Abstract
Introduction
Antivenom is first line treatment for snake envenomation worldwide, despite few placebo controlled clinical trials demonstrating effectiveness. We aimed to investigate whether early antivenom in red-bellied black snake (Pseudechis porphyriacus) bites would prevent systemic myotoxicity.
Methods
We undertook a multicentre randomized placebo-controlled trial of antivenom for red-bellied black snake bites with patients recruited from the Australian Snakebite Project (July 2014 to June 2020). In addition, we report all patients with red-bellied black snake bites during the same period, comparing the same outcomes. Patients over 2 years of age with definite red-bellied black snake bites and early systemic effects were randomized to receive 50 per cent glucose (placebo) or tiger snake antivenom within 6 hours post-bite, or in the cohort group received antivenom determined by the treating clinician. The primary outcome was the proportion of patients with myotoxicity (peak creatine kinase activity >1,000 U/L). Secondary outcomes were: area under the curve of total creatine kinase elevation over 48 hours, presence of venom post-antivenom, and adverse reactions. We analyzed both the randomized control trial patients and the combination of randomized control trial and cohort patients.
Results
Fifteen patients were recruited to the randomized controlled trial, and a cohort of 68 patients who were not randomized were included in the analysis. After treatment, two of seven patients given placebo had a peak creatine kinase activity >1,000 U/L versus none of the eight given antivenom (difference in favour of antivenom; 29 per cent; 95 per cent confidence interval:-18 per cent to +70 per cent; P = 0.2). The median area under the curve of total creatine kinase elevation over 48 hours in patients given placebo was 0 U/L.h (interquartile range: 0–124 U/L.h), which was not significantly different to those given antivenom: 197 U/L*h (interquartile range: 0–66,353 U/L*h; P = 0.26). Venom was not detected post-antivenom in six patients with measured venom concentrations given antivenom. Two patients given antivenom had immediate hypersensitivity reactions, one severe anaphylaxis, and another had serum sickness. Combining randomized and not randomized patients, three of 36 (8 per cent) administered antivenom less than 6 hours post-bite had a peak creatine kinase activity > 1,000 U/L versus 17/47 (36 per cent) patients not receiving antivenom less than 6 hours post-bite (difference in favour of antivenom 29 per cent; 95 per cent confidence interval: 8 per cent to 44 per cent; P < 0.004). Overall, 13/36 (36 per cent) patients administered antivenom within 6 hours had hypersensitivity reactions, six severe anaphylaxis (17 per cent).
Discussion
We found that early antivenom was effective in red-bellied black snake bites, and only three patients need to be given antivenom within 6 hours to prevent myotoxicity in one (number needed to treat = 3). However, one in three patients administered antivenom developed a hypersensitivity reaction, and one in six had severe anaphylaxis. The major limitation of this study was the small number of patients recruited to the randomized controlled trial.
Conclusion
Administration of antivenom in red-bellied black snake envenomation within 6 hours post-bite appeared to decrease the proportion of patients with myotoxicity, but a third of patients had adverse reactions.
Introduction
Snake envenomation is a major health issue in the rural tropics [Citation1]. It also remains an uncommon serious medical condition in many parts of the developed world, such as Australia [Citation2]. The major envenomation syndromes from Australian snakebites are venom-induced consumption coagulopathy [Citation3], myotoxicity [Citation4], neurotoxicity and thrombotic microangiopathy [Citation5]. Systemic myotoxicity occurs less commonly than other envenomation syndromes but, in severe cases, is life-threatening [Citation2] and difficult to treat [Citation4]. Myotoxicity is most commonly seen with black snake (Pseudechis spp.) and tiger snake (Notechis scutatus) bites [Citation4,Citation6].
Antivenom is the main treatment for snake envenomation, but there is limited evidence that antivenom is clinically effective for snake envenomation [Citation7,Citation8] despite numerous pre-clinical studies demonstrating that antivenom binds and neutralizes toxins in vitro. However, there is increasing evidence that early antivenom will prevent myotoxicity [Citation9,Citation10] and neurotoxicity [Citation11] in Australasian snakebites and venom-induced consumption coagulopathy in Viperidae bites [Citation12,Citation13]. Conversely, antivenom does not appear to be effective in neutralizing or preventing other envenomation effects, such as venom-induced consumption coagulopathy in Australasian snakebites [Citation14]. Therefore, it is important to assess antivenom effectiveness for specific clinical effects or clinical syndromes.
