Abstract
Early-life stress (ELS) induces long-lasting changes in gene expression conferring an increased risk for the development of stress-related mental disorders. Glucocorticoid receptors (GR) mediate the negative feedback actions of glucocorticoids (GC) in the paraventricular nucleus (PVN) of the hypothalamus and anterior pituitary and therefore play a key role in the regulation of the hypothalamic-pituitary-adrenal (HPA) axis and the endocrine response to stress. We here show that ELS programs the expression of the GR gene (Nr3c1) by site-specific hypermethylation at the CpG island (CGI) shore in hypothalamic neurons that produce corticotropin-releasing hormone (Crh), thus preventing Crh upregulation under conditions of chronic stress. CpGs mapping to the Nr3c1 CGI shore region are dynamically regulated by ELS and underpin methylation-sensitive control of this region's insulation-like function via Ying Yang 1 (YY1) binding. Our results provide new insight into how a genomic element integrates experience-dependent epigenetic programming of the composite proximal Nr3c1 promoter, and assigns an insulating role to the CGI shore.
Abbreviations
Avp | = | arginine vasopressin |
BPD | = | borderline personal disorder |
CGI | = | CpG island |
ChIP | = | chromatin immunoprecipitation |
Crh | = | corticotropin releasing hormone |
CUS | = | chronic unpredictable stress |
Dusp1 | = | dual specificity phosphatase 1 |
ELS | = | early-life stress |
EMSA | = | electrophoretic mobility shift assay |
Fkbp5 | = | FK506 binding protein 51 |
GC | = | glucocorticoid |
GR | = | glucocorticoid receptor |
GRE | = | glucocorticoid response element |
HPA | = | hypothalamic-pituitary-adrenal |
MDD | = | major depressive disorder |
Pomc | = | pro-opiomelanocortin |
PTSD | = | posttraumatic stress disorder |
PVN | = | paraventricular nucleus |
Sgk1 | = | serum glucocorticoid kinase 1 |
YY1 | = | Yin Yang |
Introduction
Early-life adversity can elicit life-long increases in glucocorticoid (GC) secretion and disruption of the homeostatic mechanisms that regulate the activity of the hypothalamic-pituitary-adrenal (HPA) axis.Citation1 All of these events increase the risk for the development of stress-related diseases, including mood and affective disorders, anxiety disorders, borderline personal disorder (BPD), and posttraumatic stress disorder (PTSD).Citation1,2
Epigenetic mechanisms are increasingly recognized for their role in the dynamic transduction of the effects of changing environments on the genetic blueprint.Citation3 In this regard, DNA methylation has been recently shown to translate social experiences into long-lasting changes in gene expression and the manifestation of distinct phenotypes. This form of ‘molecular plasticity’ is thought to facilitate an organism's capacity to mount an adaptive response through integration of multilayered gene-environment interactions.Citation4
The glucocorticoid receptor gene (NR3C1) encodes a ligand-gated transcriptional regulator that controls endocrine responses to stress as well as metabolism, inflammation, and reproduction.Citation5 The structure of NR3C1 is highly conserved between human, rat,Citation6 and mouseCitation7; strong homologies are also found in multiple 5’untranslated exons in the proximal promoter regions, which produce various mRNA isoforms encoding the same protein.Citation8 Pioneer studies in the rat showed that persistent changes in exon 17 DNA methylation occur as a function of quality of maternal careCitation9; subsequently, childhood trauma,Citation10-14 suicide,Citation15,16 BPD,Citation17-19 and PTSDCitation20-22 were shown to produce similar epigenetic modifications. While most of the latter studies focused on exon 1F, the human homolog of 17, some authors reported upregulation of multiple transcripts after early-life stress (ELS).Citation15,16,22 Comprehensive analysis of the NR3C1 gene in ratCitation23 and humanCitation24 demonstrated broad changes in DNA methylation, histone modifications, and upregulation of multiple exon 1 transcripts in individuals that had experienced adverse events during early life, although the cause-effect relationships of these observations remain unclear.
We previously showed that ELS in mice causes sustained HPA-axis activity and hypomethylation of the hypothalamic arginine vasopressin (Avp)Citation25 and pituitary pro-opiomelanocortin (Pomc) gene.Citation26 Here, we report that ELS induces site-specific hypermethylation of an Nr3c1 control element, which coordinates expression of multiple GR transcripts and overall GR protein in Crh-expressing neurons and thus prevents upregulation of Crh under conditions of chronic stress in adulthood.
