Abstract
An α-amylase with molecular weight of 73 kDa was purified from midgut of Dociostaurus maroccanus using ammonium sulfate precipitation, gel filtration, and ion exchange chromatography. The optimal pH and temperature were 6 and 45 °C, respectively. As calculated using Lineweaver–Burk plots, the Km was about 0.62 mM and the Vmax was 1.113 (μmol/min/mg protein). Mn2+, Hg+, Zn2+ and ethylene diamine tetraacetic acid (EDTA) decreased α-amylase activity of D. maroccanus, whereas the addition of K+, Na+, Fe2+, Ba+, Mg2+, Ca2+ and Co2+ increased enzyme activity. Alpha-amylase inhibitors (AI1, AI2) with molecular weights of 43 kDa and 29 kDa, respectively, were purified from the seeds of Phaseolus vulgaris and its inhibitory effect on purified α-amylase of D. maroccanus was investigated. These inhibitors inhibited the D. maroccanus gut α-amylase activity significantly.
Introduction
The Moroccan locust, Dociostaurus maroccanus (Thunberg) (Orthoptera: Acrididae) is a polyphagous pest with migratory capability. This pest is distributed throughout Europe, Africa, Central Asia, the Middle East and in northeastern Iran. This insect is a serious pest in wheat, barley, millet, rice and maize grain crops and attack the leaves, stalks and grain of legumes (peas, beans, lentil, alfalfa, clover) (FAO, Citation2010). Crop damage by D. maroccanus has been reported in more than 25 countries (Lachininskii, Citation1989).
Alpha-amylase (a-1,4-glucan-4-glucanohydrolases, EC 3.2.1.1) is an endo-amylase family that catalyzes hydrolysis of α-d-(1,4)-glucan linkages in starch, glycogens, and other carbohydrates (Franco et al., Citation2002; Sharma, Citation2009). Alpha-amylase is a digestive hydrolase involved in energy production in insects (Pelegrini et al., Citation2006). These enzymes are synthesized and secreted by midgut epithelial cells of insects and play an essential role in their digestion and survival (Sharma, Citation2009). Alpha-amylase has been characterized in the digestive systems of insects belonging to orders Orthoptera, Diptera, Hymenoptera, Lepidoptera and Coleoptera (Terra & Ferreira, Citation2005) with the aim of gathering knowledge for development of new methods for pest control.
Considering the importance of food in insect development and physiology and the fact that important control measures employ inhibitors and poisons that interact with digestive juices, investigation of the effects of digestive enzymes on substrates and their inhibition by synthetic and non-synthetic means is necessary (Ghadamyari et al., Citation2010; Kazzazi et al., Citation2005; Ramzi & Hossininaveh, Citation2010; Sharifi et al., Citation2011). Insect digestive enzymes, especially α-amylase, are good targets for inhibitors that exist in plant seeds (Macedo & Freire, Citation2011). Alpha-amylase inhibitors are potential tools for increasing the resistance of crop plants to pests.
Transgenic plants containing α-amylase inhibitors have been produced by plant engineering. These inhibitors, which are abundant in legumes and cereals, are proteins and have a remarkable role in the natural defense against insect pests, especially those that feed on starchy food (Franco et al., Citation2002). Expression of insecticidal proteins such as α-amylase inhibitors in plants are a new strategy for insect control. With the current efforts to decrease the use of pesticides, scientists are looking for new methods of pest control. Investigation of insect digestive enzymes is essential for gathering knowledge to achieve this goal. The current study purified and characterized α-amylase in D. maroccanus and determined the effect of purified Phaseolus vulgaris α-amylase inhibitors on its activity.
Materials and methods
Insects and chemicals
The last instar nymph of D. maroccanus were collected from the cultivated farmlands in Golestan province of Iran and transferred to the laboratory of entomology at the University of Giulan. The synchronized adults were selected for dissecting.