There are five different monovalent antivenoms available in Australia for medically important snakes. For all but one group of medically important snakes, antivenom is the standard of care because of the life-threatening nature of systemic envenomation [Citation2]. The one exception is red-bellied black snakes (Pseudechis porphyriacus), for which systemic envenomation is not life-threatening [Citation10,Citation15], and historically, there has been clinical equipoise in regards to the administration of antivenom due to the risk of adverse reactions and unclear benefit. For example, the Australian Medicines Handbook only recommends that antivenom be considered in the most severe cases (including very young children or elderly with pre-existing significant medical problems) because of the low fatality potential with this snakebite [Citation16]. Thus, it was regarded as ethical to randomize most patients with red-bellied black snake bite to antivenom or placebo. In addition, tiger snake antivenom is recommended for red-bellied black snake envenomation. This is a historical clinical practice based on recommendations from the antivenom manufacturer.
We had a unique opportunity to undertake a placebo-randomized controlled trial to determine the effectiveness of early antivenom for the prevention of snake bite myotoxicity. We aimed to investigate whether early antivenom in red-bellied black snake envenomation would prevent systemic envenomation, including myotoxicity, and monitor the frequency of allergic reactions, including anaphylaxis.
Methods
We undertook a placebo controlled randomized trial of tiger snake antivenom in patients bitten by a red-bellied black snake with systemic envenomation. The primary outcome was myotoxicity, which was defined as a peak creatine kinase activity >1,000 U/L. In addition, we report all patients with red-bellied black snake bites during the same study period, comparing the same outcomes. The study was approved by the Hunter Area Health Human Research Ethics Committees to cover all five hospital sites. The trial was registered with the Australian New Zealand Clinical Trials Registry, http://www.anzctr.org.au/, ACTRN12611000588998.
This randomized controlled trial was nested within the Australian Snakebite Project, similar to a previous randomized controlled trial of fresh frozen plasma in snakebite coagulopathy [Citation17]. The Australian Snakebite Project commenced in 2004 as a large prospective cohort study with the aim to use serial quantitative venom and antivenom concentrations in snake envenomation to assess:
the efficacy of antivenom [Citation18];
antivenom dose [Citation2,Citation19,Citation20];
first aid - pressure immobilization;
pharmacokinetics-pharmacodynamic models of envenomation [Citation21,Citation22]; and use this information to undertake controlled trials of treatment [Citation17] and develop evidence-based guidelines [Citation23].
In addition to the predefined aims, clinical outcomes and snake envenomation syndromes were developed and clearly defined during the early part of the Australian Snakebite Project [Citation2,Citation24]. The design of the Australian Snakebite Project then allowed the concurrent prospective collection of red-bellied black snake bites that could not be recruited to the randomized controlled trial because they were at hospitals with only ethical approval for the Australian Snakebite Project and not the clinical trial. Identical infrastructure was used to recruit both trial patients and cohort study participants.
Study patients and recruitment
For the randomized controlled trial, patients with a red-bellied black snake bite were identified by emergency medicine staff or study investigators from five emergency departments in the Hunter Area in Eastern Australia between July 2014 and June 2020 as part of the Australian Snakebite Project [Citation2]. For the cohort study, red-bellied black snake bite patients were identified from all other hospitals in Australia participating in the Australian Snakebite Project. The treating doctor then contacted the study investigators via a national toll-free number to determine if the patient was eligible for the study or would be included in the cohort study.
Patients (> 2 years of age) were eligible for the randomized controlled trial and cohort study if they had a definite bite by a red-bellied bellied black snake and they had evidence of systemic envenomation within 6 h of the bite. Identification was based on expert identification of the snake, identification from a photograph or characteristic description of the snake (black snake with red sides and cream/yellow ventral surface). Evidence of systemic envenomation included any of the following occurring within 6 h post-bite:
non-specific systemic effects including two of nausea/vomiting, headache, abdominal pain, diarrhoea;
anticoagulant coagulopathy defined as an activated partial thromboplastin time greater than the normal range; and
significant local tissue injury with swelling, bruising and erythema >5 cm diameter.
Treatment protocol
The randomized controlled trial or cohort study was explained to the patient, and then written consent was obtained from the patient or their parents/guardians for children aged less than 17 years. For the randomized controlled trial, the treating doctor then re-contacted the investigators to randomize the patient to antivenom or placebo, which then needed to be administered within 6 h of the bite. The patient was then moved to an acute observation area with cardiac monitoring, pulse oximetry and automated blood pressure measurement. Epinephrine was available for the treatment of anaphylaxis.
The pharmacy department at Calvary Mater Newcastle, in conjunction with Stenlake Compounding Chemist (Bondi, NSW), produced pre-packed kits for the trial. Each kit contained either one vial of tiger snake antivenom (A) or 50% glucose (B). Glucose 50% was chosen as a placebo because it was more viscous and had a slight yellow tinge, similar to the appearance of the antivenom, unlike normal saline. Both tiger snake antivenom vials and identical (empty) vials were purchased from CSL Ltd. (now Sequiris). The empty vials were filled with an equivalent volume of glucose 50% (placebo). The pharmacy then packed kits and labelled these A or B, using a block randomization schedule.
