ABSTRACT
The occupation of small islands presents particular challenges for people largely related to limited terrestrial resources and susceptibility to natural disasters. Nevertheless, the challenges and risks inherent in maintaining stable populations on small islands can be offset or overcome through the use of maritime technologies and exchange networks. The archaeology of Here Sorot Entapa rockshelter (HSE) on Kisar Island in the Wallacean Archipelago provides an unparalleled record for examining these issues in Southeast Asia. Kisar is the smallest of the Wallacean islands known to have a Pleistocene occupation record, and one of the smallest permanently inhabited today. Our results indicate that Here Sorot Entapa was first occupied in the terminal Pleistocene by people with advanced maritime technology who made extensive use of local marine resources and engaged in social connections with other islands through an obsidian exchange network. As a result, populations appear to have been maintained on the island for approximately 6,000 years. In the early Holocene occupation at HSE ceased for unknown reasons, and the site was not reoccupied until the mid-Holocene, during which time a major change in the lithic resources can be observed and the exchange network appears to have ceased.
INTRODUCTION
Islands are generally characterized in archaeology as remote, marginal, and isolated. Small islands in particular (i.e., those between ∼1 and 500 km2) are often neglected by archaeologists due to the perception that they would have been too marginal to have sustained human populations (Fitzpatrick et al. Citation2016). This widely held perception is in large part due to the acceptance of the ‘Theory of Island Biogeography’ (MacArthur and Wilson Citation1967) by archaeologists. This theory posits that smaller islands will have lower ecological and biological diversity (i.e., a smaller equilibrium species number) due to their smaller area, and thus be less able to sustain human settlement over longer timescales. However, the theory also posits that the smaller the distance of the island from another landmass from which recolonization can occur, the larger the equilibrium species number. Thus, islands closer to the mainland will have higher species equilibrium numbers than isolated islands, helping to mitigate the area effect. When considering human dispersal and colonization of islands, other factors may further offset the small island effect (Fitzpatrick et al. Citation2016; Keegan et al. Citation2008), perhaps most importantly the degree of maritime and fishing technology and knowledge available, which would free colonizers from insular terrestrial resource restrictions.
Recent publications have questioned archaeologists' use of, and the utility of the term ‘colonization’ in discussions of the occupation of islands. Leppard and Runnels (Citation2017:511), for example, point out the potential “spectrum of ecodynamic and behavioral processes” implied by this term and question whether its use is even appropriate to pre-Holocene populations. They highlight the need to draw a distinction between ‘passive’ and ‘strategic’ colonization (Leppard and Runnels Citation2017). While their argument pertains primarily to the capabilities of non-sapiens hominins, it is perhaps equally applicable to island colonization by Homo sapiens in the Pleistocene. For example, Pleistocene watercraft may have been adequate for navigating rivers and inshore coastal environments, but are likely to have been caught by strong currents or storms if used further out to sea and, without the ability to travel against the wind, been taken off-course, potentially leading to castaway settlements.
These concerns notwithstanding, central to archaeological investigations of settlement histories is the ability to differentiate continuous settlement versus occasional visitation, at both the site and island scale. Radiocarbon dates represent probabilities, and the error range does not allow discrimination between annual short-term visits by those using an island as a foraging locale and residential island occupation, where a site may be intensively used for only a few days or weeks prior to movement to another camp on some other part of the island. Fitzpatrick et al. (Citation2016) point out the necessity to at least try to differentiate what type of occupation we are dealing with. While in some regions this may be possible based on the presence of seasonally available faunal resources or other seasonal indicators, in most tropical islands where seasonality is low, this is not possible. Research on the Central Torres Strait islands has even questioned the utility of such distinctions when dealing with small islands, arguing that island use is much more fluid and that patterns and length of occupation will vary annually depending on weather patterns and availability of food and fresh water (McNiven Citation2015a, Citation2015b, Citation2016).
Pivotal to all of these issues is the sophistication of maritime technology possessed by a community. Occupation is only sustainable on small islands with unpredictable fresh water supplies, or in the event of environmental or geological catastrophes, if one has both the ability to leave and somewhere to relocate to. Populations on small islands with marginal environments can offset risk by using a variety of social strategies such as mobility, storage, and exchange. In this case, high mobility to cement social networks of reciprocity (even in good years) increases the odds of long-term survival in the event that resources fail (McNiven Citation2015a, Citation2015b). Barham (Citation2000:238) notes that in the Central Torres Strait Islands, in the event of drought, warfare, environmental catastrophes or crop failure, “investment in canoe technology and social alliances permitted temporary abandonment of islands, immediate access to expanded resource areas, and population relocation”.
Here we discuss the archaeological record from the Here Sorot Entapa rockshelter on Kisar Island in the Wallacean Archipelago. We pay particular attention to human adaptations to this small island using faunal, lithic, and other artifactual data in light of some of the issues raised above. Kisar, and the Wallacean Archipelago more generally, provide useful additions to the literature on island archaeology due to the depauperate nature of the terrestrial fauna in most islands of the archipelago, and its smaller islands in particular. Kisar is the smallest of the Wallacean islands known to have a Pleistocene occupation record, and one of the smallest permanently populated today. Due to its steep coastline, Kisar's total land area would have changed little during the course of fluctuations in sea level over the past 50,000 years, making it an interesting case study in terms of viability of colonization and settlement of small islands in the Pleistocene.
Kisar Island
Kisar is located just over 25 km north east of Timor-Leste in the Indonesian province of Maluku Barat Daya (). Kisar is an emergent island in the hinterland of the active Banda arc–continent collision zone. Today it is ca. 10 × 8.7 km with a total area of 81.15 km2. It has a narrow coastal platform owing to its rapid rate of uplift and deep offshore profile. The steep offshore profile means that its land area has changed little during the sea level fluctuations of the past 50,000 years. Even during periods of maximum low stand, it would never have been much larger than it is today. During the Last Glacial Maximum (LGM) Kisar had an estimated land area of ca. 123 km2; accounting for an average uplift rate of 0.5 m/ka (Major et al. Citation2013) and a sea level 135 m below present (Lambeck and Chappell Citation2001). Kisar originated from uplifting, high-pressure metamorphic rocks that formed through the collisional deformation of the India-Australian Plate–Banda Sea Plate interaction (Hall Citation2002; Major et al. Citation2013). While the metamorphic core of Kisar first emerged ca. 450 ka (Major et al. Citation2013), the island is almost completely encircled by a series of Quaternary, coralline limestone terraces (Agustiyanto et al. Citation1994; Major et al. Citation2013) that run parallel to the coastline. It is within these terraces that erosion and dissolution has produced shelters and caves, including Here Sorot Entapa (HSE), the subject of our excavation.
Figure 1 Maps showing the location of Kisar Island and HSE site, and its proximity to neighboring islands. A) Dark gray indicates reconstructed sea levels at c. 15 ka. B) Distance (km) from Kisar to its nearest neighbors for present day (small values), and ca. 15 ka (larger values).
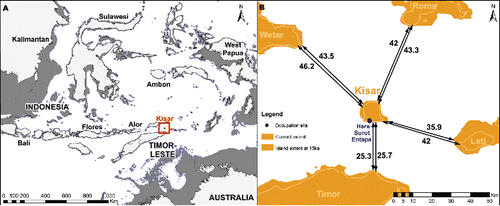
Kisar today has an extremely depauperateterrestrial fauna with only a few species of bats, small rodents, small lizards, and snakes recorded (How et al. Citation1996; Maryanto et al. Citation2005; Monk et al. Citation1997; Prawasti et al. Citation2013; Zug Citation2010). During the course of our surveys we also identified the presence of at least one shrew species. The bird fauna of Kisar is comparatively well studied with 44 resident species so far identified (Finsch Citation1898; Natus Citation2005; Trainor Citation2003). Present-day annual rainfall on Kisar ranges from 900 to 1200 mm/pa (Direktorat Pendayagunaan Pulau-Pulau Kecil Citation2012), 80% of which falls between the months of December and May (Regional Physical Planning Project for Transmigration Citation1989), making it the driest island in south Maluku (Trainor Citation2003). Freshwater is scarce during the dry season and some of the settlements on the island today buy water for domestic use, which is pumped from bores. Savannah and plantations dominate the present vegetation, however, Trainor (Citation2003) predicts the island was once covered almost entirely in tropical dry and semi-evergreen forests. While the coastal waters surrounding Kisar abound with marine life, this is somewhat offset by narrow reef platforms resulting from the steep offshore profile, and depth of surrounding waters. The uplifted limestone terraces create steep, almost vertical cliffs girting large parts of the island, and easy access to the sea is available in only a few areas. A high energy beach about 135 m west of HSE provides an access point to the coast.