3,5-dinitrosalicylic acid (DNS), triton X-100, bovine serum albumin (BSA) and starch were purchased from Merck (Merck, Darmstadt, Germany). Protein standards, which used for determination of molecular mass of protein on polyacrylamide gel electrophoresis (PAGE), were provided by Bio-Rad (Hercules, CA). All other chemicals and reagents were analytical grade.
Sample preparation and enzyme alpha amylase inhibition assay
Insect adults were randomly selected and immobilized on ice and dissected under light microscope in ice-cold saline buffer. Digestive systems of them were removed and their content was eliminated. Then, the guts were transferred to a freezer (−20 °C). For measuring enzyme activity, the samples were homogenized in cold double-distilled water and/or buffer using a hand-held glass homogenizer and centrifuged at 12 000×g for 15 min at 4 °C.
Alpha amylase activity was determined according to Bernfeld (Citation1955) using dinitrosalisylic acid (DNS) procedure. Ten microliter of supernatant was added to a tube containing 40 μl of the universal buffer (25 mM phosphate, boric-citric acid and glycine) and 50 μl of 1% soluble starch (Merck, Darmstadt, Germany) as the substrate and incubated for 30 min at 37 °C. Eighty microliter of DNS was added and the reaction was heated in boiling water for 10 min. After cooling the reaction in room temperature, the absorbance was measured at 540 nm with a Microplate Reader Stat Fax® Model 3200 (Asadi et al., Citation2010). One unit of amylase activity (IU) is defined as the amount of enzyme that released one μmol of maltose equivalent per min under the mentioned conditions. Amylase specific activity was expressed as units per mg of protein. Protein concentrations were measured as described by Bradford (Citation1976), using BSA as standard.
Purification process
Alpha amylase was purified using the procedures as described in Dennison (Citation1999). The enzyme was purified by three-step:
1. Amonium sulfate treatments: Forty milliliter of crude extract from midgut homogenates of D. maroccanus was precipitated using 75% saturation of ammonium sulfate at 4 °C for 30 min. The precipitates were collected by centrifugation at 10 000 rpm for 10 min. The pellet was dissolved in the citrate buffer (40 mM; pH 6.0) and then dialyzed against the 20 mM phosphate cold buffer (pH 6.0) for 24 h at 4 °C.
2. Sephacryl 100-HR gel filtration: The dialyzed enzyme loaded on a Sephacryl 100-HR column, equilibrated in Bis-Tris buffer (pH 6.0) based on the manufacture procedure. The enzyme was eluted with a flow rate of 0.5 ml/min. Protein concentration in fractions was measured by determining the absorbance at 280 nm and also, the amylase activities in fractions were measured based on the Bernfeld method using 1% soluble starch as substrate as mentioned above.
3. Diethylaminoethyl (DEAE) cellulose: Fractions (obtained from step 2) comprising the highest α-amylase activities were applied to a DEAE ion-exchange chromatography. The enzyme was eluted from ion exchange column using a linear NaCl gradient (0–0.5 M) at a flow rate of 0.5 ml/min. The protein concentration in each eluted fraction (each fraction contain 1.5 ml) was measured at 280 nm using Cary 3 spectrophotometer and also, α-amylase activity in fractions containing protein were measured as mentioned above. The active fractions were used for further analysis.
Denaturing SDS–PAGE
The molecular weights of the purified α-amylase were estimated by SDS–PAGE. SDS–PAGE was performed according to the procedure described by Laemmli (Citation1970). The acrylamide concentration was 10% for the separating gel and 4% for the stacking gel. After the end of electrophoresis in 100 V at 4 °C, the separated protein bands were observed by 0.2% Coomassie brilliant blue R-250 staining. Protein molecular weight was estimated using the protein markers (14.4–116 kDa).
Kinetic parameters of α-amylases
The Michaelis–Menten constant (Km) and maximal velocity (Vmax) of the α-amylase were estimated using different concentrations of starch over the range of 0.05–1.5% % (w/v), in 25 mM citrate buffer (pH 6.0). The Michaelis–Menten constant (Km) and maximal velocity (Vmax) were estimated from the Lineweaver–Burk plots. The kinetic values are the averages of three experiments and standard errors are less than 10%.