The allocation sequences were based on block randomization with random block sizes of two (e.g., AB, AA, BB etc.) or four (AABB, ABAB, etc.). These were then organized into packs of four treatments for each hospital site. Each site had one pack containing four treatment kits. The use of variable block sizes of two and four meant that each pack of four may contain a mixture of blocks of two or half-blocks of four making it impossible for the treating clinicians to predict the last kit in each pack. The allocation to each treatment pack was then done based on a pre-randomized list of all packs for all study sites kept by the on-call investigators. They provided the next study code to the treating doctor/nurse to identify the treatment kit to be used. Allocation of each study code/treatment pack to active or placebo was only known by the pharmacy at Calvary Mater Newcastle.
The study vial (tiger snake antivenom or glucose 50%) was administered in normal saline 200 mL over 20 min. No unblinded tiger snake antivenom could be given within 6 h of the bite. Other supportive treatments such as anti-emetics, intravenous fluids and analgesia could be given to the patient at the discretion of the treating doctor. If the patient had ongoing symptoms after 6 h, then antivenom could be administered to the patient by the treating doctor.
For the cohort study treatment with antivenom was determined by the treating doctor and could be at any time after the bite.
Data collection
The clinical trial and cohort study used infrastructure already in place for the Australian Snakebite Project [Citation2], except the datasheets were modified to include the primary and secondary outcomes for this trial. Datasheets were filled out by the treating clinician or local investigator and faxed back to the chief investigator. All data were then entered into the Australian Snakebite Project database in Microsoft AccessTM. Predefined data collection included patient demographics, information on the bite and bite time, timed clinical observations [non-specific systemic effects (nausea/vomiting, abdominal pain, headache, diarrhoea), local and general muscle pain and myalgia, smell sensation, bite site effects], timed laboratory investigations (creatine kinase activity, activated partial thromboplastin time, white cell count), adverse effects from antivenom/placebo, intensive care admission and length of stay. Creatine kinase activity was measured every 6 h in patients recruited to the clinical trial as part of the protocol. For patients in the cohort, serial measurements of creatine kinase activity were recommended for all patients as per clinical guidelines but were ultimately determined by the treating clinician.
Serum samples were collected for each patient before and after antivenom/placebo administration, centrifuged at 2,000 g for 10 min and stored at −80 °C for the later measurement of venom concentrations. Venom was measured in serum using a previously described venom-specific enzyme immunoassay [Citation10,Citation25].
Outcomes
The primary outcome was the proportion of patients with myotoxicity defined as a peak creatine kinase activity >1,000 U/L. The 1,000 U/L cut-off for the creatine kinase activity is based on the well-established definition of systemic myotoxicity in snake bites [Citation2,Citation4,Citation24]. Secondary outcomes were the area under the curve (AUC) of the total creatine kinase activity elevation over the first 48 h (Creatine kinase activity-AUC0-48h; a measure of cumulative muscle injury), presence of free circulating venom 1 h after antivenom [Citation18], early systemic hypersensitivity reactions and serum sickness (three or more of the following characteristic clinical features 7–21 days after antivenom administration - fever, erythematous rash, urticaria, myalgia/arthralgia, headache, malaise and nausea/vomiting) [Citation26]. Systemic hypersensitivity reactions were graded according to the Brown grading system [Citation27] and anaphylaxis was defined according to the National Institute of Allergy and Infectious Diseases/Food Allergy and Anaphylaxis Network (NIAID-FAAN) consensus criteria [Citation28].
Data analysis
The sample size for the clinical trial was based on patients with red-bellied black snake bites recruited to the Australian Snakebite Project, in which a quarter of envenomated patients developed a peak creatine kinase activity > 1,000 U/L [Citation10]. The aim was to prevent myotoxicity in all cases based on the 100% efficacy of antivenom in binding venom. Therefore, a decrease of 23% from 25% to 2% would be clinically significant and would be sufficient to warrant the use of antivenom administration as the standard of care. In order to detect whether antivenom decreases the proportion of patients developing myotoxicity from 25% to 2%, with a 1:1 ratio of antivenom to placebo with a significance level (alpha) of 0.01 and a power of 80%, a minimum of 38 treatment patients and 38 control patients were to be recruited (that is a total of 76 patients). We aimed to recruit 80 patients over five snake bite seasons (five years).