THE HERE SOROT ENTAPA EXCAVATION: MATERIALS AND METHODS
Here Sorot Entapa (HSE) was excavated by a joint Australian-Indonesian team over one field season in September 2015. HSE is formed in a raised coralline terrace located approximately 80 m from the coast and about 24 m above mean sea level on the south coast of Kisar, facing Timor-Leste (). The site comprises a shelter about 12 m in length at its widest point, and 10 m in depth from the back wall to the dripline (). The wall of the shelter opens into an enclosed chamber with a dome roof (approximately 5 m high) that is on average 9 m deep from the opening to the back wall and about 6 m across. The narrow entrance allows little light to enter the cave. The floor of the site has several stone features that were said by the locals to be sacred, and the shelter walls contain painted motifs in a variety of colors (O'Connor et al., Citationin press).
Figure 3 Plan of the HSE shelter and cave with excavation squares indicated in yellow and large rocks/boulders in blue.
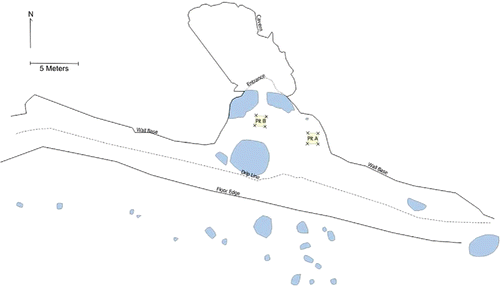
Two 1 × 1 m2 test pits (Squares A and B) were excavated in the main shelter floor outside the cave entrance but within the dripline (). Square A was located near the east wall of the shelter; Square B was situated centrally but avoiding the stone features. Both were excavated in 5 cm spits within sedimentary units. Square A was excavated to a depth of 1.3 m and Square B to a depth of 1.2 m, when natural coralline rubble and sand was encountered.
Excavation and Recovery
All excavated sediment was dry sieved and then wet sieved through a 1.5 mm mesh screen. This ensured good recovery of small bones and shell artifacts. All finds were then dried before being sorted and separated into categories of bone, stone, shell, urchin, crab, charcoal, and seeds.
Charcoal and Shell Sampling for Radiocarbon Dating and the Bayesian Model
Charcoal and shell samples for radiocarbon dating were removed in situ during excavation and their 3D position recorded. Samples were also removed from the walls at the end of the excavation and the sample location marked on section drawings.
Nineteen charcoal and marine shell samples were dated by the Australian National University Radiocarbon Dating Laboratory, while 12 marine shell samples were dated at the University of Waikato Radiocarbon Dating Laboratory. The C14 ages were then calibrated in OxCal v.4.3 (Ramsey Citation2009a) using the IntCal13 (for charcoal samples) and Marine13 (for marine shell samples) calibration curves (Reimer et al. Citation2013). A multiphase Bayesian model was constructed using these dates in OxCal to reduce the uncertainty of the age estimates and to predict the timing of the transitions between the different identified stratigraphic units. Each unit identified in the stratigraphy () was modeled as a Phase and ordered based on their stratigraphic position. Double boundaries were inserted between each unit to account for discontinuous sedimentation rates and possible hiatuses in the record. One exception was in Unit 8 where the date from Square A was significantly older than those from Square B and, based on the location of the excavation squares in the shelter and the contiguity of the corresponding stratigraphic units between squares, was thought to represent an earlier time in the deposition of Unit 8. We therefore split Unit 8 into two Phases within the model, Lower Unit 8 and Upper Unit 8, but with a continuous sedimentation rate (only a single boundary) modeled between them. The General t-type Outlier Model (Ramsey Citation2009b) and a prior outlier probability of 5% was applied to the overall model and each individual date, respectively. This model is the one most commonly used for archaeological dates in determining the probabilities of outliers and the scale of offset applied to the data within the model (Ramsey Citation2009b; Wood et al. Citation2016).
Stone Artifacts
The total number of stone flaked artifacts [TNA] was counted for all raw materials. Analysis also included a count of the minimum number of flakes [MNF], an artifact count sensitive to flake fragmentation (Hiscock Citation2002). Statistical tests were conducted using SPSS V24, and included the Wilcoxon Signed Rank test for comparison of related non-parametric variables.
Vertebrate Fauna
The HSE vertebrate material was first sorted into rough biological classes (bony and cartilaginous fish, bird, lizard, marine turtle, medium-sized mammal, murid, and snake) at the Australian National University in Canberra. All bone fragments from Square B were anatomically identified to the lowest taxonomic level possible by comparison with modern reference collections housed at the Department of Archaeology and Natural History Osteology Laboratory at the Australian National University (ANU), and at the Muséum National d'Histoire Naturelle in Paris, as well as some reference illustrations in ichthyoarchaeology (Bellwood Citation1994; Cannon Citation1987; Gregory Citation1933; Lepsikaar Citation1994; Rivaton and Bourret Citation1999; Tercerie et al. Citation2014 [v.2017]; Tyler Citation1980). Ichthyoarchaeology in the tropical Indo-Pacific island region is especially challenging due to the combination of high regional marine biodiversity and conservative bone morphological variability within most fish families (Samper Carro et al. Citation2017). Indeed, there are no less than 3,567 species of marine fishes living near Indonesian coastlines (Froese and Pauly Citation2010 [v.2017]). Among these, 2,103 are reef-associated. Identification based on comparative anatomy is difficult and often limited to family level (Fitzpatrick et al. Citation2011; Lambrides and Weisler Citation2013, Citation2016).
Fortunately, the reference collections used in this study are among the best for Pacific fish in the world. They facilitated the identification of a wide range of anatomical elements that include the five-paired jaw bones and unique post-cranial bones such as pharyngeal plates and dermal spines (Campos Citation2009; Leach Citation1997; Samper Carro et al. Citation2016, Citation2017), as well as an expanded set of cranial elements, and vertebrae. The taxonomic determination of fish vertebrae is important as it permits the identification of fish families whose cranial bones are very fragile and do not preserve (Lambrides and Weisler Citation2013). The unidentified fish fragments have been counted and classed as boney ray-fined fishes (Teleostei) of the Actinopterygii, which makes up the largest number of fish species in the oceans of the world (Nelson Citation2006). Some fish skeletal elements are better represented than others, e.g., identification of Balistidae dorsal spines is straightforward compared with those of other families. This creates bias during the identification phase, although this bias is consistent between units within the sequence.
Tetrapod bones were identified to various taxonomic levels depending on degree of fragmentation and reference material available at ANU. The most fragmented bones, lacking diagnostic morphological features, were only identified to superclass (Tetrapoda) or class (Reptilia, Aves, Mammalia). Bird bones were not identified to any level lower than class, due to time and comparative collection limitations; likewise limited reference material for lizards, snakes, and bats restricted identification of these taxa to suborder (Lacertilia, Serpentes, Chiroptera). However, fruit bat (Pteropodidae) bones are quite distinctive from insectivorous bats (Yangochiroptera) and these could often be distinguished based on articulating limb appendages and cranial material. Turtle bones were identified to the superfamily level (Chelonioidea) as the seven extant species worldwide can only be distinguished by mandibles and crania (Wyneken and Witherington Citation2001), elements lacking in the HSE vertebrate assemblage. Rodents belonging to Muridae were sorted on size (small, large, and giant). More specific identifications to species and genus were made on the maxillae and mandible tooth rows.
Vertebrates were quantified by the Number of Identified Specimens Present (NISP) and weight. These quantitative methods are independent of aggregated provenance units and avoid the overestimation of rare taxa (Lyman Citation2008). However, we recognize that this may result in an inflated estimate of some taxa with regards to the disproportionate preservation of certain bones between different taxa (such as pharyngeals of Scaridae or Labridae) (Poplin Citation1976). For further discussion of the taphonomic observations at HSE, see Supplementary Information: S1.
Molluscan Fauna
All shells and shell fragments from the site were retained for analysis and are stored at the University of Gadjah Mada. To date only the shell from two spits has been identified. Shells and shell fragments were identified to the lowest possible taxonomic level using reference books for Indonesian molluscs (Dharma Citation1988, Citation1992, and Citation2005; and the online database WoRMS [WoRMS Editorial Board Citation2017]). Molluscs were quantified by weight (g), Minimum Number of Individuals (MNI) and Number of Identified Specimens Present (NISP). The comparative merits and disadvantages of weight, MNI, and NISP methodologies for quantifying shell are well known (see for example, Claassen Citation2000). Weight, MNI, and NISP were all calculated in an attempt to assess potential biases inherent in each method. For MNI a single shell feature was selected for each species and used throughout, e.g., the apices for gastropods and the posterior valve for chitons. For bivalves, both left and right hinges were counted, with the greater number selected from the total.