Results
ELS upregulates hypothalamic GR expression
In contrast to changes in Avp expression, which appear within days of exposure to ELS,Citation25 increases in hypothalamic GR levels are delayed, becoming first detectable after termination of the stressor. However, like those of Avp, the changes in GR expression persisted for at least 6 months (). Notably, GR expression was not altered in the hippocampus and pituitary, both GR-responsive tissues that are prominent GC negative feedback sites (Fig. S1).
Figure 1. Increased GR expression translates to higher GR transactivation in ELS mice. (A) GR mRNA expression detected by ISH in PVN in mice aged 10 days, 6 weeks, 3 and 6 months (interaction of ELS and age; P = 0.043 by 2-way ANOVA; *P < 0.0001 by univariate F-tests; n = 4–7). (B) Map of the distal (1) and proximal (3 to 12) untranslated exon 1 of the mouse GR promoter region (upper panel). Regulation of GR exon 1 transcripts detected by qPCR in PVN of 3-month old control (Ctrl) and ELS mice. Expression data are normalized to Atpj5 and relative to the expression of the Ctrl group (ELS effect; P < 0.0001 by one-way MANOVA and aver. F-test; *P < 0.005, #P < 0.05 by univariate F-tests; n = 7–9) (C) Intraperitoneally injected corticosterone (Cort; 0.1, 1.0, 10.0 mg/kg) induced GR-responsive genes in PVN of Ctrl and ELS mice as measured by qPCR. Expression data are normalized to Atpj5; fold-induction is shown (interaction of ELS and dose; P = 0.006 by 2-way MANOVA; *P < 0.017, #P < 0.05 by analysis of simple effects; n = 4–8). (D) GR occupancy of regulatory regions of GR target genes analyzed by in vivo ChIP (effect of corticosterone treatment, P < 0.0001; effect of ELS, P = 0.058 by 2-way MANOVA and average F-test; *P ≤ 0.001, #P < 0.05 by univariate F-tests; n = 4–5 ChIPs per group, PVNs of 3 mice were pooled per ChIP analysis). Data are means ± SEM.
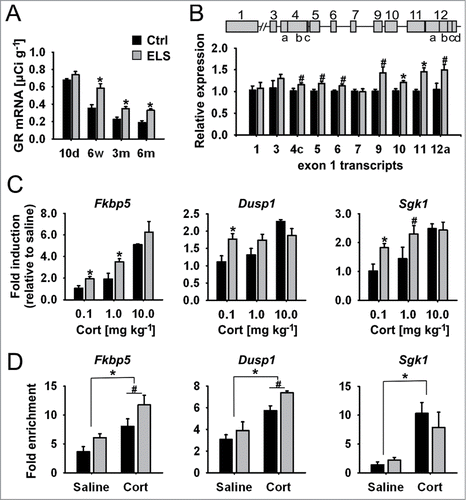
Nr3c1 contains multiple 5′untranslated exons in its promoter region that can give rise to various mRNA isoforms. Although differentially regulated and expressed, these isoforms can be translated into the same protein.Citation7,8 Here we show that multiple GR transcripts derived from the proximal Nr3c1 promoter region are upregulated in the PVN of 3-month-old ELS-treated mice (), consistent with the observed net increase in total GR transcript levels ().
Increased GR expression translates into higher GR transactivation
Corticosterone injections in ELS mice resulted in higher induction of several GC-responsive genes—Fkbp5 (FK506 binding protein 51), Dusp1 (dual specificity phosphatase 1), and Sgk1 (serum glucocorticoid kinase 1) —indicating enhanced transcriptional activity of ELS-upregulated GR (). These target genes play a crucial role in GC signaling, but also in neuronal plasticity,Citation27-30 and encode a maximum of 2 glucocorticoid response elements (GREs).Citation31-33 Results from in vivo ChIP experiments on chromatin derived from microdissected PVN of corticosterone-treated ELS mice revealed higher GR occupancy at the intronic glucocorticoid response element (GRE) of Fkbp5 and at one of the 2 GREs of Dusp1 (GRR-29) (). In summary, the above analysis shows that ELS results in a site-specific upregulation of multiple GR transcripts, a net increase in receptor mRNA, and enhanced transcriptional regulation of target genes.