Unfortunately, recruitment rates were much lower than expected, and it was not possible to include the intended 10 to 15 hospitals in the study due to the complexity of maintaining four treatment kits at each site. Even at the five study sites, consent and recruitment rates were low, with no recruitment at one site. The randomized controlled trial was stopped after six years as there was no prospect of reaching the planned sample size, and grant funding for the study had finished. The decision was made at this time to include the cohort patients with red-bellied black snake envenomation, who were recruited using the same infrastructure and only differed from the trial patients because they were not randomized to antivenom or placebo. We then conducted a further analysis, including the randomized controlled trial patients combined with the cohort patients prospectively recruited to the Australian Snakebite Project.
All the data were extracted from the Australian Snakebite Project database at the completion of the study by SJ, who was not involved in recruiting or treating patients and was blinded to the treatment. Cases were only identifiable by study codes. Classification of primary and secondary outcomes was done by GI, who then undertook the analysis.
Statistical analysis
The dichotomous primary outcome was analyzed by intention to treat using Fisher’s exact test comparing those administered antivenom to those receiving placebo. For secondary outcomes appropriate statistical tests were used for continuous outcomes, depending on whether the data was parametric or non-parametric. The creatine kinase activity-AUC0-48h was designed to only count the extent of creatine kinase activity elevation above 200 U/L. Only patients with more than two creatine kinase activity measurements were included for the creatine kinase activity -AUC0-48h. We used the trapezoidal method to estimate the AUC of all measured creatine kinase activity samples for each individual as follows. Time = 0 creatine kinase activity values were set to 200. We added all trapezoids for each individual with a sum of two creatine kinase activity values > 400 U/L (that is an average creatine kinase activity > 200 U/L). The area of other trapezoids were calculated as 0.5*(creatine kinase activity 1+ creatine kinase activity 2-400)*(time 2-time 1). For trapezoids that crossed the 48 h, value Time 2 was set to 48 h. For individuals who did not have creatine kinase activity measurements over 48 h the last measured creatine kinase activity was carried forward to be the 48 h value (this was mostly people with minor or no elevation in creatine kinase activity values). All analyses and graphs were undertaken in Microsoft Excel 365 and GraphPad Prism version 10.1.1 for Windows (GraphPad Software, San Diego, California, USA).
Results
There were 894 patients recruited to the Australian Snakebite Project during the study period: 790 were bites from other snakes or non-envenomated bites, 19 were non-envenomated red-bellied black snake bites, one had no creatine kinase activity measured and one self-discharged. Of the remaining 83 patients, 15 presented to hospitals recruiting for the clinical trial (). There were no differences in baseline characteristics between patients randomized to placebo or antivenom or between patients recruited to the cohort given antivenom within 6 h or not ().
Figure 1. Flow diagram of patients recruited to the randomized controlled trial and patients not randomized and included in the second analysis.
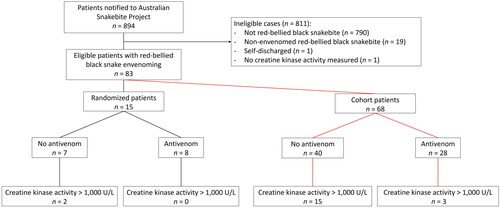
Table 1. Demographics for patients recruited to the randomized controlled trial and cohort patients not recruited to the randomized controlled trial comparing those administered antivenom within 6 h and those not administered antivenom within 6 h.
After treatment, two of seven patients from the placebo arm had a peak creatine kinase activity > 1,000 U/L versus none of eight from the antivenom arm (difference in favour of antivenom; 29%; 95% CI: −18% to +70%; P = 0.2). The median creatine kinase activity-AUC0-48h in patients given placebo was 0 U/L.h (IQR: 0 to 124 U/L*h), compared to those given antivenom of 197 U/L*h (IQR: 0 to 66,353 U/L*h; P = 0.26; , and ). Nine patients had venom concentrations measured, with three patients from the placebo arm having detectable venom post-administration compared to none of six patients given antivenom. Two patients (25%) in the antivenom arm had immediate hypersensitivity reactions, one being severe anaphylaxis; another patient had serum sickness (). There was no difference in length of stay between patients in the placebo and antivenom arms.
Figure 2. Scatter plot of the creatine kinase activity [A] and creatine kinase activity-area under the curve0-48h [B] comparing patients administered antivenom within 6 h and those not administered antivenom within 6 h, for randomized patients (filled blue circles), non-randomized patients (filled green circles) and patients given late antivenom > 6 h (filled red circles).