HSE CHRONOLOGY AND STRATIGRAPHY
The stratigraphy is composed of nine stratigraphic units (). Unit 1, the lowest, is a reddish yellow (7.5 YR 6/6–5/6) sand with abundant coral. A small amount of cultural material was recovered in the upper part of Unit 1; however, it is thought to have been mixed into the culturally sterile coralline sands at the time of initial occupation. Unit 2, the first unit with significant amounts of cultural material, is composed of a dark brown (7.5 YR 3/3–3/4) sandy silt, with some shell and small rock clasts. A hearth feature is preserved within this unit in the west wall of Square A. Unit 3 is a light gray (7.5 YR 7/1) compact ashy layer, dense with shell and containing most of the cultural material recovered. This ash layer is vertically thinner in Square A, varying between approximately 5 to 20 cm, whereas in Square B it is up to 70 cm thick. Square B also contains several discrete charcoal and ash-rich features within Unit 3 in the southern and eastern walls. Unit 4 is composed of a dark brown (7.5 YR 2.5/2) sandy silt, which appears in Square A directly above the dense shell layer, with hearth features visible in the western and southern walls. Unit 4 is most clear in the southern wall of Square B, with less shell and ash than the preceding unit. Unit 5 is a concentration of rock clasts and angular gravels surrounded by very loose dark brown silt (7.5 YR 3/2–4/2), with some ash and shell. This unit is only visible in the Square A walls, and was not detected in Square B. Unit 6, a compact pink to gray ash (7.5 YR 2.5/2) with lenses of white ash is present in both squares, particularly in the southern and western walls of Square A. The overlying Unit 7 is characterized by dark brown sandy silt with increased charcoal content, and several hearth features evident in both squares. The boundary between Unit 7 and the underlying units (Unit 6 in Square A, and Units 6 and 4 in Square B) is very sharp, with clear changes in color, compaction, sorting, and content. Unit 8 is the same color and sediment composition, but contains more charcoal and organics. The boundary between Unit 7 and 8 in the western and northern wall of Square B includes several thin layers of fine ash, dense charcoal, and burnt orange/yellow sediment (10 YR 4/6). In situ dating of samples from one of these charcoal lenses suggests these thin layers are contemporaneous with either Unit 7 or 8. The uppermost Unit 9 includes multiple thin layers of topsoil with fine rootlets. This unit is thinly distributed across the surface of Square A, although reaches greater depth in the northern wall of Square B.
Radiocarbon dating of shell and charcoal samples removed from the walls indicates that the stratigraphic units are similar in age across the site (; ). The deposit can be divided into two broad chronological phases. Phase 1 begins with initial occupation around 15,500 years ago and continues into the early Holocene ∼9,500 years ago. This phase includes stratigraphic Units 2 to 6, and is characterized by the abundance of siliceous chert and the presence of obsidian. The second phase of occupation commences about 4,900 years ago and continues until about 1800 cal BP or more recently. Phase 2 includes stratigraphic Units 7 to 9, and is characterized by an abundance of crystal quartz and the presence of pottery (see Supplementary Information: S2). Between these two phases, specifically between stratigraphic Units 6 and 7, there appears to have been a temporal hiatus in human occupation. The multiphase Bayesian model of the dates and likely unit terminals also supports this hiatus in the record, with a lack of overlap between the modeled end date for Unit 6 and start date for Unit 7 (; ).
Table 1. Radiocarbon samples analyzed from squares (Sq.) A and B, HSE. ANU = The Australian National University Radiocarbon Dating Laboratory. Wk = The University of Waikato Radiocarbon Dating Laboratory. Calibrated and modelled in OxCal v.4.3 (Ramsey Citation2009a) using the IntCal13 (for charcoal) and Marine13 (for shells) calibration curves (Reimer et al. Citation2013). Unmodelled and modelled calibrated dates are all shown at 95.4% posterior probability. Units and spits correspond to the stratigraphy (see ), Phase 1 is shaded purple, Phase 2 is orange. Modelled dates are shown in .
Figure 5 Bayesian model of radiocarbon dates from HSE. Modeled in OxCal v.4.3 (Ramsey Citation2009a) using the IntCal13 (for charcoal) and Marine13 (for shells) calibration curves (Reimer et al. Citation2013). Units correspond to and . Black probability distributions represent the modeled date (with 95.4% probability range bracketed underneath), while the pale distributions represent areas where the unmodeled, calibrated date does not overlap with the model. Sample codes are written thus: Square_Radiocarbon Laboratory_Individual Sample Number; and correspond to . Prior and posterior outlier probabilities follow in square brackets as follows: [O:posterior/prior].
![Figure 5 Bayesian model of radiocarbon dates from HSE. Modeled in OxCal v.4.3 (Ramsey Citation2009a) using the IntCal13 (for charcoal) and Marine13 (for shells) calibration curves (Reimer et al. Citation2013). Units correspond to Figure 4 and Table 1. Black probability distributions represent the modeled date (with 95.4% probability range bracketed underneath), while the pale distributions represent areas where the unmodeled, calibrated date does not overlap with the model. Sample codes are written thus: Square_Radiocarbon Laboratory_Individual Sample Number; and correspond to Table 1. Prior and posterior outlier probabilities follow in square brackets as follows: [O:posterior/prior].](/cms/asset/863cb138-16fe-4ffd-b1e7-7194c66efa9b/uica_a_1443171_f0005_c.gif)
Phytoliths in the HSE Sediments
Analysis of phytoliths from sediment samples down the profile of Square B identified 17 morphotypes, the majority of which are from the Poaceae (grasses), one specific to Bambusoideae (Alifah Citation2016). Morphotypes distinctive of the Arecaceae (palms) were also identified from sediment samples as well as residue on some of the pottery sherds (Alifah Citation2016), along with types found in most tropical trees and shrubs (An et al. Citation2015; Bowdery Citation1999; Piperno Citation2006). The sample from the dense ash layer of Stratigraphic Unit 3 produced the greatest number of phytoliths. The sample corresponding with Spit 7 records an almost complete lack of phytoliths (three Poaceae types) and is notable for its temporal alignment with the sequence hiatus (Alifah Citation2016).
HSE ARTIFACTS
Stone Artifacts
A total of 3,703 flaked stone artifacts were recovered from the two excavation squares, with most found in Square B, likely due to its more central location in the living floor of the shelter (A = 744, B = 2959). The dominant raw material is a siliceous chert () ranging in color from blue to red, and often containing flaws from chemical and physical weathering. Chert occurs in Timor-Leste as cobbles in creeks and rivers along the north coast (Hawkins et al. Citation2017a; Marwick et al. Citation2016); however, the HSE chert is less homogenous than chert in the Timor-Leste sites so is unlikely to have had the same origin. Chert seams may occur on Kisar but none have yet been identified. Crystal quartz, recognized by a highly translucent and fine crystal structure, was occasionally exploited, with a major shift in preference in the upper mid- to late Holocene part of the sequence (). For example, in Square B chert overwhelmingly dominates the assemblage between ∼14,000 cal BP and ∼11,000 cal BP (Spits 19–10), with a reversal in the upper part of the site where crystal quartz dominates between ∼4800 cal BP and ∼2000 cal BP (Spits 6–1). Likewise in Square A chert dominates from ∼13,600 cal BP to 10,400 cal BP (Spits 16–9) and quartz dominates in Spits 8–1, bracketing a similar period of time (Spit 8 is undated but it likely dates to ca. 5000 and Spit 1 is dated to ∼1800 cal BP) (; ). Comparing the frequency of quartz and chert with a Wilcoxon Signed Rank test these trends were found to be statistically significant (A: Z = −2.046, p = 0.041 B: Z = −4.018, p = 0.001). One hundred and ninety-three obsidian artifacts were recovered in Square B, with a discrete peak in deposition between Spits 19 and 16, coinciding with the general increase in stone artifacts and other cultural materials (). Obsidian was infrequently deposited in Square A (n = 2, Spit 8). In Square B obsidian was mostly deposited in Phase 1. Three glass flakes recovered in the uppermost spit of Square A must be the result of site use after European contact.
Figure 6 Lithics histograms. Artifact frequencies: A) Square A TNA and MNF values, B) Square B TNA and MNF values. Raw material types: C) Square A, D) Square B.