ELS induces persistent hypermethylation at the CGI shore in Nr3c1
Computational analysis predicted one major and 2 minor CpG-dense islands (CGIs)Citation25 within the proximal promoter of the mouse Nr3c1 (). Since ELS upregulates the expression of multiple GR transcripts, the entire proximal promoter was investigated for experience-induced changes in DNA methylation. This analysis revealed only sparse methylation in ELS-naive mice (), consistent with the view that promoter CGIs, especially those with high CpG content, generally escape DNA methylation.Citation25 Also, no significant differences in DNA methylation of the mouse Nr3c1 proximal promoter (including the rat homolog of exon 17, previously shown to respond to differences in the quality of maternal care),Citation9 were found in PVN tissues from control and ELS mice (Fig. S2).
Figure 2. Methylation of proximal GR promoter and ELS-induced hypermethylation at CGI shore. (A) Map of the proximal untranslated first exons (gray boxes) of the mouse Nr3c1 gene. CpG density, CGIs (red line) and experimentally determined CpG methylation in PVN are shown. Positions within the CpG panel are bordered by blue lines. Methylation of CpGs of the GR promoter region of 3-month old mice was analyzed by sequencing of 15 Alicons (green lines). Moderate CpG methylation was detected at CGI shore region (red box). CpGs are numbered, starting at CpG 1 at -4,732 base pairs (bp) relative to the ATG start codon. (B) Individual methylation of CpG1–9 in PVN of Ctrl and ELS mice aged 10 days, 6 weeks and 3 months (interaction of ELS and age; P = 0.001 by 2-way MANOVA and average F-tests with significance for CpG3, CpG5 and CpG7; ELS effects; *P < 0.006, #P < 0.05 by univariate F-tests; n = 9–10 mice per age). (C) Overall methylation across 9 CpGs at the CGI shore in PVN (interaction of ELS and age; P = 0.013 by 2-way ANOVA; *P < 0.05 by univariate F-tests). Data are means ± SEM.
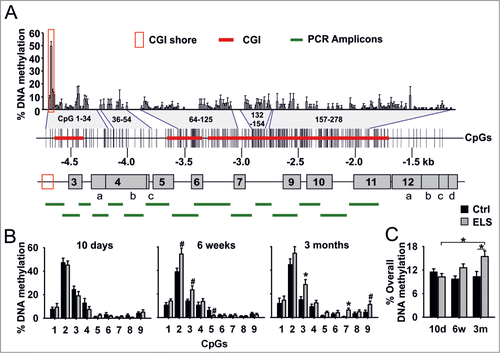
On the other hand, a cluster of moderately methylated CpGs was found adjacent to the most distal CGI (), a topographic region called “CGI shore.”Citation34 Shore regions show a lower CpG content compared to canonical CGIs and frequently undergo DNA methylation.Citation34 Tissue-specific differential methylation was found in CpG1-3 at the Nr3c1 shore region in samples originating from different embryonic lineages (Fig. S3), a finding consistent with the view that CGI shore regions contribute to tissue-specific differences in gene expression.Citation34,35 Accordingly, we were prompted to investigate whether ELS programs Nr3c1 by targeting its shore region.
Methylation of CpGs increased with age (specifically, at CpGs 2 and 3 in 6-week old ELS mice, and CpGs 3, 7 and 9 in 3-month old ELS mice). Importantly, hypermethylation of CpG3 was robustly maintained () and resulted in gradual overall hypermethylation and age-related increases in GR mRNA expression in ELS mice ().
The above results demonstrate that mouse Nr3c1 contains a CGI shore region that can be differentially methylated in a tissue-specific fashion; this CGI shore region serves as a template for ELS-induced hypermethylation.
Methylation-sensitive YY1 binding to the Nr3c1 CGI shore region
DNA methylation is thought to hinder binding of transcription factors to DNA and, at the same time, to favor the recruitment of protein complexes that promote an inactive chromatin structure.Citation4 Computational analysis of the CGI shore region predicted a canonical binding site for the multifunctional and ubiquitously-expressed zinc finger transcription factor YY1 which straddles the ELS-responsive residue CpG3. As in the human NR3C1 promoter,Citation36 2 additional YY1 binding sites are present in the CGI, but these either do not contain a CpG dinucleotide or are poorly methylated and unresponsive to ELS (Fig. S2).
Binding of YY1 at the shore region was assessed by electrophoretic mobility shift assays (EMSA) on nuclear extracts from YY1-transfected cells and using oligonucleotides spanning either the wild type sequence or a mutation of the ELS-responsive CpG3 residue. Specific and strong binding of YY1 to the wild type sequence was revealed by competition and supershift experiments (Fig. S4A). In addition, mutational analysis demonstrated that YY1 binding critically depends on the central CpG3 dinucleotide (Fig. S4B). Confirming the importance of CpG3 in methylation-sensitive YY1 binding, in vitro methylation of wild type and CpG2 mutated oligonucleotides impaired YY1 binding to similar extents ().