![Figure 2. Scatter plot of the creatine kinase activity [A] and creatine kinase activity-area under the curve0-48h [B] comparing patients administered antivenom within 6 h and those not administered antivenom within 6 h, for randomized patients (filled blue circles), non-randomized patients (filled green circles) and patients given late antivenom > 6 h (filled red circles).](/cms/asset/05003f63-ca3e-4242-8ae2-84bad9014ee3/ictx_a_2367677_f0002_c.jpg)
Figure 3. Plot of creatine kinase activity-area under the curve 0-48h versus time to antivenom for patients given antivenom (open blue circles), patients given late antivenom after an abnormal creatine kinase activity (filled red circles) and patients not given antivenom (open black circles).
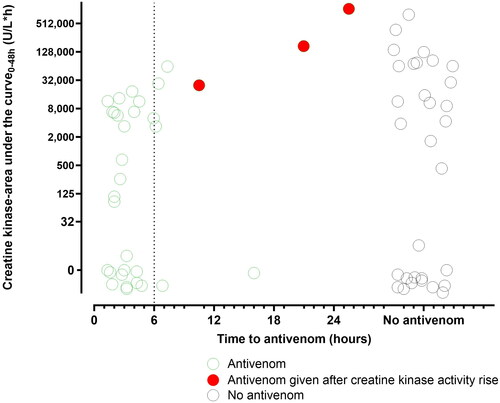
Table 2. Outcome for patients administered antivenom and those not given antivenom (within 6 h) for the randomized controlled trial and the cohort patients not recruited to the randomized controlled trial.
Of 68 patients not randomized, 15/40 (38%) patients who did not receive antivenom or received it more than 6 h post-bite had a creatine kinase activity >1,000 U/L, compared to 3/28 patients who received antivenom within 6 h (). Combining randomized and not randomized patients, three of 36 (8%) receiving antivenom post-bite <6 h had a peak creatine kinase activity >1,000 U/L versus 17/47 (36%) patients not receiving antivenom <6 h (difference in favour of antivenom; 29%; 95% CI: 8% to 44%; P < 0.004). The median creatine kinase activity-AUC0-48h in patients given antivenom was 96 U/L*h (IQR: 0 to 5,914 U/L*h), statistically significantly lower than for those not given antivenom: 4,048 U/L*h (IQR: 0 to 64,443 U/L*h; P = 0.038 []; details broken down by randomized controlled trial versus non-randomized controlled trial shown in ). The median peak creatine kinase activity was non-significantly higher for patients not receiving antivenom (or > 6 h post-bite) compared to those receiving antivenom < 6 h post-bite for both patients in the randomized controlled trial and cohort (, ). Supplementary Figure 1 compares the serial creatine kinase activity in patients receiving antivenom < 6 h post-bite and those not receiving antivenom < 6 h.
Of the non-randomized patients, seven of the eight patients with an abnormal activated partial thromboplastin time pre-antivenom had a normal activated partial thromboplastin time immediately post-antivenom. A repeat activated partial thromboplastin time was not done in the eighth. Eleven patients (39%) administered antivenom had an immediate hypersensitivity reaction, and five of these had severe anaphylaxis. Overall, 13/36 (36%) of the patients administered antivenom within 6h had hypersensitivity reactions, six being severe anaphylaxis (17%). There were no deaths.
Three patients given antivenom < 6 h post-bite in the non-randomized patients developed a creatine kinase activity >1,000 U/L (). The first was a 16-year-old male with severe envenomation after multiple bites who developed severe rhabdomyolysis despite early antivenom. Free venom was detected post-antivenom, suggesting that, in this case, one vial of tiger snake antivenom was insufficient () [Citation29]. The other two patients only had peak creatine kinase activities of 1,221 U/L and 1,228 U/L at 72 h post-bite. There were eight patients administered antivenom > 6 h post-bite (five between 6 h and 12 h, and three > 12 h post-bite), and five of eight (63%) had myotoxicity (). In addition, three of these eight patients had immediate hypersensitivity reactions, one with severe anaphylaxis.
Discussion
We found in a small randomized controlled trial that the administration of antivenom within 6 h of the bite reduced the number of patients with myotoxicity (creatine kinase activity > 1,000 U/L), and no patients administered antivenom had venom detected post-antivenom. We combined the randomized controlled trial patients with a larger cohort of patients administered antivenom within 6 h and compared those given antivenom within 6 h to those not given antivenom within 6 h and found a statistically significant reduction in patients with myotoxicity. There were no deaths, severe morbidity was uncommon, and we did not have sufficient numbers to examine differences in the rare severe consequences of myotoxicity (ventilation, electrolyte abnormalities and acute kidney injury). Twenty-five per cent of patients in the randomized controlled trial and 39% of the patients in the observational cohort had immediate hypersensitivity reactions ().