Effect of pHs and temperatures on α-amylase activity
The pH profiles of the α-amylases were characterized in a mixed buffer containing phosphate, boric-citric acid and glycine (25 mM of each) adjusted to various pHs (pH 3–12) by adding HCl or NaOH for acidic and basic pH values, respectively. Before determining α-amylase activity, the enzyme and buffer mixtures were incubated at different pH for 5 min. Then the enzyme activity was measured according to the Bernfeld (Citation1955) method as mentioned above. Also, the activity of α-amylase was measured by incubating the reaction mixtures in optimal pH value 25 mM citrate, pH 6 for 5 min at different temperatures ranging from 15 to 65 °C with 10 °C intervals.
Effect of activators and inhibitors on enzyme activity
The effects of various chemicals including Mn2+, Hg+, Zn2+, K+, Na+, Fe2+, Ba+, Mg2+, Ca2+, Co2+ and ethylene diamine tetraacetic acid (EDTA) (20 mM for all the ions) were determined on α-amylase activity. After 30 min of pre-incubation of ions and EDTA with purified enzyme at room temperature, residual activity was measured according to Bernfeld (Citation1955) method as mentioned above.
Purification of α-amylase inhibitors from seeds of P. vulgaris and their inhibition assay
Effects of the seed extract on the purified α-amylase activity of D. maroccanus were investigated. Seeds were milled to a powder and the proteinous inhibitors were extracted with 0.15 M NaCl under continuous stirring for 1 h at 4 °C. The material was then centrifuged at 6,000 × g at 4 °C for 30 min. The supernatant was heated to 80 °C and centrifuged again at 6000 g for 15 min. Inhibitor was purified using gel filtration and ion exchange chromatography as the same steps procedure that was applied to the enzyme purification.
Ten microliters of the D. maroccanus purified α-amylase (protein content = 45 μg) was preincubated with 10 μl of purified inhibitor and 30 μl of citrate buffer (pH 6) for 30 min at room temperature; then the amylase activity was determined by measuring absorbance at 540 nm based on the Bernfeld (Citation1955) procedure as mentioned above.
Estimation of molecular weight of the purified α-amylase inhibitor
The molecular mass of the purified α-amylase inhibitor was determined by denaturing SDS–PAGE with standard protein.
Zymogram analysis and in gel inhibitory assay on α-amylase
Non-denaturing polyacrylamide gel electrophoresis (Native-PAGE) was performed according to the method presented by Laemmli (Citation1970) for zymogram of α-amylase. The samples were mixed with sample buffer and loaded to the polyacrylamide gel (4% and 10% polyacrylamide for the stacking and resolving gels, respectively). Electrophoresis was carried out in 100 V at 4 °C. After electrophoresis, the gel was shacked in 2.5% Triton X-100 for 30 min at room temperature. Then, the gel washed with double distilled water and then by 25 mM of Bis-Tris buffer with pH 6. The rinsed gel was incubated in 1% (w/v) starch solution at 30 °C and shacked for 60 min. After washing the gel with distilled water, it was stained with Lugol solution (I2 1.3% and KI 3%). The bands of α-amylase activity were appeared as bright bands in a dark background and photographed.
Enzyme was pre-incubated with inhibitor for 20 min at 30 °C, and then the remaining α-amylase activity was determined by non-denaturing SDS–PAGE was carried out using the procedures described by Laemmli (Citation1970) as mentioned above.
Statistical analysis
The data were compared by one-way analysis of variance (ANOVA) followed by Tukey's test using SAS program (SAS Institute, Citation1997). All assays were repeated at least three times. When significant differences were found at p ≤ 0.05 and marked in figures with letters.