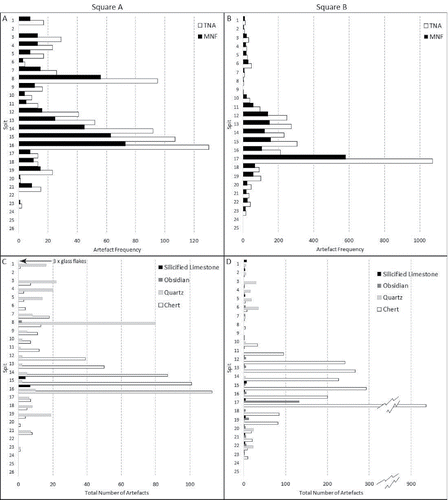
The distribution of artifacts throughout the sequence shows a unimodal discard peak with most artifacts concentrated within the ashy and shell-rich Unit 3 (Spits 16 and 12 in Square A, and Spits 17 and 10 in Square B). The total number of artifacts [TNA] and the minimum number of flakes [MNF] show the same unimodal peak () indicating that the increase in numbers of artifacts at this time is unrelated to breakage. Heat shatter and heat-affected pieces are also moderately rare in each square (A = 48, B = 433), as are flaked pieces (those artifacts displaying partial conchoidal surfaces excluded from the MNF count) which occur in similarly low frequencies (A: n = 206, 27%, B: n = 458, 15%).
Technological diversity is highest around the discard peak with the majority of retouched flakes (A: n = 6, B: n = 17), retouched flake fragments (A: n = 11, B: n = 22), and cores (A: n = 20, B: n = 31) deposited during this time. Retouched flakes are predominately made on chert and described and illustrated in the Supplementary Information: S3.
Shell and Bone Artifacts
Shell technology at HSE is dominated by finely made fishhooks on Tectus niloticus shell. These tools are spread fairly evenly throughout the excavated deposit (), with 35 examples identified. All are jabbing hooks. Most of the hooks are fragmentary, commonly represented by a ca. 10 mm segment of the hook bend, though seven are complete or near-complete (>85% intact). The largest specimen (found in Square B Spit 10) is a finished hook measuring 32.5 mm in absolute height by 24.5 mm in maximum width (). This artifact is significantly larger than the other finished and preform examples recovered, the latter being more gracile, averaging around 10 mm shorter and thinner than the B10 hook (see typical examples in ). Manufacture of the fishhooks appears to have followed the same sequence: first chipping out a rough blank using direct percussion, then targeted pressure flaking, followed by successive phases of grinding to finalize the form. Striations and facets from the refining of the fishhook are clearly evident on all of the finished examples, including those shown in . The presence of six blanks and three preforms alongside the finished fishhooks indicates that all phases of manufacture were undertaken at HSE.
Table 2. Stratigraphic distribution of fishhooks and shell beads. Square A first, shaded blue, followed by Square B shaded pink.
Figure 7 Shell and bone tools from HSE. A) Nautilus pendant with thick red ochre residue from Square B Spit 12; B) bone point from Square B Spit 17; C–D) Tectus fishhooks from Square B Spit 13; E) Tectus fishhook from Square B Spit 10; F–G) fragments of Nautilus two-holed beads from Square A Spit 7; H) Nautilus disc bead from Square A Spit 1 I) Detail of perforations on Nautilus pendant, scale bar = 1 mm; J) working tip on bone tool, scale bar = 1 mm (Photos MCL).
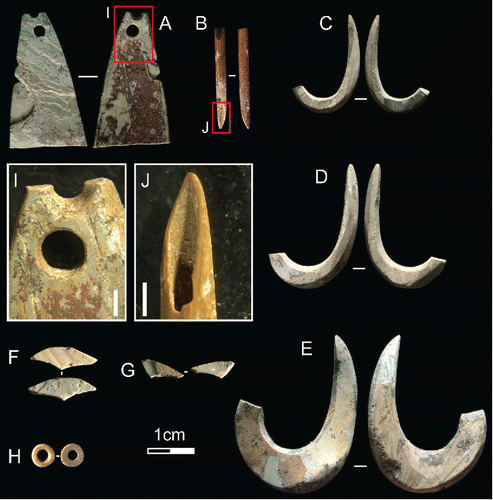
A shaped and perforated artifact made on Nautilus pompilius (length 28.9 mm; ) was recovered from Square B, Spit 12. This artifact exhibits a snap fracture across its proximal end, along with two pressure fractures on its left side. The distal extremity features two perforations, one complete beneath a second which has snapped through its middle (). Given the thick coating of ochre on the ventral face of the piece, it seems likely that this artifact was an item of personal ornamentation, rather than a fishing lure, and one which was rejuvenated when the initial perforation failed.
Other shell artifacts made for personal adornment include small disc beads () and fragments of two-holed beads ()—both made on Nautilus pompilius (). Interestingly, most of these beads were recovered from Spits 6 and 7 of Square A, suggesting either the loss of a beaded object, or a temporally constrained beading activity in this space. The four complete disc beads are similar in size, each falling around 4.5 mm in diameter, an attribute shared by a single bead made on Strombus sp. The 18 fragments originating from two-holed beads would have measured somewhere in the range of 13 mm to 19 mm each when intact. All of these beads and bead fragments exhibit unifacially drilled perforations and striations resulting from the grinding of their surfaces. The two-holed beads also display evidence for the pressure flaking of their perimeter—the primary process through which their final shape was achieved. Also found in Square B Spits 18 and 19 were several worked pieces of Nautilus which exhibited a thick bright red ocherous residue. These pieces were small, and without any characteristic features (such as perforations), and so it is possible that these artifacts originated from either beads/pendants or a shell vessel utilized for holding ground red ochre.
Interestingly, four pieces of branch coral showing signs of use were also identified in Square B Spits 6, 8, and 17 (see example from B6 in ). The coral artifacts display chipped and crushed points (), alongside ground surfaces (), polished high points (), and traces of red, and possibly yellow, ochre. These marks suggest that the coral points were held in the hand (to account for the high polish), and used to reduce marine shell and/or ochre at HSE. A single bone point was recovered from Square B Spit 17. Measuring only 20 mm long (), it may have been used in the production of the shell disc beads, for its diameter reaches only 2.1 mm at its widest point (the perforations of the beads described above average around 1.8 mm), the proximal extremity has snapped through pressure during use, and the distal point displays a rounded and polished bevel (). Light traces of red ochre were also observed on the shaft.
THE FAUNAL ASSEMBLAGE
Preliminary field sorting divided the HSE faunal material into five categories: shell, barnacle, crustacean, urchin, and bone (see Supplementary Information: S1, S4, S5, and S6, for details of laboratory faunal analyses). All categories of faunal data, with the exception of the urchin, peak at ca. 13.5 ka coinciding with the discard peak in stone artifacts in Square B ( and ). Bone weights in Square A also suggest that more bone was discarded during this period; however, the trend is less distinct than in Square B, presumably due to the smaller sample size. A significant decrease in bone and shell NISP and weights in Phase 2 (Spits 6–1 of Sq. A; and 6–1 of Sq. B) compared with Phase 1 (Spits 26–7 of Sq. A; and 25–7 of Sq. B), could indicate that smaller groups of people occupied the site over shorter time periods during the mid-late Holocene compared to the terminal Pleistocene–early Holocene occupation at HSE ( and ; ).
Figure 10 Faunal Histograms for Square B, invertebrate abundance by weight (g) per spit, vertebrate abundance by NISP.
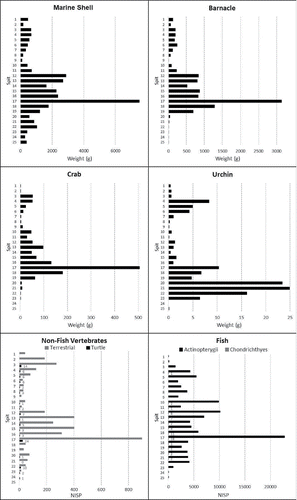
Fish Remains
A total of 106,762 fish bones and bone fragments were recorded in Square B (see Supplementary Information: S4). Only 3.7% of the bones (NISP = 3806) could be identified to the suborder or family level. This low percentage is a direct result of high fragmentation rates and a limited set of identifiable fish elements (see Supplementary Information: S1 and S5). Six orders of fish are present in the HSE assemblage: Carcharhiniformes, Anguilliformes, Beloniformes, Beryciformes, Perciformes, and Tetraodontiformes, representing 26 fish families and one suborder (see Supplementary Information: S4 and S5). Triggerfishes (Balistidae; NISP = 924), sea basses and groupers (Serranidae; NISP = 881) dominate. Labridae (wrasses; NISP = 426), Holocentridae (squirrelfishes and soldierfishes; NISP = 365), Ostraciidae (boxfishes; NISP = 316), and Scaridae (parrotfishes; NISP = 287) are also relatively abundant at HSE (see Supplementary Information: S4 and S5).