Previous studies have demonstrated that early antivenom prevents myotoxicity in black snake bites, including mulga (Pseudechis austalis) snake bites within 2 h [Citation9] and red-bellied black snakes within 6 h [Citation10]. Other studies have similarly demonstrated that antivenom within 2 to 4 h prevents myotoxicity in taipan envenomation [Citation11,Citation30]. When combining clinical trial and cohort patients, we found a statistically significant benefit of antivenom within 6 h in preventing myotoxicity (proportion of patients with a creatine kinase activity > 1,000 U/L) for red-bellied black snake bites () similar to a previous study [Citation10]. This differs from other snakes and toxidromes, in which antivenom appears to be beneficial only within 2 to 3 h [Citation9,Citation11,Citation30].
The study demonstrates that early antivenom appears effective in red-bellied black snake bites, and only three patients need to be administered antivenom within 6 h to prevent myotoxicity in one (number needed to treat = 3). However, one in three patients administered antivenom developed a hypersensitivity reaction, and one in six had severe anaphylaxis. In practical terms, this means that antivenom administration on average will prevent moderate morbidity in red-bellied black snake bites, with a significant risk of immediate hypersensitivity reactions and anaphylaxis. There were a number of severe cases with rhabdomyolysis (; ), in which the benefits are likely to outweigh the risks of antivenom, so clinicians will need to decide for individual patients. The study also shows that late antivenom, >6 h post-bite, is not effective (), has a similar risk of adverse reactions and should not be administered.
The proportion of patients with anaphylaxis is greater in this study compared to all antivenom administrations in Australian snakebites [Citation31]. It may be due to the previously shown greater rate that occurs with tiger snake antivenom [Citation31,Citation32]. In addition, the rationale for the use of tiger snake antivenom is historical and remains unclear. One patient in this study was administered tiger snake antivenom early but still developed severe myotoxicity, so larger doses or perhaps polyvalent antivenom (in lieu of black snake antivenom) should be considered in these cases.
The study adds to the overall evidence that early antivenom prevents myotoxicity in snake envenomation. It supports ongoing efforts to increase early antivenom use in snakebite patients. However, a major barrier to earlier administration is recognizing systemic envenomation. Currently, the best early indicators of systemic envenomation are non-specific systemic symptoms, such as vomiting, headache, nausea and abdominal pain. For this reason, the decision to give antivenom should be made soon after a patient presents to hospital, based on the likelihood of a definite bite and the absence and/or severity of symptoms, prior to blood tests being sent [Citation33]. Ideally, bedside or rapid laboratory tests will be developed to quickly determine if there is systemic envenomation.
In red-bellied black snake envenomation, there may be evidence of an anticoagulant coagulopathy, with a prolonged activated partial thromboplastin time on the initial blood drawn. However, this occurred in only one fifth of patients (). There is variability in the reagents and analyzers used for the activated partial thromboplastin time [Citation34]. A prolonged activated partial thromboplastin time is highly indicative of systemic envenomation, but a normal activated partial thromboplastin time has a low negative predictive value. None of the patients recruited to the randomized controlled trial had an abnormal activated partial thromboplastin time, which is most likely due to these patients all being recruited from the one regional health service, in which the laboratory analyzer and reagents for the activated partial thromboplastin time assay are known to not elevate in red-bellied black snake bites [Citation34]. The activated partial thromboplastin time normalized in all patients re-tested post-antivenom, indicating that it is a reversible effect of venom.
The major limitation of this study was the small number of patients recruited to the randomized controlled trial, making the results not statistically significant. However, we recruited a large contemporaneous group of non-randomized patients, using exactly the same research infrastructure and pre-defined outcomes [Citation2]. The effect size in randomized and non-randomized patients was remarkably similar, and the greater numbers indicated a statistically significant result. Patients administered antivenom > 6 h post-bite were generally more severe than patients never receiving antivenom, indicating that developing myotoxicity drove late antivenom administration ( and ).
Conclusion
We have shown that tiger snake antivenom significantly reduces the proportion of patients developing myotoxicity in red-bellied black snake bites. In translating this into clinical practice, this needs to be balanced with over one third of patients developing hypersensitivity reactions. If antivenom is administered, it must be administered within 6 h. Late antivenom should not be given as it carries the same risks of hypersensitivity reactions but appears completely ineffective.
Authors’ contributions
GI and NB designed the study; GI and MD identified patients, recruited them and supervised data and blood collection; KF undertook venom assays; GI and SJ did the data extraction; GI and NB carried out the analysis of the data; GI did the literature review; GI drafted the manuscript. All authors read and approved the final manuscript. GI is guarantor of the paper.