Results
Purification of α- amylase
The one isoform of D. maroccanus gut α-amylase (iso-enzyme 3) was purified following a three-step procedure and the results obtained after each steps were summarized in . After ammonium sulfate precipitation, samples were subjected to sephacryl 100-HR column (). At this step of the purification process, the fraction number 13 has a specific activity of 2.22 ± 0.07 U/mg protein (). Fractions containing α-amylase activities were used for further purification by DEAE cellulose chromatography. Sephacryl 100-HR fraction containing α-amylase was further fractionated on an ionic exchange DEAE cellulose column. The chromatogram of α-amylase showed two major peaks and four minor peaks (). Assay of peaks revealed that one peak contained α-amylase with total specific activity of 4.8 ± 0.09 U/mg protein (), while the others did not.
Figure 1. (a) Elution profile on sephacryl 100-HR column of midgut crude extract of D. Maroccanus; (b) Chromatogram obtained from ionic exchange DEAE cellulose chromatography of D. Maroccanus midgut extract. (♦) protein absorbance at 280 nm; and (dashed line) NaCl gradient; (c) Analysis of purified α-amylase by SDS-PAGE. Lane a: Crude extract of midgut extract of D. maroccanus, Lane b: purified α-amylase Lane c: Molecular weight of markers: lysozyme (14.4 kDa); lactoglobulin (18.4 kDa); restriction endonuclease bsp 981 (25 kDa); lactate dehydrogenase (35.5 kDa); ovalbumin (45 kDa); bovine serum albumin (66 kDa); α-galactosidase (116 kDa).
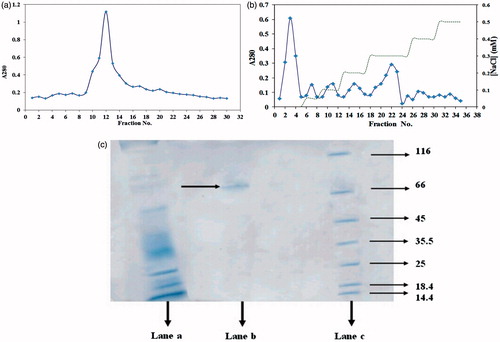
Table 1. Purification of α-amylase from digestive system of D. maroccanus.
Molecular weights of the purified α-amylase
The result of SDS–PAGE of the purified α-amylase is shown in . The single band on the gel was demonstrated and the molecular mass of the purified α-amylase was determined to be 73 kDa by SDS–PAGE ().
Effect of pHs, temperatures, activators and inhibitors on purified enzyme activity
The α-amylases purified from guts of D. maroccanus showed an optimal pH of 6 using soluble starch as substrate (). Purified α-amylase showed the highest activity in a range of 35–55 °C with maximum activity at 45 °C (). The effects of various chemical agents on the α-amylase activity were summarized in . Mn2+, Hg+, Zn2+ and EDTA exhibited the inhibitory effect in a descending order at a final concentration of 0.1, 1, 2 and 5 mM. While, K+, Na+, Fe2+, Ba+, Mg2+, Ca2+ and Co2+ activated the enzyme.
Figure 2. (a) The effect of pHs on the mean relative activities of α-amylase purified from the digestive system of D. maroccanus; (b) The effect of temperatures on the mean relative activities of α-amylase purified from the digestive system of D. maroccanus.
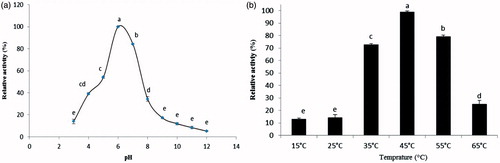
Table 2. Effect of some organic and inorganic compounds on α-amylase activity (iso-enzyme 3) of D. maroccanus..
Kinetics parameters of the purified α-amylase (iso-enzyme 3)
Alpha-amylase showed a Michaelis–Menten type kinetics using soluble starch as substrate at its optimal pH. As calculated from Lineweaver–Burk plots, the Km and Vmax of α-amylase purified from D. maroccanus were 0.620 ± 0.005 mM and 1.113 ± 0.1 μmol/min/mg protein, respectively.