The diversity of fish taxa, reflecting both carnivorous and herbivorous/omnivorous species, shows that a variety of mostly in-shore fishing techniques were practiced at HSE over the past 15,000 years (see Supplementary Information: S5, for descriptions of HSE fish family ecology and capture methods). Based on archaeological data and ethnographic observations, these are likely to include angling, trolling, spear fishing, netting, and traps (Ono Citation2010). All fish taxa documented are present in Phase 1, whereas diversity declines in Phase 2, which has a much smaller sample size (12.1%) compared to Phase 1. Linear and non-linear temporal changes in these fishing behaviors were significant, with an increase in the proportions of carnivores relative to herbivores and omnivores during Phase 1 (Units 2–6), a proportional decline in carnivores at the beginning of Phase 2 (Unit 7), followed by a dramatic increase in carnivores by the end of Phase 2 (Units 8–9) (, X² trend = 18.649, p < 0.001, X²departure = 34.279, p = < 0.001). This likely represents fluctuating changes in fish capture methods in response to changes in the structure of fish ecologies within the local marine environment (Boulanger Citation2014). This is in direct contrast to Tron Bon Lei on Alor, where larger carnivores significantly declined and smaller herbivores/omnivores increased in relative abundance over the same time period during the Pleistocene–Holocene transition (Samper Carro et al. Citation2016).
Figure 11 Temporal changes in fishing practice at Here Sorot Entapa as represented by the proportions of carnivores relative to herbivores and omnivores {(Carnivore NISP/Herbivore NISP + Omnivore NISP) × 100} by temporal phase after Butler (Citation1994).
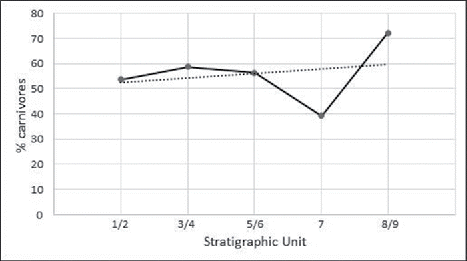
Tetrapod Remains
Tetrapods (NISP = 3634) likely only formed a minor component of people's diet at HSE. The assemblage is dominated by small reptiles (snakes and lizards), mammals (small rats), and birds, most of which are likely troglodytes or prey of owls (see Hawkins et al. Citation2017b and Supplementary Information: S4 and S6). Marine turtles were exploited during both phases of human occupation, while the greater abundance of fruit bats during Phase 1 may indicate more intensive harvesting (Hawkins et al. Citation2016, Citation2017b) during the Pleistocene–Holocene transition. Of interest is the presence of two likely extinct species of rat (one large and one giant) in Phase 1 and the introduction of the Pacific rat, black rat, and domestic dogs during Phase 2. The presence of extinct rat species and more abundant fruit bats in Phase 1, followed by extinction and introduction of commensal rats by Phase 2 may indicate a decline in forested environments during the late Holocene. The cave appears to have had more natural deposition of vertebrates during Phase 2: insectivorous bats, small lizards, and birds were deposited in greater numbers during this late Holocene period, suggesting human site use was less intense.
Shellfish Remains
Preliminary analysis of the shellfish remains in Square A identified 37 different shell species within Gastropoda, 4 species of Bivalveia, 1 species of Polyplacophora, and at least 1 species of Cephalopoda. The following 17 gastropod families were identified: Buccinidae (Buccinidae), Trochidae (Trochus), Tegulidae (Tectus), Nacellidae (Cellana), Patellidae (Scutellastra), Lottiidae (genus unknown), Muricidae (Thalessa, Mancinella, Menthais, Purpura, Muricidea, and Morula), Fasciolariidae (Peristernia), Haliotidae (Haliotis), Nassaridae (Bullia), Cassidae (Cypraeacassis), Cypraeidae (Cypraea, Monetaria, and Mauritia), Strombidae (Lambis, Strombus, and Conomurex), Littorinidae (Tectarius), Camaenidae (genus unknown), Turbinidae (Turbo and Lunella), and Neritidae (Nerita). Families identified from Bivalvia include Tridacnidae (Tridacna), Ostreidae (genus unknown), and Veneridae (genus unknown). Chitonidae were identified from the Class Polyplacophora, and the Family Nautilidae (Nautilus) from the cephalopods. Turbo and Nerita are the most common shell genera recovered from the deposit. The Nautilus was likely collected post-mortem from the shore edge for production of shell artifacts (Langley et al. Citation2016).
DISCUSSION
Our excavation demonstrates that Kisar was first occupied at least 15,500 years ago (15,327–15,730 cal BP, 13,395 ± 33, Wk 43368). The lowest occupation horizon is directly underlain by sterile coralline rubble dated to 45,840 ± 2687 (WK 46537). While this may be a minimum age, it demonstrates that the shelter would have been available for habitation well prior to the date when it was actually occupied. Stone and shell artifacts, as well as all categories of faunal remains, peak about 13,500 years ago, suggesting that site use was most intense during this period. Although we do not know the regularity of HSE occupation or the duration of visits, the site appears to have been as intensively occupied as any of the coastal shelters so far reported from eastern Indonesia. The presence of well-made fishhooks of different sizes shows a high degree of fishing technology, necessary on a small island where terrestrial fauna was extremely depauperate even by Wallacean standards. The finely finished shell hook dated to ∼15,000 years ago is evidence that fishhook technology was well established prior to settlement at HSE. Fishhook manufacture, breakage, and discard were taking place at HSE, which, coupled with the large and diverse fish bone assemblage, suggests that the site was used as a base from which to undertake fishing trips, as well as maintenance activities on a range of fishing gear.
Fishing strategies appear to have fluctuated in response to either changes in technological practice and/or changes in marine ecology. Marine turtles and fruit bats were likely exploited in small numbers to complement protein intake. The presence of bats and extinct rat species suggests that the environment surrounding HSE had some degree of forestation, as proposed by Trainor (Citation2003). Plant foods such as seeds, fruits, nuts, and tubers would have been available in the tropical dry and semi-evergreen forests and provided carbohydrates and calories needed to complement the marine resources; however, we currently have no evidence of what these may have been. The phytolith record leaves open the possibility of palm fruits or starch. Invasive rat species and domestic dogs were introduced to HSE during the late Holocene.
The shell beads show that domestic activities associated with the manufacture and wearing of personal ornaments also took place at the shelter. Such activities are more consistent with a home base or residential site rather than an occasional foraging locale, although by the late Holocene a greater abundance of fauna likely derived from owl pellet remains indicates periods when the site was unoccupied.
Based on the geology of Kisar (Agustiyanto et al. Citation1994), obsidian is exotic to the island. The source of this raw material for lithic manufacture is currently not known. Small numbers of obsidian flakes also occur in coastal Timor-Leste sites and are similarly thought to be exotic to that island (Reepmeyer et al. Citation2011). Obsidian artifacts from the Tron Bon Lei site on nearby Alor have been found to be made from both locally sourced obsidians and exotic obsidian from the same source as the Timor-Leste samples (Reepmeyer et al. Citation2016). In view of its temporal and geographic distribution, in addition to the material quality and lack of a cortex on the artifacts, this latter obsidian is not believed to be native to Alor (Reepmeyer et al. Citation2016). The Kisar obsidian could be from Alor, but it could also be sourced from one of the volcanic islands to the northeast of Alor in the Banda Arc. At least one source sample of obsidian currently housed in the Geologi Museum, Bandung (sample B71), has been collected from these islands and is provisionally ascribed to Wetar.
The HSE assemblage thus indicates that obsidian from a source external to the island appears with earliest occupation in Phase 1. In neighboring Timor-Leste obsidian from an exotic source also appears in a number of sites after 15,000 cal BP. In Jerimalai shelter at the eastern end of Timor, and with an occupation record extending to 42,000 cal BP, most of the obsidian occurs in excavation units bracketed by dates of 15,000 to 4000 cal BP (Reepmeyer et al. Citation2011). At Laili in northern Timor-Leste, where the deposits date between approx. 44,000 and 11,000 cal BP (Hawkins et al. Citation2017a), the only two obsidian artifacts were found in a unit dated between 15,000 and 11,000 cal BP (Hawkens et al. 2017). In Tron Bon Lei in Alor obsidian with the same geochemical signature as the Timor-Leste artifacts occurs in layers post-dating 12,000 cal BP (Reepmeyer et al. Citation2016). In this context Reepmeyer et al. (Citation2016) argued that inter-island maritime connectivity in this region of Wallacea began about 15,000 years ago. They suggested that increased maritime interaction and social connections between populations on different islands may have followed on as a consequence of coastal communities pursuing maritime subsistence pursuits more intensively, as rising sea levels increased the productivity in marine environments (Woodroffe et al. Citation2000). This interpretation is strongly supported by the data from Kisar, which was likely first settled during this period of widespread maritime interaction. Prior to ca. 15,000 years ago, connections between Kisar and neighboring islands appear to have been too tenuous to support a permanent occupation of this tiny island.