Figure 3. Methylation-sensitive YY1 binding to CGI shore. (A) Schematic view of GR wild type (wt) and CpG2 mutated probe (mut TpG2; upper panel). Representative EMSA shows methylation-sensitive binding of YY1 to in vitro methylated (m) CpG3 at the wt and mut TpG2 probe (lanes 1–4). YY1 binding to 32P-labeled probes was competed with a fold5- molar excess of unmethylated and methylated wt and mut TpG2 unlabeled competitors (comp.) (lanes 5–8; lower panel). (B) Methylation levels of GR CGI shore region of N6 cells after treatment with increasing concentrations of 5-azacytidine (5-aza). (C) Relative values of binding of YY1 to CGI shore region by ChIP in N6 cells either treated with DMSO or increasing concentrations of 5-aza (*P < 0.05 by one sample t-test; n = 4 experiments). (D) Methylation of non-enriched and YY1-immunoprecipitated (αYY1) chromatin was analyzed by single clone bisulfite sequencing (*P < 0.05 by paired t-test; n = 3 ChIPs; PVN tissues from 2–3 mice were pooled for each ChIP). Data are means ± SEM.
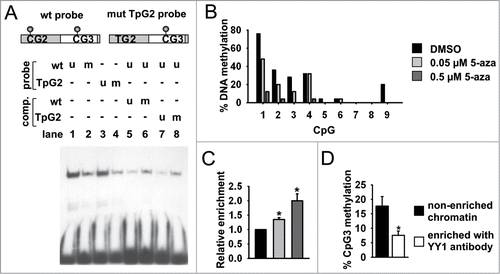
The GR-expressing N6 mouse hypothalamic cell line displays a methylation profile at the shore region that, with the exception of CpG1, resembles the one observed in the mouse PVN (). Treatment of these cells with 5-azacytidine, a potent inhibitor of DNA methylation, reduced the level of methylation at the CGI shore () and concomitantly increased YY1 occupancy, as evidenced by ChIP experiments () in which an antiserum specifically directed against YY1 was used (Fig. S5). Bisulfite sequencing of YY1-immunoprecipitated DNA recovered from the PVNs of ELS-naive mice corroborated methylation-sensitive DNA binding of YY1 in vivo (). These observations indicate that low CpG methylation levels favor YY1 binding to the CGI shore region.
YY1 occupancy at the CGI shore represses Nr3c1 transcription in an ELS-responsive mode
Our finding that GR expression simultaneously increases with CpG3 hypermethylation () and methylation-sensitive YY1 binding to CpG3 () suggests that YY1 occupancy of this region confers transcriptional repression. Previous work has shown that, depending on the cellular and promoter context and available binding partners, YY1 can act as either an activator or repressor of transcription.Citation37 Here, transfection assays in hypothalamic N6 cells revealed that insertion of the CGI shore region reduces promoter activity by 40% compared to the parent vector and that this effect can be partly reversed by a point mutation of CpG3 at the YY1 binding site (). Similarly, knockdown of YY1 in N6 cells enhanced reporter activity, strengthening the evidence that YY1 has a repressor function at the CGI shore region (; Fig. S6).
