordance with the amount of sulfamic acid indicated by the manufacturer (15%). The addition of milk powder marginally altered the pH. In all descalers the increase in pH with increasing dilution followed the theoretically determined pH, using weak acid-base equilibria with the Ka values of involved acids, indicating that no buffer capacity was present in the descalers. Also, no temperature effects on pH were observed in undiluted samples with milk powder.
Figure 1. The effect of dilution and addition of milk powder on the pH of different descalers. Cleaning vinegar, descaler 1, 2 and 3 contained acetic acid, hydroxyacetic acid (supplemented with D-gluconic acid), phosphoric acid and sulfamic acid, respectively. Black circles represent solutions without milk powder, black triangles represent solutions containing milk powder (3.33 g/30 mL), all measured at 20 °C. Grey triangles represent calculated pH values based on acetic acid, hydroxyacetic acid, phosphoric acid and sulfamic acid concentrations (undiluted) of respectively 5.7%, 35%, 22% and 18% (m/V). ▲* Represents the measurement of the undiluted sample with milk powder at 37 °C. The y-axis represents the measured pH, the x-axis, in –log10 transformation, represents the dilution factor.
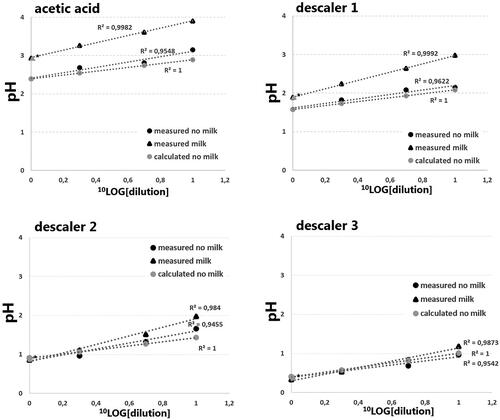
Calculation of blood pH after ingestion of acetic acid by infants
We used a minimal PBPK model to estimate the pharmacokinetics of acetic acid after ingestion by infants (). The obtained maximal plasma concentrations of acetic acid were used to calculate the blood pH after ingestion of acetic acid (). After the intake of 1000 mg acetic acid, corresponding to the ingestion of 100 mL 1% acetic acid, the calculated maximal plasma concentration is 3161 µmol/L (189 mg/L). At this blood concentration the pH is predicted to be 7.33, which is regarded as below normal.
Figure 2. Estimation of blood pH after the ingestion of acetic acid by an infant. (A) The modeled pharmacokinetics of different amounts of ingested acetic acid, using a minimal physiological based pharmacokinetic (PBPK) model (see inlay, ka= 20 (1/h), Vss = 0.5 (L/kg), CLpo = 84.76 (L/h). The y-axis represents the plasma concentration, the x-axis represents the time after ingestion. (B) The calculated blood pH after ingestion of different amounts of acetic acid, using the maximum concentrations of acetic acid depicted in . The y-axis represents the calculated blood pH, the x-axis represents the amount of ingested acetic acid.
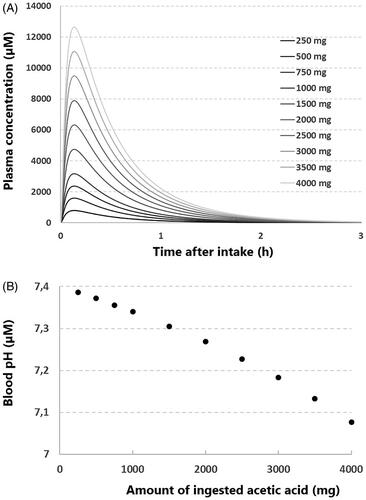
Conclusions and discussion
The main purpose of this work was to determine if ingestion of electric kettle descalers by infants may pose a health risk. Analysis of the pH of different descalers was performed as measure for caustic risk. Moreover, since most of the cases of descaler ingestion by infants reported to the DPIC involved cleaning vinegar, an in silico approach was used to determine the effects of acetic acid ingestion on blood pH in infants.
Measurements of the pH of different descalers showed that oral exposure to these type of products can result in caustic effects. First, several commercially available electric kettle descalers have pH values lower than 2. In general descaling products are diluted before use, which increases the pH of the acidic solution. The manufacturers of the tested descaling products recommended dilutions of 1:10. In case of descalers containing stronger acids like sulfamic acid or phosphoric acid, a 1:10 dilution can still result in a solution with a pH <2, as is shown by the presented measurements. Often infants are exposed to diluted electric kettle descalers mixed with baby milk powder, which largely consists of whey proteins and casein. Due to the presence of ionizable amino acid side groups it was expected that milk powder would have a buffer capacity. Indeed for acetic acid and hydroxyacetic acid there was a clear increase in pH upon addition of milk powder. It was calculated that 50–80% of the protons released by the acids were neutralized by the milk powder (see Supplementary data). The neutralizing capacity increased with increasing dilution and hence increasing pH. For phosphoric acid and sulfamic acid the buffering effects of milk powder were considerable less, with respectively 50% and 40% of released protons being neutralized by milk powder, but only at the 1:10 dilution (see Supplementary data). In the undiluted samples no effects of milk powder could be observed. Moreover, it is likely that a low-pH descaler, 1:10 diluted and supplemented with milk powder can still have a pH <2 and may pose a caustic risk, causing esophageal or gastric damage when ingested by infants. On the other hand, exposures to (diluted or undiluted) cleaning vinegar probably has a low risk for caustic damage, especially when milk powder is present. Information about the composition of descalers to which infants are exposed may help caregivers to estimate the possible severity of local effects, although it should be taken into account that, as our results show, the composition as shown on packages may not always reflect the actual composition.