While the distances between Kisar and neighboring islands might not seem so great as to inhibit regular visitation, this is very much a perspective based on current maritime technological capacity. Such distances could and did pose severe constraints for groups with simple boats without sails. For example, most Australian islands have no evidence for occupation or even casual visitation prior to ca. 3,000 years ago or more recently (Bowdler Citation1995; Rosendahl et al. Citation2015). At the time of European contact it was observed that most islands more than 10 km distant from the Australian mainland or the large island of Tasmania, were not inhabited or regularly visited by Indigenous groups (Jones Citation1977). Kangaroo Island, a very large island (4400 km2) only 14.0 km from the coast of South Australia, is a case in point. It was occupied when it was part of the Australian mainland in the Pleistocene, was insulated at ca. 10,000 cal BP but was not occupied or visited at the time of European contact. The archaeological record of Kangaroo Island indicates that some people continued to live on the island until about 4,000 years ago and possibly until as recently as 2,000 years ago (Draper Citation1991; Lampert Citation1981). Lampert (Citation1981:184) believed that local extirpation of the population likely occurred as a result of environmental, demographic, and biogeographic constraints. Some of the larger Tasmanian islands in Bass Strait, such as King and Flinders Islands, also have sparse evidence suggesting that they were populated for a few thousand years after insulation, but like Kangaroo Island, were not occupied or used at the time of first European contact (Bowdler Citation1995, Citation2015).
Historical records and oral accounts of maritime crossings to and between islands off the Australian coast indicate that voyaging accidents were common. Records for the Wellesley group of islands in north Queensland are instructive. Although in the protected waters of the Gulf of Carpentaria, Tindale (Citation1977:269) reports that navigation over distances of even a few kilometers was hazardous and casualties were often high. Travel from Bentinck Island (∼150 km2) in the southeast of the archipelago to the mainland was made using rafts, and via stages, with the longest single watercrossing of 10.5 km between Bentinck and Allen Island. Evans (Citation2005:14) reports that this trip was rarely attempted, with some people making the return voyage only a few times in a lifetime. On two separate voyages from Bentinck to Allen Island, drownings occurred, with casualty rates of 20% and 74%, respectively, of the 15 and 19 persons who commenced the voyages. Compared with travel between Kisar and its neighbor islands, currents would have been minimal in the Gulf, putting into perspective the accomplishment of the Kisar colonists 15,000 years ago and the maritime network of obsidian exchange which operated from this time.
Anderson (Citation2018) has argued that early seagoing in the western Pacific can be explained by the “fortuitous conjunction of the warmest seas with a ready availability of large-diameter bamboo that occurred as natural rafts, and which could also be constructed into rafts large enough to transport viable colonizing groups from island to island across Wallacea to Sahul”. While large diameter bamboo grows in the islands of Wallacea today, its distribution appears to be that of a managed plant rather than a native, suggesting it is a translocation to the islands east of the Wallace line. On the basis of Trainor's (Citation2003) prediction of past forest environments on Kisar, we hypothesize that the boats used could have been hollowed canoes or simple plank constructed boats, made from forest timbers. However, the absence of stone axes in ISEA assemblage prior to the Neolithic perhaps suggests boat construction in raft form was more likely. Without the aid of sails, crossing between islands would have been challenging and boating casualties may have taken a toll on the Kisar population.
Although we have no direct evidence of what happened at HSE 9,500 years ago to lead to site abandonment, life on a small depauperate island like Kisar would have been precarious. If the population increased, resource depletion of marine staples may have occurred, and freshwater sources are likely to have been strained or exhausted. Added to this, larger populations would have made rapid relocation to another island in the event of environmental collapse more difficult. If the population remained low, fortuitous accidents and random factors acting to unbalance the demographic profile, may have rendered it unviable over the long term.
HSE was reoccupied in the mid-Holocene at which time there appears to have been a change in raw materials used for stone artifact manufacture as well as in the range of fish caught. Quartz dominates the stone artifact assemblage in Phase 2 and obsidian is absent above Spit 7 in Square A and Spit 5 in Square B, perhaps indicating a tighter focus on locally procurable resources and the cessation of the obsidian exchange network during the late Holocene occupation. Aside from these changes there is continuity in terms of the range of resources exploited and the types and methods of manufacture of shell hooks and items of personal decoration.
CONCLUSION
The finds in the HSE shelter demonstrate that the small island of Kisar was occupied by at least 15,500 years ago. That the shelter was available to be occupied earlier, by ∼40,000 cal BP, is demonstrated by the dating of the emerged coralline terrace underlying the occupation deposit. Kisar is extremely depauperate in terrestrial fauna and protein was acquired almost exclusively from marine resources. Plant foods would have been critical to complement the largely marine diet, however, we currently have no insight into what these may have been. Exploitation of the waters surrounding the island was facilitated by the use of finely made fishhooks with most fishing focused on small to medium fish from the inshore reef zone. Crustaceans, shellfish, and urchins were also dietary staples collected from the rocky shoreline or coral reef. While we cannot be sure that settlement on Kisar was ‘permanent’, the dense material culture signature in HSE shelter suggests sustained and intensive occupation during the terminal Pleistocene and early Holocene when compared with other coastal sites on larger neighboring islands such as Timor and Alor. However, the presence of obsidian artifacts, a raw material not available on Kisar, demonstrates that at least some members of the population were making maritime voyages to obtain this high-quality raw material or, alternatively, were engaged in exchange with visitors from another island. While we do not yet know the source of the obsidian, it is not derived from Timor, the closest island to Kisar. Despite the inherent dangers involved in maritime crossings, this interaction network would have constituted a risk buffering strategy, providing relationships of reciprocity which were vital to ensuring that the population could relocate in the event that water shortage or catastrophic damage to marine resources rendered the small island of Kisar uninhabitable. Some such catastrophe may have occurred around 9,500 years ago when the HSE shelter ceased to be used.
Why a hiatus occurred at HSE during the early to mid-Holocene is currently unclear and it is also uncertain whether occupation of the island or only the shelter ceased at this time. Future survey and excavation work on Kisar will hopefully provide some clarification on this. HSE was reoccupied approximately 5,000 years ago and continued in use until about 1,800 years ago. The obsidian markers of inter-island synergies are not present during the mid- to late Holocene habitation, when all forms of occupation evidence are generally less dense. Pottery appears in the upper spits (see Supplementary Information: S2) and it seems likely that this marker of the Neolithic signals the beginning of sedentary village life on the island. After 1800 cal BP HSE was little used, but the presence of a few glass flakes close to the surface shows that the site was still occasionally visited into the European contact period.
Kisar represents a unique regional record for Pleistocene occupation of very small islands, allowing us to examine the dynamics behind initial settlement and use of such environments. While it is impossible to determine whether the first landfall on Kisar was passive or strategic, given that exotic obsidian is found throughout the first phase of occupation, repeat voyages to the island must have been in some way regionally strategic. On the basis of the production of shell artifacts at the site, the occupation of HSE, and thus Kisar, was also most probably permanent during this phase. However, on the basis of its size, locality, and available terrestrial resources, Kisar represents an extremely marginal island—not obviously appealing for Pleistocene human occupation. That people did occupy the island successfully for thousands of years speaks to the ability of people to overcome the twin insular constraints of distance (from a larger landmass) and size (in terms of total habitable area). However, the archaeological record of Kisar also demonstrates that continual subsistence on the island was not possible without the co-occurrence of advanced maritime technologies and a vibrant exchange network. The former was clearly present in Wallacea for thousands of years before HSE was settled, as evidenced by the number and types of maritime artifacts preserved on both Timor and Alor (O'Connor et al. Citation2011, Citation2014, Citation2017). But it was only with the introduction of an exchange network approximately 15,000 years ago, with concomitant economic and/or familial ties with neighboring islands, that the ecological constraints of small island living were broken, and that Kisar was able to be successfully occupied.
uica_a_1443171_sm8672.xlsx
Download MS Excel (37 KB)uica_a_1443171_sm8671.docx
Download MS Word (175.4 KB)ACKNOWLEDGEMENTS
This research was funded through an ARC Laureate Fellowship to Sue O'Connor (FL120100156) and the Centre of Excellence for Australian Biodiversity and Heritage CE170100015. The research in Indonesia was carried out on a FRP visa granted by RISTEK; O'Connor #1456/FRP/SM/VII/2015. We also thank the UMR7209 laboratory (Muséum National d'Histoire Naturelle) and Philippe Béarez for giving us access to the fish osteological reference collection and for his valuable advice.