Figure 4. YY1 repressor binding is selectively reduced by ELS. (A) Relative values of repressive activity of the CGI shore region containing wt or a C-to-T mutation of CpG3 (mut) in N6 cells in a reporter assay (*P = 0.01 by paired t-test, n = 4 experiments) (B) Effect of YY1 and scramble (sc) siRNA on repressive activity of CGI shore region in N6 cells (*P < 0.05 by one sample t-test; n = 4 experiments). (C) Sequential ChIP analysis shows presence of H3ac, H3K9me2 and H3K27me3, Kdm5a, Ehmt2 and Hdac2 at the CGI shore after first ChIP step (n = 3 ChIPs; PVN tissues from 2–3 mice were pooled for each ChIP). (D) Subsequent ChIP with YY1-specific antiserum reveals enriched recovery of chromatin, which was precipitated with antibodies against H3K9me2 and H3K27me3, Kdm5a, Ehmt2, and Hdac2. (E) Insulation activity of Nr3c1 CGI shore. The CGI shore region was inserted in both orientations between the γ-globin promoter (γ) and the β-globin enhancer HS2 (E) that drives expression of a neomycin resistance gene (Neo). Arrows indicate orientation and coverage of the region. The presence of insulator activity diminishes the effect of the enhancer on Neo expression and the formation of viable cell colonies in the presence of neomycin. An interposed core sequence of the insulator of the chicken β-globin locus (cHS4) served as a positive control for efficient insulation. Insertion of the CGI shore sequence in either orientation reduced the number of viable colonies (*P < 0.05 by one sample t-test). Mutation of the YY1 binding site negated insulation, consistent with the idea that the CGI shore function is subject to regulation by YY1 (#P < 0.05 by paired t-test, n = 4 experiments). (F) YY1 expression in the PVN of control and ELS mice. Representative immunoblot of 3 independent experiments. Molecular weight marker (kDa) indicated. (G) YY1 occupancy at the -6 kb region, the distal (d) YY1 binding site and the proximal (p) site of the GR promoter was analyzed in Ctrl and ELS mice by in vivo ChIP (ELS effect on region-specific binding; P = 0.016 by one-way MANOVA; * P < 0.01 by univariate F-test; n = 5 ChIPs per group; PVN tissues from 3 mice were pooled for each ChIP). Data are means ± SEM.
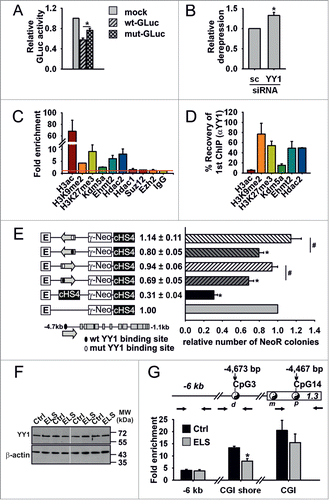
To directly assess YY1s transcriptional function at the CGI shore, we conducted sequential in vivo ChIP experiments, using antisera directed against histone modifications and proteins [namely, H3ac,Citation38 H3K27me3, H3K9me2,Citation39,40 Hdac1, Hdac2,Citation41 Suz12,Citation42 Ezh2,Citation39 and Ehmt2/Ehmt1 (alias G9a/GLP),Citation40 which interacts with Kdm5a (alias Jarid1a)]Citation43 that associate and interact with YY1 to maintain transcriptional repression. Besides observing co-precipitation of YY1 with the repressive histone marks H3K9me2 and H3K27me3 at the Nr3c1 CGI shore, we also found that Ehmt2, Kdm5a, and Hdac2 co-precipitate with YY1. These findings suggest that these proteins may contribute to the repression of Nr3c1 through posttranslational modifications of histones ().
The fact that ELS-induced upregulation of multiple GR transcripts in the PVN of ELS mice () raised the possibility that the CGI shore region regulates transcription across the entire proximal Nr3c1 promoter and, furthermore, that YY1 binding might affect this function. We therefore next investigated whether the CGI shore region shields Nr3c1 promoter activity from upstream regulatory influences. Using a well-established assay system,Citation44 we detected an insulation-like activity of the CGI shore region (). Notably, this control function was largely abolished after mutation of the YY1 DNA-binding site, supporting the view that YY1 plays a critical role in the regulation of the proximal Nr3c1 promoter.
The binding of YY1 at the CGI shore region was further assessed in the PVN of control and ELS mice. While immunoblot analysis did not reveal changes in YY1 expression () in vivo ChIP studies showed region-specific reduction of YY1 occupancy at the CGI shore region in ELS vs. control mice (), consistent with the observed hypermethylation of CpG3 in ELS animals () and the methylation-sensitive mode of YY1 binding to DNA ().
Adult chronic stress differentially regulates hypothalamic Crh expression
The PVN is a major site of GC-mediated negative feedback regulation through Avp and Crh, the primary neuropeptidergic drivers of the HPA axis.Citation5 Since GR expression was upregulated by ELS, we were prompted to compare the effects of an acute injection of corticosterone on Crh expression in ELS vs. control mice. While corticosterone did not significantly inhibit Crh mRNA expression in either treatment group (), it should be recalled that corticosterone nevertheless induces other canonical Nr3c1 target genes (). The lack of effects on Crh are consistent with the results of other studies showing that basal or stimulated Crh transcription in vivo are barely affected by alterations of the corticosterone milieu over a broad range of concentrations.Citation45
Figure 5. Glucocorticoids and chronic stress differentially regulate Crh expression. (A) Effect of intraperitoneally injected corticosterone (Cort; 0.1, 1.0, 10.0 mg kg-1) on Crh expression in PVN of Ctrl and ELS mice as measured by qPCR. Expression data are normalized to Atpj5. (B) Representative immunohistochemical images showing GR, Crh and Avp staining on PVN sections from Ctrl, ELS, CUS and ELSxCUS mice. Scale bar = 50 μm. (C) Avp, Crh and GR mRNA detected by ISH (interaction of ELS and CUS; P = 0.004 by 2-way MANOVA; *P ≤ 0.001 by univariate F-tests; n = 7–15). Data are means ± SEM.