P. Vulgaris α-amylase inhibitor purification
Ammonium sulfate precipitation of inhibitors was further fractionated on sephacryl 100-HR gel filtration () and an ionic exchange DEAE cellulose column (). Assay of the peaks of DEAE cellulose column revealed that two peaks of P. vulgaris strongly inhibited the D. maroccanus gut α-amylase (). The peak numbers 17 and 24 had the highest inhibitory effect on α-amylase activity. The peak number 24 (NaCl concentration 0.3 mM; protein concentration= 1.08 mg/ml) from P. vulgaris inhibited purified α-amylase activity by 48.56 ± 0.57% called AI1 and peak number 17 (NaCl concentration 0.2 mM; protein concentration= 0.94 mg/ml) inhibited 28 ± 0.63%. The peak number 24 from P. vulgaris (AI1) inhibited crud midgut extract (containing three isoforms) by 63.34 ± 0.41%.
Figure 3. (a) Elution profile for inhibitor extracted from seeds of P. vulgaris using sephacryl 100-HR column chromatography; (b) Elution profile for inhibitor extracted from seeds of P. vulgaris using ionic exchange DEAE cellulose as bed, equilibrated with 20 mM Tris-HCl buffer. (♦) protein absorbance at 280 nm; and (dashed line) NaCl gradient; (c) SDS–PAGE of proteins at different process of α-amylase inhibitor purification. Lane a: purified P. vulgaris amylase inhibitor (Fraction 17), lane b: crude plant extract, Lane c: purified P. vulgaris amylase inhibitor (Fraction 24), lane d: Molecular weight markers: lysozyme (14.4 kDa); lactoglobulin (18.4 kDa); restriction endonuclease bsp 981 (25 kDa); lactate dehydrogenase (35.5 kDa); ovalbumin (45 kDa); bovine serum albumin (66 KDa); α-galactosidase (116 kDa); (d) molecular weights-relative mobility of proteins for determination of purified α-amylase inhibitor.
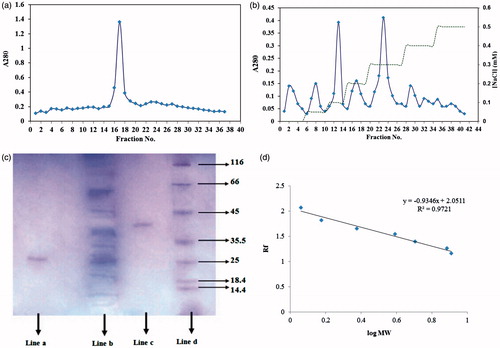
Molecular weight of P. Vulgaris α-amylase inhibitor
The purified amylase inhibitors AI1, AI2 from P. vulgaris as estimated using SDS-PAGE by comparison with standard protein marker showed a molecular weight of 43 kDa and 29 kDa, respectively ().
Zymogram analysis and the inhibitory effect P. Vulgaris on α-amylase
Native gel electrophoresis analysis showed that a mixture of three iso-enzyme α-amylases was present in the gut homogenate of D. maroccanus (). Iso-enzyme three was purified using different technique at this study.
Figure 4. Zymogram of α-amylases extracted from the digestive system of D. maroccanus. Enzyme extract was pre-incubated with P. vulgaris inhibitor (AI1) for 30 min and then gel was run at 4 °C. Lane numbers are as follows: (1) Crude enzyme extract treated with AI1, (2) Crude enzyme extract with no inhibitor, (3) Crude enzyme extract treated with AI2, (4) Purified α- amylase incubated with AI1, (5) Control (Purified α- amylase with no inhibitor), (6) Purified α- amylase incubated with AI2.
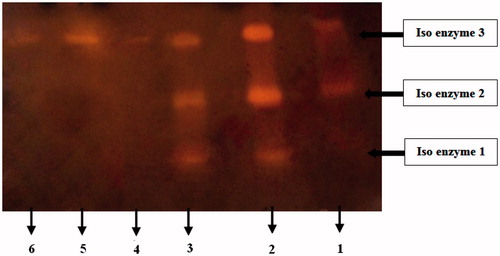
Also, the inhibitory effect of AI1, AI2 on the crud and purified α-amylase activities of D. maroccanus in zymogram studies are shown in .