Supplemental Material
Download JPEG Image (346.6 KB)Acknowledgments
We acknowledge the assistance of the medical and nursing staff at the emergency departments across Eastern Australia for assisting with data collection. We also thank the Clinical Toxicology Research Group research staff members for data entry, maintenance of the Australian Snakebite Project database, and for obtaining medical records for study participants, including Leonie Calver, Kylie Tape, Marea Herden, Shane Jenkins, Suzanna Belavic (education), Rosemary James. We thank Mal Eutick for advice in regards to the placebo.
Disclosure statement
All authors have completed the Unified Competing Interest form at http://www.icmje.org/coi_disclosure.pdf (available on request from the corresponding author) and declare: no support from any organization for the submitted work; no financial relationships with any organizations that might have an interest in the submitted work in the previous three years; no other relationships or activities that could appear to have influenced the submitted work.
Data availability statement
Data supporting the findings of this study are available on request from the corresponding author [GI]. The data are not publicly available due to confidentiality and ethical requirements.
Additional information
Funding
References
- Kasturiratne A, Wickremasinghe AR, de Silva N, et al. The global burden of snakebite: a literature analysis and modelling based on regional estimates of envenoming and deaths. PLoS Med. 2008;5(11):e218. doi:10.1371/journal.pmed.0050218.
- Johnston CI, Ryan NM, Page CB, et al. The australian snakebite project, 2005-2015 (ASP-20). Med J Aust. 2017;207(3):119–125.
- Isbister GK, Scorgie FE, O'Leary MA, et al. Factor deficiencies in venom-induced consumption coagulopathy resulting from Australian elapid envenomation: australian Snakebite Project (ASP-10). J Thromb Haemost. 2010;8(11):2504–2513.
- Johnston CI, Isbister GK. Australian snakebite myotoxicity (ASP-23). Clin Toxicol (Phila). 2021;59(7):611–618. doi:10.1080/15563650.2020.1836377.
- Noutsos T, Currie BJ, Isoardi KZ, et al. Snakebite-associated thrombotic microangiopathy: an Australian prospective cohort study [ASP30]. Clin Toxicol (Phila). 2022;60(2):205–213. doi:10.1080/15563650.2021.1948559.
- Campbell CH. Myotoxic paralysis and hemolytic anemia due to king brown snake bite. Aust N Z J Med. 1984;14(2):169.
- Maduwage K, Buckley NA, de Silva HJ, et al. Snake antivenom for snake venom induced consumption coagulopathy. Cochrane Database Syst Rev. 2015;2015(6):CD011428.
- Silva A, Hodgson WC, Isbister GK. Antivenom for Neuromuscular Paralysis Resulting From Snake Envenoming. Toxins (Basel). 2017;9(4):143. doi:10.3390/toxins9040143.
- Johnston CI, Brown SG, O'Leary MA, et al. Mulga snake (Pseudechis australis) envenoming: a spectrum of myotoxicity, anticoagulant coagulopathy, haemolysis and the role of early antivenom therapy - Australian Snakebite Project (ASP-19). Clin Toxicol (Phila). 2013;51(5):417–424. doi:10.3109/15563650.2013.787535.
- Churchman A, O'Leary MA, Buckley NA, et al. Clinical effects of red-bellied black snake (Pseudechis porphyriacus) envenoming and correlation with venom concentrations: australian Snakebite Project (ASP-11). Med J Aust. 2010;193(11-12):696–700.
- Lalloo DG, Trevett AJ, Korinhona A, et al. Snake bites by the Papuan taipan (Oxyuranus scutellatus canni): paralysis, hemostatic and electrocardiographic abnormalities, and effects of antivenom. Am J Trop Med Hyg. 1995;52(6):525–531. doi:10.4269/ajtmh.1995.52.525.
- Mion G, Larréché S, Benois A, et al. Hemostasis dynamics during coagulopathy resulting from Echis envenomation. Toxicon. 2013;76:103–109. doi:10.1016/j.toxicon.2013.09.003.
- Silva A, Scorgie FE, Lincz LF, et al. Indian Polyvalent Antivenom Accelerates Recovery From Venom-Induced Consumption Coagulopathy (VICC) in Sri Lankan Russell’s Viper (Daboia russelii) Envenoming. Front Med (Lausanne). 2022;9:852651. doi:10.3389/fmed.2022.852651.
- Isbister GK, Duffull SB, Brown SG. Failure of antivenom to improve recovery in Australian snakebite coagulopathy. QJM. 2009;102(8):563–568. doi:10.1093/qjmed/hcp081.
- Pearn J, McGuire B, McGuire L, et al. The envenomation syndrome caused by the Australian Red-bellied Black Snake Pseudechis porphyriacus. Toxicon. 2000;38(12):1715–1729. doi:10.1016/s0041-0101(00)00102-1.