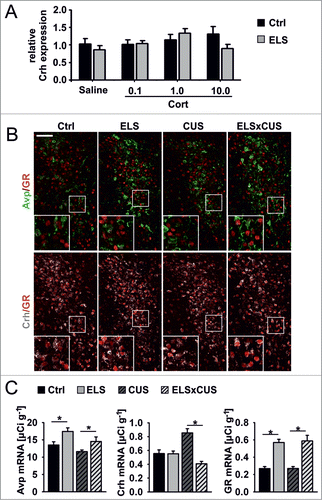
The parvocellular division of the PVN harbors distinct sub-populations of peptidergic neurons, among them neurons that express only Crh or Avp, or both.Citation46-48 Here we used triple fluorescence immunohistochemistry to identify which subsets of PVN neurons express GR. GR expression was confined to Crh neurons in control mice and ELS mice, although expression levels were significantly higher in the latter group (; Fig. S7).
Corticosterone application failed to repress Crh in ELS and control mice (), whereas acute stress triggered an enhanced glucocorticoid response in ELS miceCitation25 (Fig. S8). Together, these findings suggest that higher GR expression in the PVN of ELS mice does not inhibit HPA-axis activity following acute surges in glucocorticoids. This prompted us to investigate the consequences of ELS-induced GR upregulation on Crh expression after exposing animals with a history of ELS to chronic stress in adulthood. Since chronic stress has been consistently reported to upregulate hypothalamic Crh mRNA expression in adult rodents,Citation5 we predicted results that would reflect enhanced inhibitory control of Crh in ELS mice because of their higher levels of GR expression (cf. ), i.e., Crh mRNA levels would be higher in non-ELS animals than in ELS-treated animals when exposed to a chronic unpredictable stress (CUS) paradigm. As shown, however, Crh expression was attenuated in ELSxCUS vs. ELS mice (); this is remarkable because mice with a history of either ELS alone or ELSxCUS expressed significantly higher levels of GR mRNA as compared to control and CUS animals (); again, GR expression was restricted to Crh neurons in CUS and ELSxCUS mice (; Fig. S7). Moreover, consistent with these Crh expression profiles, ELSxCUS and control mice showed similar levels of blood corticosterone at 30 min after an acute stressor, responses that were significantly lower than those observed in animals exposed to either ELS or CUS alone. In addition, baseline corticosterone levels were fastest restored to baseline after the acute stressor in ELSxCUS mice, indicative of their more efficient GC negative feedback mechanisms (Fig. S8).
Taken together, these data show that ELS occludes the normal upregulation of Crh expression when mice are exposed to chronic stress during adulthood.
Discussion
The present work shows that ELS programs Nr3c1 expression by site-specific hypermethylation at the CGI shore in parvocellular Crh-positive neurons of the hypothalamus and prevents Crh upregulation by subsequent exposure to chronic stress.
Most studies on epigenetic programming of GR expression have focused on the promoter region upstream of exon 17 (rat), 1F (human) or the mouse orthologous region;Citation9,16,49-53 in addition, some authors have reported changes in the expression of multiple GR transcript variants.Citation15,23 Although a large proportion of the Nr3c1 locus appears subject to epigenetic regulation,Citation23,24 genomic regions that regulate multiple Nr3c1 promoters within the CGI have not been described hitherto.
Recent in-depth methylation analysis of the whole genome in diverse human tissues showed that only approximately 20% of autosomal CpGs are subject to dynamic epigenetic modifications.Citation35 These residues localize more distal to the regions that are usually studied and presumably harbor genomic elements involved in tissue-type differentiation. This study also revealed that CGI shores, which are often differentially methylated in tissues derived from different lineages, were among those genomic regions that showed the greatest variation in epigenetic marking during normal development.Citation34
Two key findings of the present study are that CpGs mapping to the Nr3c1 CGI shore region i) underpin methylation-sensitive control of this region's insulation-like function via YY1 binding and ii) are dynamically regulated by ELS. Collectively, our results provide new insight into a genomic element integrating experience-dependent epigenetic programming of the composite proximal Nr3c1 promoter and assign to the CGI shore a new role in insulation.