Discussion
Ammonium sulfate precipitation followed by gel filtration chromatography on sephacryl 100-HR and anion-exchange chromatography on DEAE-sepharose purified one isoform of α-amylase with a molecular weight of 73 kDa. The mean molecular weight of insect α-amylase has been reported to be 28–87 kDa (Terra & Ferreira, Citation1994). SDS–PAGE and zymography revealed three isoforms of amylase in D. maroccanus. The current research purified and characterized one isoform with a molecular weight of 73 kDa. Two isoforms of α-amylase (Amy A1 and A2) with molecular masses of 85–89 kDa and 66–72 kDa, respectively, have been purified from the digestive system of Gryllodes sigillatus (Kouadio et al., Citation2010). The molecular weights of α-amylase purified from Tenebrio molitor larvae (Buonocore et al., Citation1976) and Periplaneta americana have been estimated to be 68 kDa (Ahipo Dué et al., Citation2008), respectively.
Temperature plays a major role in the function of enzymes. D. maroccanus α-amylase showed optimum activity at 45 °C (). The optimum temperature reported for α-amylase activity is 40 °C in the Italian locust, Calliptamus italicus (Orthoptera: Acrididae) (Darvishzadeh & Bandani, Citation2012), 40–50 °C in the midgut of Rhynchophorus ferrugineus (Riseh & Ghadamyari, Citation2012), and 50–60 °C in the digestive system of Callosobruchus maculatus (Wisessing et al., Citation2008). Optimum temperature for α-amylase purified from the midgut of Andrallus spinidens F. (Hemiptera: Pentatomidae) (Zibaee et al., Citation2012) was 35–40 °C and from P. americana was 55 °C (Ahipo Dué et al., 2008).
Maximum α-amylase activity purified from the digestive system of D. maroccanus was observed at pH 6.0 (). The optimal pH of α-amylase in the Italian locust was determined to be about 8.0 (Darvishzadeh & Bandani, Citation2012). The optimum pH for Amy A1 and A2 isolated from the digestive tract of the tropical house cricket, G. sigillatus, was 6.6 for Amy A1 and 7.0 for Amy A2 (Kouadio et al., Citation2010). Each enzyme has specific optimum activity at temperatures and pH conditions; a change in these values changes the protonation pattern and can, in extreme cases, result in denaturation and loss of tertiary structure and produces a decrease in activity.
Insect α-amylase is activated or inhibited by chemicals and ions. The results of the current study showed that Ca2+ significantly increased α-amylase activity purified from the digestive system of D. maroccanus (). Insect α-amylases are Ca2+ dependent. The activity of the α-amylase of G. sigillatus (Kouadio et al., Citation2010), Naranga aenescens (Asadi et al., Citation2010), and Rhyzopertha dominica (Priya et al., Citation2010) all increased with the addition of Ca2+ to the assay mixture. EDTA, Hg+, Mn2+ and Zn2+ decreased D. maroccanus α-amylase activity. EDTA decreased α-amylase activity by removing Ca2+ through chelation and destabilizing of the enzyme (Buonocore et al., Citation1976). EDTA is a general chelating agent that removes all metal ions from active sites of the enzyme.
As calculated using Lineweaver–Burk plots, the Km for D. maroccanus α-amylase was 0.620 ± 0.05 mM. Km values calculated for Amy A1 and A2 of G. sigillatus were 0.29 and 0.5 mg/ml, respectively (Kouadio et al., Citation2010). Km values for α-amylase purified from T. molitor (Buonocore et al., Citation1976) and P. americana (Ahipo Dué et al., Citation2008) were 1.8 and 5.0 mg/ml, respectively. It appears that the Km values for orthoptera are lower than that of the average values reported for α-amylase purified from other insects. A low value for Km means only a small amount of substrate is required to saturate the enzyme and indicates high dependence on the substrate.