- AMH. Tiger snake antivenom: in Australian Medicines Handbook (online). Adelaide: Australian Medicines Handbook Pty Ltd.; 2024. https://amhonline.amh.net.au/
- Isbister GK, Buckley NA, Page CB, et al. A randomized controlled trial of fresh frozen plasma for treating venom-induced consumption coagulopathy in cases of Australian snakebite (ASP-18). J Thromb Haemost. 2013;11(7):1310–1318.
- O'Leary MA, Isbister GK, Schneider JJ, et al. Enzyme immunoassays in brown snake (Pseudonaja spp.) envenoming: detecting venom, antivenom and venom-antivenom complexes. Toxicon. 2006;48(1):4–11. doi:10.1016/j.toxicon.2006.04.001.
- Allen GE, Brown SG, Buckley NA, et al. Clinical effects and antivenom dosing in brown snake (Pseudonaja spp.) envenoming–Australian snakebite project (ASP-14). PLoS One. 2012;7(12):e53188. doi:10.1371/journal.pone.0053188.
- Isbister GK, Buckley NA, Brown SG. Tiger snake (Notechis spp) envenoming: australian Snakebite Project (ASP-13). Med J Aust. 2013;198(4):194–195. doi:10.5694/mja12.11690.
- Sanhajariya S, Duffull SB, Isbister GK. Investigating myotoxicity following Australian red-bellied black snake (Pseudechis porphyriacus) envenomation. PLoS One. 2021;16(9):e0256653. doi:10.1371/journal.pone.0256653.
- Gulati A, Isbister GK, Duffull SB. Effect of Australian elapid venoms on blood coagulation: australian Snakebite Project (ASP-17). Toxicon. 2013;61:94–104. doi:10.1016/j.toxicon.2012.11.001.
- Isbister GK, Brown SG, Page CB, et al. Snakebite in Australia: a practical approach to diagnosis and treatment. Med J Aust. 2013;199(11):763–768.
- Isbister GK, White J, Currie BJ, et al. Clinical effects and treatment of envenoming by Hoplocephalus spp. snakes in Australia: australian Snakebite Project (ASP-12). Toxicon. 2011;58(8):634–640. doi:10.1016/j.toxicon.2011.09.013.
- Kulawickrama S, O'Leary MA, Hodgson WC, et al. Development of a sensitive enzyme immunoassay for measuring taipan venom in serum. Toxicon. 2010;55(8):1510–1518. doi:10.1016/j.toxicon.2010.03.003.
- Ryan NM, Kearney RT, Brown SG, et al. Incidence of serum sickness after the administration of Australian snake antivenom (ASP-22). Clin Toxicol (Phila). 2016;54(1):27–33. doi:10.3109/15563650.2015.1101771.
- Brown SG. Clinical features and severity grading of anaphylaxis. J Allergy Clin Immunol. 2004;114(2):371–376. doi:10.1016/j.jaci.2004.04.029.
- Sampson HA, Muñoz-Furlong A, Campbell RL, et al. Second symposium on the definition and management of anaphylaxis: summary report–Second National Institute of Allergy and Infectious Disease/Food Allergy and Anaphylaxis Network symposium. J Allergy Clin Immunol. 2006;117(2):391–397. doi:10.1016/j.jaci.2005.12.1303.
- Lim AY, Singh PN, Isbister GK. Severe rhabdomyolysis from red-bellied black snake (Pseudechis porphyriacus) envenoming despite antivenom. Toxicon. 2016;117:46–48. doi:10.1016/j.toxicon.2016.03.016.
- Johnston CI, Ryan NM, O'Leary MA, et al. Australian taipan (Oxyuranus spp.) envenoming: clinical effects and potential benefits of early antivenom therapy - Australian Snakebite Project (ASP-25). Clin Toxicol (Phila). 2017;55(2):115–122. doi:10.1080/15563650.2016.1250903.
- Isbister GK, Brown SG, Investigators ASP. Bites in Australian snake handlers–Australian snakebite project (ASP-15). QJM. 2012;105(11):1089–1095. doi:10.1093/qjmed/hcs132.
- Isbister GK, Tankel AS, White J, et al. High rate of immediate systemic hypersensitivity reactions to tiger snake antivenom. Med J Aust. 2006;184(8):419–420. doi:10.5694/j.1326-5377.2006.tb00297.x.
- TherapeuticGuidelines. Toxicology and toxinology. Melbourne: Therapeutic Guidelines Ltd.; 2020.
- Lincz LF, Scorgie FE, Johnston CI, et al. Comparative sensitivity of commercially available aPTT reagents to mulga snake (Pseudechis australis) venom. Pathology. 2014;46(5):444–449. doi:10.1097/PAT.0000000000000120.