Additional information
Funding
REFERENCES
- Agustiyanto, D. A., M. Suparman, E. Partoyo, and D. Sukarna. 1994. Peta Geologi Lembar Moa, Damar dan Bandanaira, Maluku. Bandung, Indonesia: Pusat Penelitian dan Pengembangan Geologi.
- Alifah. 2016. Sumberdaya Tumbuhan dan Pemanfaatannya di Situs Gua Here Sorot Entapa dan Kuil Eu Lapa, Pulau Kisar, Maluku: Berdasarkan Studi Arkeobotani. M.A. Thesis. Departemen Arkeologi. Yogyakarta: Universitas Gadjah Mada.
- An, X., H. Lu, and G. Chu. 2015. Surface soil phytoliths as vegetation and altitude indicators: a study from the southern Himalaya. Scientific Reports 5:15523.
- Anderson, A. 2018. Ecological contingency accounts for earliest seagoing in the Western Pacific Ocean. The Journal of Island and Coastal Archaeology 13(2):224–234. doi:10.1080/15564894.2016.1277286.
- Barham, A. J. 2000. Late Holocene maritime societies in the Torres Strait Islands, northern Australia – cultural arrival or cultural emergence? In East of Wallace's Line: Studies of Past and Present Maritime Societies in the Indo-Pacific Region. Modern Quaternary Research in Southeast Asia 16 (S. O'Connor, and P. Veth, eds.):223–314. Rotterdam: A.A. Balkema.
- Bellwood, D. R. 1994. A phylogenetic study of the parrotfishes family Scaridae (Pisces: Labroidei), with a revision of genera. Records of the Australian Museum (Suppl.) 20:1–86.
- Boulanger, C. 2014. Etude des comportements de subsistence d'un site austral-mélanésien: Bubog I (île d'Ilin, Mindoro, Philippines). Ichtyofaune, crustacés décapodes et grands mammifères. M.S. Thesis. Paris: Muséum National d'Histoire Naturelle.
- Bowdery, D. 1999. Phytoliths from tropical sediments: Reports from Southeast Asia and Papua New Guinea. Bulletin of the Indo-Pacific Prehistory Association 18:159–168.
- Bowdler, S. 1995. Offshore islands and maritime explorations in Australian prehistory. Antiquity 69(266):945–958.
- Bowdler, S. 2015. The Bass Strait Islands revisited. Quaternary International 385:206–218.
- Butler, V. L. 1994. Fish feeding behaviour and fish capture: The case for variation in Lapita fishing strategies. Archaeology in Oceania 29:81–90.
- Campos, F. Z. 2009. The Ichthyoarchaeology of Batanes Islands, Northern Philippines. M.S. Thesis. Quezon City: University of the Philippines Diliman.
- Cannon, D. Y. 1987. Marine Fish Osteology: A Manual for Archaeologists. Publication Number 18. Burnaby, British Columbia: Simon Fraser University.
- Claassen, C. 2000. Quantifying shell: Comments on Mason, Peterson, and Tiffany. American Antiquity 65(2):415–418.
- Dharma, B. 1988. Siput Dan Kerang Indonesia I. Jakarta, Indonesia: Sarana Graha.
- Dharma, B. 1992. Siput Dan Kerang Indonesia II. Wiesbaden: Verlag Christa Hemmen.
- Dharma, B. 2005. Recent and Fossil Indonesian Shells. Hackenheim, Germany: ConchBooks.
- Direktorat Pendayagunaan Pulau-Pulau Kecil. 2012. Pulau Information: Pulau Kisar. Jakarta: Kementerian Ketautan dan Perikarian, Ditjen Kelautan, Pesisir dan Pulau-Pulau Kecil. http://www.ppk-kp3k.kkp.go.id/direktori-pulau/index.php (accessed September 15, 2017).
- Draper, N. 1991. Cape du Couedic Rockshelter and the Aboriginal Archaeology of Kangaroo Island, South Australia. Ph.D. Dissertation. Albuquerque: University of New Mexico.
- Evans, N. 2005. East across a narrow sea: Micro-colonization and synthetic prehistory in the Wellesley Islands, Northern Australia. In Linguistics, Archaeology and the Human Past (T. Osada, ed.):9–40. Kyoto, Japan: Indus Project, Research Institute for Humanity and Nature.
- Finsch, O. 1898. On seven new species of Birds in the Leyden Museum from the islands of Wetter, Kisser, Letti and New-Guinea. Notes from the Leyden Museum 20(2/3):129–136.
- Fitzpatrick, S. M., C. M. Giovas, and O. Kataoka. 2011. Temporal trends in prehistoric fishing in Palau, Micronesia over the last 1500 years. Archaeology in Oceania 46(1):6–16.
- Fitzpatrick, S. M., V. D. Thompson, A. S. Poteate, M. F. Napolitano, and J. M. Erlandson. 2016. Marginalization of the margins: The importance of smaller islands in human prehistory. The Journal of Island and Coastal Archaeology 11(2):155–170.
- Froese, R. and D. Pauly (eds.) 2010. FishBase: A Global Information System on Fishes. Los Baños: FishBase Information and Research Group, Inc. www.fishbase.org (accessed February 2017).
- Gregory, W. K. 1933. Fish skulls: A study of the evolution of natural mechanisms. Transactions of the American Philosophical Society 23(2):75–481.
- Hall, R. 2002. Cenozoic geological and plate tectonic evolution of SE Asia and the SW Pacific: Computer-based reconstructions, model and animations. Journal of Asian Earth Sciences 20(4):353–431.
- Hawkins, S., S. O'Connor, and S. Kealy. 2016. Late Quaternary hominin–bat (Chiroptera) interactions in the Asia-Pacific. Archaeology in Oceania 51(1):7–17.
- Hawkins, S., S. O'Connor, T. R. Maloney, M. Litster, S. Kealy, J. N. Fenner, K. Aplin, et al. 2017a. Oldest human occupation of Wallacea at Laili Cave, Timor-Leste, shows broad-spectrum foraging responses to late Pleistocene environments. Quaternary Science Reviews 171:58–72.
- Hawkins, S., S. C. Samper Carro, J. Louys, K. Aplin, S. O'Connor, and Mahirta. 2017b. Human palaeoecological interactions and owl roosting at Tron Bon Lei, Alor Island, Eastern Indonesia. The Journal of Island and Coastal Archaeology doi:10.1080/15564894.2017.1285834.
- Hiscock, P. 2002. Quantifying the size of artefact assemblages. Journal of Archaeological Science 29:251–258.
- How, R. A., L. H. Schmitt, and A. Suyanto. 1996. Geographical variation in the morphology of four snake species from the Lesser Sunda Islands, eastern Indonesia. Biological Journal of the Linnean Society 59(4):439–456.
- Jones, R. 1977. Man as an element of a continental fauna: The case of the sundering of the Bassian bridge. In Sunda and Sahul: Prehistoric Studies in Southeast Asia, Melanesia and Australia (J. Allen, J. Golson, and R. Jones, eds.):317–386. London: Academic Press.
- Keegan, W. F., S. M. Fitzpatrick, K. S. Sealey, M. J. LeFebvre, and P. T. Sinelli. 2008. The role of small islands in marine subsistence strategies: Case studies from the Caribbean. Human Ecology 36(5):635–654.
- Lambeck, K. and J. Chappell. 2001. Sea level change through the last glacial cycle. Science 292(5517):679–686.
- Lambrides, A. B. J. and M. I. Weisler. 2013. Assessing protocols for identifying Pacific Island archaeological fish remains: The contribution of vertebrae. International Journal of Osteoarchaeology 25(6):838–848.
- Lambrides, A. B. J. and M. I. Weisler. 2016. Pacific Islands ichthyoarchaeology: Implications for the development of prehistoric fishing studies and global sustainability. Journal of Archaeological Research 24(3):275–324.
- Lampert, R. J. 1981. The Great Kartan Mystery. Terra Australis 5. Canberra: Department of Prehistory, Research School of Pacific Studies, Australian National University.
- Langley, M. C., S. O'Connor, and E. Piotto. 2016. 42,000-year-old worked Nautilus shell from Jerimalai (Timor-Leste): Evidence for early coastal adaptation in ISEA. Journal of Human Evolution 97:1–16.
- Leach, B. F. 1997. A Guide to the Identification of Fish Remains from New Zealand Archaeological Sites. Wellington: Archaeozoology Laboratory, Museum of New Zealand Te Papa Tongarewa.