Three isoforms of α-amylase were identified in the digestive system of D. maroccanus one isoform with a molecular weight of 73 kDa was purified and shown to have a specific activity of 4.8 ± 0.09 U/mg of protein ( and ). Thus far, 1–8 isoforms of α-amylase have been reported from the digestive systems of insects. The α-amylase from the digestive system of Brachynema germari (Ramzi & Hossininaveh, Citation2010), Xanthogaleruca luteola Mull. (Col.: Chrysomelidae) (Sharifi et al., Citation2011), Eurygaster integriceps Puton (Heteroptera: Scutelleridae) (Kazzazi et al., Citation2005), Sitophilus oryzae (L.) and Tribolium castaneum (Herbst) (Sivakumar et al., Citation2006) show one isoform. Wisessing et al. (Citation2008) showed that C. maculatus α-amylase had one isoform with a molecular weight of 50 kDa. Two isoforms of α-amylase were reported in the digestive system of G. sigillatus (Kouadio et al., Citation2010), N. aenescens L. (Asadi et al., Citation2010) C. italicus (Orthoptera: Acrididae) (Darvishzadeh & Bandani, Citation2012), Helicoverpa armigera (Hubner), Spodoptera litura (F.), Callosobruchus chinensis and Corcyra cephalonica exhibited more than five isoforms (Sivakumar et al., Citation2006). The diversity of isoforms in insect α-amylase has been ascribed to the existence of natural inhibitors in their hosts (Dojnov et al., Citation2008).
Alpha-amylase inhibitors (AI1, AI2) purified from P. vulgaris by sephacryl 100-HR gel filtration and the ionic exchange DEAE cellulose column exerted inhibitory effect on the D. maroccanus α-amylase. Zymogram of the inhibitory effect of AI1 on α-amylase isoforms of D. maroccanus showed that one isoform was completely inhibited and two isoforms were partially inhibited (. AI1 inhibited α-amylase activity 48.56 ± 0.57%.
The adverse effects of synthetic pesticides on humans, natural enemy populations, and the environment has increased interest in transgenic plants that express α-amylase inhibitors for insect pest management strategies. P. vulgaris seeds can be used as a source of α-amylase inhibitor genes for the production and design of inhibitor transgenic crops with resistance to D. maroccanus Modification of crops and the use of digestive enzyme inhibitor genes could open a avenues for development of insect pest management strategies.
Acknowledgements
This study was supported by grant No. 13226 from research deputy of the University of Guilan.
Declaration of interest
The authors report no conflicts of interest. The authors alone are responsible for the content and writing of this article.
References
- Ahipo Dué E, Kouadio JP, Kouakou HT, et al. (2008). Purification and physicochemical properties of amylase from cockroach, Periplaneta Americana (LINNAEUS), for starches saccharification. Afr J Biotechnol 7:2707–16
- Asadi A, Ghadamyari M, Sajedi HR, et al. (2010). Biochemical characterization of midgut, salivary glands and haemolymph α-amylases of Naranga aenescens. Bull Insectol 63:175–81
- Bernfeld P. (1955). Amylase, α and β. Methods Enzymol 1:149–51
- Bradford MM. (1976). A rapid and sensitive method for the quantitation of microgram quantities of protein utilizing the principle of protein-dye binding. Anal Biochem 72:248–54
- Buonocore VE, Poerio Silvano V, Tomasi M. (1976). Physical and catalytic properties of alpha-amylase from Tenebrio molitor L. larvaeα-Amylase from Tenebrio molitor L. Larvae. J Biochem 153:621–5
- Darvishzadeh A, Bandani AR. (2012). Identification and characterization of alpha-amylase in the Italian locust, Calliptamus italicus (Linnaeus, 1758) (Orthoptera: Acrididae). Mun Entomol Zool 7:1028–34
- Dennison C. (1999). A guide to protein isolation. Dordrecht, The Netherlands: Kluwer Academic Publisher
- Dojnov B, Bozic N, Nenadovic V, et al. (2008). Purification and properties of midgut amylase isolated from Morimus funereus (Coleoptera: Cerambycidae). Comp Biochem Physiol 149B:153–60
- FAO. (2010). Available at: http://www.fao.org/ag/locusts-CCA/en/1010/1018/1076/index.html
- Franco OL, Rigen DJ, Melo FR, Grossi-de-Sa MF. (2002). Plant α-amylase inhibitors and their interaction with insect α-amylases structure, function and potential for crop protection. Eur J Biochem 269:397–412
- Ghadamyari M, Hosseininaveh V, Sharifi M. (2010). Partial biochemical characterization of α- and β-glucosidases of lesser mulberry pyralid, Glyphodes pyloalis Walker (Lep.: Pyralidae). C R Biologies 333:197–204
- Kazzazi M, Bandani AR, Hosseinkhani S. (2005). Biochemical characterization of alpha-amylase of the Sunn pest, Eurygaster integriceps. Entomol Sci 8:371–7
- Kouadio EJP, Due EA, Etchian OA, et al. (2010). Purification and characterization of two dimeric α-amylases from digestive tract in the tropical house cricket Gryllodes sigillatus (Orthoptera: Gryllidae). Aust J Basic Appl Sci 4:5241–52
- Lachininskii AV. (1989). Moroccan locust Dociostaurus maroccanus (Thunberg, 1815): a faunistic rarity or an important economic pest? Insect Conserv Diver 2:167–78.