Administration of corticosterone led to transactivation of a set of canonical GR target genes in the hypothalamus of ELS mice, indicating the transcriptional potency of the GR upregulated by ELS. The genes activated in this paradigm included Fkbp (role in an intracellular feedback loop terminating GR function),Citation27 Sgk1 (promotes nuclear localization of GR and prolongs its activation in the absence of GC, upregulates ion channels, enzymes, and transcription factors that modulate hormone release, neuroexcitabililty, and cell proliferation)Citation28,30 and the serine-threonine phosphatase Dusp1, alias MKP-1 (triggers a decrease in phosphorylation-activated ERK1/2 MAPKs and CREB-dependent transcription of brain-derived neurotrophic factor).Citation29 The products of all of these genes are implicated in stress-related affective disorders;Citation28,29,54 however, it is unclear as to whether they play a role in the hypothalamic control of ELS-induced stress responses.
Given that CRH is the major neuropeptidergic driver of the endocrine response to stress and that chronic stress (CUS) during adulthood upregulates hypothalamic Crh expression (), the finding that Crh expression was not elevated in adult mice with a history of ELS was unexpected (). These divergent responses most likely reflect the recruitment of specific physiological mechanisms by the different types of stressor, context and age at which they were imposed.Citation2,55,56 This interesting observation suggests that ELS buffers against subsequent lifetime stressors, a phenomenon also recently reported in rodents and humans.Citation57-59
Glucocorticoids inhibit hypothalamic synthesis and secretion of hypothalamic CRH, their actions being mediated by GR.Citation5 Intriguingly, ELS and ELSxCUS mice showed control-like levels of Crh expression despite their contemporaneously upregulated levels of GR (). Nevertheless, ELS mice were still able to secrete increased amounts of corticosterone (ELS > control and ELSxCUS) when exposed to an acute stressor (Fig. S8). This result indicates that the integrity of the neural mechanisms governing pituitary-adrenal function are maintained in ELS mice and hints at compensatory mechanisms governing HPA-axis function in these animals. In contrast, the finding of control-like corticosterone secretion in ELSxCUS animals suggests that the initial stressor (ELS) attenuates the impact of subsequent CUS through mechanisms that still await elucidation.
Since stressors and the response they elicit are context-dependent and differ in quality as alluded to above, we examined whether Crh expression in ELS mice is subject to inhibition by exogenous corticosterone; the latter paradigm bypasses the complex pathways activated by stress. Contrary to expectation, especially in light of the above-mentioned corticosterone-driven transactivation of GR in ELS mice (), injection of corticosterone failed to significantly suppress Crh expression (). This unanticipated finding concurs with another recent report.Citation45 Together with the present observation that GR-mediated negative feedback is less efficient (delayed) in ELS mice (Fig. S8), these findings suggest that GR regulation of the Crh gene (specifically, in contrast to Fkbp, Sgk1, and Dusp1) is persistently altered by ELS, the molecular underpinnings of which warrant future analysis. One possibility is that enhanced GR expression in ELSxCUS mice facilitates formation of repressive chromatin complexes at the Crh promoter through direct or long-range interactions with enhancer elements.Citation60-62
The long-term effects of early-life experiences appear to be stimulus-specific and dependent on the brain areas that perceive and process the respective stimuli by coordinating downstream cellular and molecular responses, including activation of the epigenetic machinery. In this respect, pioneering studies on differences in the quality of maternal care evidenced hypomethylation of Nr3c1 at exon 17 in the hippocampus and enhanced binding of the transcriptional activator NGFI-A.Citation9 In contrast, we show here that early-life adversity resulted in hypermethylation of Nr3c1 at the shore region in Crh-positive neurons and impaired binding of the transcriptional repressor YY1. Taken together, these findings suggest that epigenetic programming in response to early-life experiences leads to tissue- and cell-type specific effects on Nr3c1 methylation and subsequent alterations in the binding of regulatory proteins conferring transcriptional regulation of Nr3c1.