- Leppard, T. P. and C. Runnels. 2017. Maritime hominin dispersals in the Pleistocene: Advancing the debate. Antiquity 91(356):510–519.
- Lepsikaar, J. 1994. Introduction to Osteology of Fishes for Paleozoologists, 3rd ed. Göteborg, Sweden: Göteborg University.
- Lyman, R. L. 2008. Quantitative Paleozoology. Cambridge: Cambridge University Press.
- MacArthur, R. H. and E. O. Wilson. 1967. The Theory of Island Biogeography. Princeton, NJ: Princeton University Press.
- Major, J., R. Harris, H. W. Chiang, N. Cox, C. C. Shen, S. T. Nelson, C. Prasetyadi, and A. Rianto. 2013. Quaternary hinterland evolution of the active Banda Arc: Surface uplift and neotectonic deformation recorded by coral terraces at Kisar, Indonesia. Journal of Asian Earth Sciences 73:149–161.
- Marwick, B., C. Clarkson, S. O'Connor, and S. Collins. 2016. Early modern human lithic technology from Jerimalai, East Timor. Journal of Human Evolution 101:45–64.
- Maryanto, I., D. J. Kitchener, and S. N. Prijono. 2005. Morphological analysis of house mice, Mus musculus (Rodentia, Muridae) in Southern and Eastern Indonesia and Western Australia. Mammal Study 30(1):53–63.
- McNiven, I. J. 2015a. Precarious islands: Kulkalgal reef island settlement and high mobility across 700 km of seascape, central Torres Strait and northern Great Barrier Reef. Quaternary International 385:39–55.
- McNiven, I. J. 2015b. Ascendancy of the Tudulgal, central Torres Strait: Socio-political manipulation and domination of an archipelago polity. Journal of Anthropological Archaeology 39:164–180.
- McNiven, I. J. 2016. Increase rituals and environmental variability on small residential islands of Torres Strait. The Journal of Island and Coastal Archaeology 11(2):195–210.
- Monk, K. A., Y. De Fretes, and G. Reksodiharjo-Lilley. 1997. Ecology of Nusa Tenggara and Maluku. Vol. 5 Ecology of Indonesia Series. Hong Kong: Periplus Editions.
- Natus, I. R. 2005. Biodiversity and Endemic Centres of Indonesian Terrestrial Vertebrates. Ph.D. Dissertation. Trier, Germany: Fachdereich Geographie/Geowissenschaften, Universität Trier.
- Nelson, J. S. 2006. Fishes of the World, 4th ed. New York: John Wiley & Sons Inc.
- O'Connor, S., Mahirta, S. C. Samper Carro, S. Hawkins, S. Kealy, J. Louys, and R. Wood. 2017. Fishing in life and death: Pleistocene fish-hooks from a burial context in Alor Island, Indonesia. Antiquity 91:1451–1468.
- O'Connor, S., Mahirta, D. Tanudirjo, M. Ririmasse, M. Husni, S. Kealy, S. Hawkins, and Alifah. In press. Ideology, ritual performance and its manifestations in the rock art of Timor-Leste and Kisar Island, Island Southeast Asia. Cambridge Archaeological Journal 1–17. doi:10.1017/S0959774317000816.
- O'Connor, S., R. Ono, and C. Clarkson. 2011. Pelagic fishing at 42,000 BP and the maritime skills of modern humans. Science 334:1117–1121.
- O'Connor, S., G. Roberston, and K. Aplin. 2014. Are osseous artefacts a window on perishable material culture? Implications of an unusually complex bone tool from the late Pleistocene of East Timor. Journal of Human Evolution 67:108–119.
- Ono, R. 2010. Ethno-archaeology and early Austronesian fishing strategies in near-shore environments. The Journal of the Polynesian Society 119(3):269–314.
- Piperno, D. R. 2006. Phytoliths: A Comprehensive Guide for Archaeologists and Paleoecologists. Lanham: Altamira Press.
- Poplin, F. 1976. A propos du nombre de restes et du nombre d'individus dans les échantillons d'ossements. Cahiers du centre de recherches préhistoriques 5(61):61–74.
- Prawasti, T. S., A. Farajallah, and R. Raffiudin. 2013. Three species of ectoparasite mites (Acari: Pterygosomatidae) infested geckos in Indonesia. HAYATI Journal of Biosciences 20(2):80–88.
- Ramsey, C. B. 2009a. Bayesian analysis of radiocarbon dates. Radiocarbon 51(1):337–360.
- Ramsey, C. B. 2009b. Dealing with outliers and offsets in radiocarbon dating. Radiocarbon 51(3):1023–1045.
- Reepmeyer, C., S. O'Connor, and S. Brockwell. 2011. Long-term obsidian use in East Timor: Provenancing lithic artefacts from the Jerimalai cave. Archaeology in Oceania 46:85–90.
- Reepmeyer, C., S. O'Connor, Mahirta, T. Maloney, and S. Kealy. 2016. Late Pleistocene/early Holocene maritime interaction in Southeastern Indonesia—Timor Leste. Journal of Archaeological Science 76:21–30.
- Regional Physical Planning Project for Transmigration. 1989. The Land Resources of Indonesia: a National Overview. Jakarta: ODA/Ministry of Transmigration.
- Reimer, P. J., E. Bard, A. Bayliss, J. W. Beck, P. G. Blackwell, C. Bronk Ramsey, P. M. Grootes, et al. 2013. IntCal13 and Marine13 radiocarbon age calibration curves 0–50,000 years cal BP. Radiocarbon 55(4):1869–1887.
- Rivaton, J. and P. Bourret. 1999. Les otolithes des poissons de l'Indo-Pacifique. Nouméa: IRD, Documents Scientifiques et Techniques II(2):378.
- Rosendahl, D., S. Ulm, C. Sloss, L. Steinberger, F. Petchey, G. Jacobsen, E Stock, and R. Robins. 2015. Mid-Holocene Aboriginal occupation of offshore islands in northern Australia? A reassessment of Wurdukanhan, Mornington Island, southern Gulf of Carpentaria, Australia. Quaternary International 385:145–153.
- Samper Carro, S. C., J. Louys, and S. O'Connor. 2017. Methodological considerations for icthyoarchaeology from the Tron Bon Lei sequence, Alor, Indonesia. Archaeological Research in Asia 12:11–22.
- Samper Carro, S. C., S. O'Connor, J. Louys, S. Hawkins, and M. Mahirta. 2016. Human maritime subsistence strategies in the Lesser Sunda Islands during the terminal Pleistocene–early Holocene: New evidence from Alor, Indonesia. Quaternary International 416:64–79.
- Tercerie, S., P. Béarez, P. Pruvost, N. Bailly, R. Vignes-Lebbe, L. Bled, S. Grosjean, E. Buenaventes, and K. Jacquez. 2014. Osteobase. World Wide Web electronic publication. www.osteobase.mnhn.fr (accessed February 2017).
- Tindale, N. B. 1977. Further report on the Kaiadilt people of Bentinck Island, Gulf of Carpentaria Queensland. In Sunda and Sahul Prehistoric Studies in Southeast Asia, Melanesia and Australia (J. Allen, J. Golson, and R. Jones, eds.):247–273. London: Academic Press.
- Trainor, C. R. 2003. Recent bird observations from Kisar Island, Lesser Sundas. Kukila 12:54–58.
- Tyler, J. C. 1980. Osteology, phylogeny and higher classification of the fishes of the order Plectognathi (Tetraodontiformes). NOAA Tech. Rept. NMFS Circular 434:1–122.
- Wood, R., Z. Jacobs, D. Vannieuwenhuyse, J. Balme, S. O'Connor, and R. Whitau. 2016. Towards an accurate and precise chronology for the colonization of Australia: The example of Riwi, Kimberley, Western Australia. PLOS ONE 11(9):e0160123.
- Woodroffe, C. D., D. M. Kennedy, D. Hopley, C. E. Rasmussen, and S. G. Smithers. 2000. Holocene reef growth in Torres Strait. Marine Geology 170(3):331–346.
- WoRMS Editorial Board. 2017. World Register of Marine Species. Available from http://www.marinespecies.org at VLIZ (accessed November 17, 2017). doi:10.14284/170.
- Wyneken, J. and D. Witherington. 2001. The Anatomy of Sea Turtles. Miami: US Department of Commerce, Southeast Fisheries Science Center, National Marine Fisheries Service, National Oceanic and Atmospheric Administration.
- Zug, G. R. 2010. An outlying Carila population from Java and comments on species groups within the Genus Carila (Reptilia: Squamata: Scincidae). Proceedings of the California Academy of Sciences 61(7):389.