- Laemmli UK. (1970). Cleavage of structural proteins during the assembly of the head of bacteriophage T4. Nature 227:680–5
- Macedo MLR, Freire MDGM. (2011). Insect digestive enzymes as a target for pest control. ISJ 8:190–8
- Pelegrini PB, Murad AM, Grossi-de-Sá MF, et al. (2006). Structure and enzyme properties of Zabrotes subfasciatus alpha-amylase. Arch Insect Biochem Physiol 61:77–86
- Priya S, Kaur N, Gupta AK. (2010). Purification, characterization and inhibition studies of α-amylase of Rhyzopertha dominica. Pestic Biochem Physiol 98:231–7
- Ramzi S, Hosseininaveh V. (2010). Biochemical characterization of digestive α-amylase, α-glucosidase and β-glucosidase in pistachio green stink bug, Brachynema germari Kolenati (Hemiptera: Pentatomidae). J Asia Pacific Entomol 13:215–9
- Riseh N, Ghadamyari M. (2012). Biochemical characterization of α-amylases from gut and hemolymph of Rhynchophorus ferrugineus Olivieri (Col.: Curculionidae) and their inhibition by extracts from the legumes Vigna radiata L. and Phaseolus vulgaris L. ISJ 9:72–81
- Sharma HC. (2009). Biotechnological approaches for pest management and ecological sustainability. New York, NY: CRC Press,. 526 pp
- SAS Institute. (1997). Online doc, version 8.01.Cary, NC: SAS Institute
- Sharifi M, Ghadamyari M, Mahdavi Moghadam M, Saiidi F. (2011). Biochemical characterization of digestive carbohydrases from Xanthogaleruca luteola and inhibition of its α-amylase by inhibitors extracted from the common bean. Arch Biol Sci 63:705–16
- Sivakumar S, Mohan M, Franco OL, Thayumanavan B. (2006). Inhibition of insect pest α-amylases by little and Winger millet inhibitors. Pestic Biochem Physiol 85:155–60
- Terra WR, Ferreira C. (1994). Insect digestive enzymes: properties, compartmentalization and function. Comp Biochem Physiol 109B:1–62
- Terra WR, Ferreira C. (2005). Biochemistry of digestion. In: Gilbert LI, Iatrou K, Gill SS, eds. Comprehensive molecular insect science. Biochem Mol Biol. Vol, 4.Amsterdam: Elsevier, 171–224
- Wisessing A, Engkagul A, Wongpiyasatid A, Chuwongkomon K. (2008). Purification and characterization of C. maculatus α-amylase. Kasetsart J Nat Sci 42:240–4
- Zibaee A, Hoda H, Fazeli-Dinan M. (2012). Purification and biochemical properties of a salivary α-amylase in Andrallus spinidens Fabricius (Hemiptera: Pentatomidae). ISJ 9:48–57