Since infants are predominantly exposed to cleaning vinegar used as descaler, the change in blood pH in infants after ingestion of low-concentrated acetic acid solution was estimated. In addition, in vivo pharmacokinetic data was available for acetic acid. The results of the used model are in line with in vivo observations. For instance, Richard et al. [Citation11] showed that the clearance of acetate from plasma is very fast (2.31 L/min or 32.4 mL/min/kg), which is in accordance with in vivo data of Sugiyama et al. [Citation9]. The used minimal PBPK model, combined with the Henderson Hasselbalch equation, suggests that relevant changes in blood pH after ingestion of 1000 mg of acetic acid or more are possible in infants and metabolic acidosis cannot be excluded. Limitations of our analyses are (1) that the estimation of kinetic parameters are based on a limited set of data, (2) that we used only a minimal PBPK model for the extrapolations to larger doses and to children, (3) the assumption that the bioavailability of acetic acid from gut uptake is 100% (the Sugiyama data suggest a bioavailability of ∼60%) and (4) possible effects of milk powder were not included in the analysis. The presence of milk powder probably results in less absorption of acetic acid, since a part of the acetic acid has been converted to acetate due to the buffer capacity of milk powder. Nevertheless, in our opinion a better and safer approximation of the kinetics of ingested acetic acid in infants was not possible. There is a lack of case reports of small children developing metabolic acidosis after the ingestions of low-concentrated acetic acids, suggesting that the in silico approach presented here may be too stringent.
To conclude, the ingestion of low-concentrated acetic acid, as found in cleaning vinegar, by infants probably does not pose a significant risk for local tissue damage, especially when mixed with milk powder, but relevant changes in blood pH cannot be excluded when larger amounts of acetic acid are ingested. Moreover, there are several electric kettle descalers available on the market that contain stronger acids, which may result in more serious health problems after ingestion by infants. During the anamnesis of an infant orally exposed to kettle descalers, the composition of the acidic solution should be taken into account, in order to be able to estimate possible health risks. In case of descalers containing stronger acids, further clinical examination is recommended when clinical symptoms (signs of pain, swallowing difficulties, rapid breathing) are present.
Supplemental Material
Download MS Word (257.2 KB)Disclosure statement
Arjen Koppen, Claudine Hunault, Regina van Kleef, Agnes van Velzen, Remco Westerink, Irma de Vries en Dylan de Lange declare that they have no conflict of interest.
References
- Alldridge G, Jones S, Vale JA, et al. Abstract: kettle descalers: a brewing issue. Clin Toxicol. 2015;53:35.
- Mrazová KN, Pelclová D. Consequences of ingestions of potentially corrosive cleaning products, one-year follow-up. Int J Electrochem Sci. 2012;7:1734–1748.
- Chirica M, Bonavina L, Kelly MD, et al. Caustic ingestion. Lancet. 2017;389(10083):2041–2052.
- Hoffman RS, Howland MA, Kamerow HN, et al. Comparison of titratable acid/alkaline reserve and pH in potentially caustic household products. J Toxicol Clin Toxicol. 1989;27(4-5):241–246.
- Caravati E. Acids. In: Dart R, editor. Clinical toxicology. 3rd ed. Philadelphia: Lippincott Williams and Wilkins; 2004. p. 1294–1303.
- Hamm LL, Nakhoul N, Hering-Smith KS. Acid-base homeostasis. Clin J Am Soc Nephrol. 2015;10(12):2232–2242.
- Lemann J, Jr., Bushinsky DA, Hamm LL. Bone buffering of acid and base in humans. Am J Physiol Renal Physiol. 2003;285(5):F811–F832.
- Berend K, de Vries AP, Gans RO. Physiological approach to assessment of acid-base disturbances. N Engl J Med. 2014;371(15):1434–1445.
- Sugiyama S, Fushimi T, Kishi M, et al. Bioavailability of acetate from two vinegar supplements: capsule and drink. J Nutr Sci Vitaminol. 2010;56(4):266–269.
- Jamei M, Marciniak S, Feng K, et al. The Simcyp population-based ADME simulator. Exp Opin Drug Metab Toxicol. 2009;5(2):211–223.
- Richards RH, Vreman HJ, Zager P, et al. Acetate metabolism in normal human subjects. Am J Kidney Dis. 1982;2(1):47–57.