Materials and Methods
Animal treatments
Maternal separation was used to induce ELS in male C57Bl/6N mice, as described elsewhere.Citation25 A slightly modified version of a uCMS protocol was used.Citation63,64 Two-month-old mice received individual stressors that alternated in order and which were applied at unpredictable times of the day over a period of 4 weeks [during the inactive phase: 1 h shaking of 3–4 mice in a 10 × 10 × 5 cm box at low frequency (∼60 rpm), 30 min restraint stress, 1 h white noise exposure (80 dB) of 3–4 mice placed in a 10 × 10 × 5 cm box; during the active phase for 12 h: tilted cage in a 45° angle, once per week illumination, damped bedding].
Mice were acutely stressed by placing them cage-wise in a plastic box (10 × 10 × 5 cm) that was fixed on a vibrating platform (25 Hz) for 2 minutes. Ten-week old mice received intraperitoneal injections of either saline or corticosterone (0.1, 1.0 or 10.0 mg/kg in 25% cyclodextrin [Sigma-Aldrich, Deisenhofen, Germany]) at the trough of endogenous corticosterone secretion. All procedures on animals were approved by the Regierung von Oberbayern and were in conformity with European Union Directive 2010/63/EU.
In situ hybridization (ISH), quantitative PCR, and bisulfite sequencing
GR transcripts were detected as described.Citation25 Reverse transcribed RNA was quantified using a LightCycler 2.0 and LightCycler FastStart DNA Master plus SYBR Green I Kit (Roche, Mannheim, Germany). Relative expression was calculated using the ΔΔCt method with efficiency correction as described.Citation65 Methylation analysis of genomic DNA (100–200 ng) was performed as described.Citation25 Primers used for qPCR and for bisulfite sequencing are listed in Tables S1 and S2.
Plasmids, cell culture, and transfections
Complementary pairs of single stranded DNA oligomers (Table S3) containing the wild type (wt) or mutated (mut) distal YY1 binding element of the Nr3c1 promoter were cloned into the BamHI and NcoI site of pBluescript II SK+ vector. Transcriptional activity of the CGI boundary sequence (−4,770 to −4,554 bp relative to start codon ATG) was tested in the Gaussia-luciferase (GLuc) expression vector pGl3Gluc. Further details on the generation of constructs described in this manuscript are available on request.
Mouse hypothalamic N6 and N44 cells (Cedarlane CELLutions, Burlington, Canada), culture conditions and 5-azacytidine treatment were described elsewhere.Citation25 N6 cells (2 × 105) were transfected with 4 μg plasmid DNA and 6 μl Turbofect (Thermo Scientific, Schwerte, Germany) per well of a 6 well plate. Relative light units were normalized against β-galactosidase activity from a cotransfected β-galactosidase expression vector.Citation25 Pilot experiments evidenced an efficient knockdown of YY1 mRNA in N6 cells by 50 pmol of YY1 siRNA without obvious effects on viability. Accordingly, 50 pmol YY1 or scramble siRNA (Eurofins MWG Operon, Ebersberg, Germany) were cotransfected with 1 μg GLuc expression vector and 5 μl Lipofectamine 2000 (Life Technologies, Darmstadt, Germany). Sequences were: YY1 sense (5′-ucaggaggugaguucucuc-3′) and antisense (5′-gagagaacucaccuccuga-3′)Citation66 and scramble siRNA sense (5′-agguaguguaaucgccuug-3′) and antisense (5′-caaggcgauuacacuaccu-3′). Cells were harvested for analysis 24–48 h post transfection.
In vitro methylation
Wild type and mutated YY1 DNA-binding sites were cloned into pBSK vectors and sequence verified constructs were in vitro methylated using CpG methyltransferase SssI (New England Biolabs, Frankfurt am Main, Germany) according to manufacturer's instructions. Unmethylated DNA was treated the same, but without SssI. Following phenol/chloroform extraction, completeness of methylation was controlled by digesting both methylated and unmethylated plasmids using methylation-sensitive restriction enzyme TauI.
Electrophoretic mobility shift assays (EMSA)
Double stranded oligonucleotides were obtained by HindIII and NcoI digestion of the corresponding pBSK vector constructs. Fragments were labeled with [α‑32P]‑dCTP and DNA Polymerase I (Klenow) (New England Biolabs, Frankfurt am Main, Germany). Electroporation of LLC-PK1 cells [ATCC CL-101] was used to efficiently express an YY1 expression vector (pCMV-YY1). About 4 μg of nuclear extract was incubated alone or with an access of competitor consensus oligonucleotide (yy1), its mutated form (yy2)Citation67 or with YY1- or pCAF-specific antibody under binding conditions described elsewhere.Citation68 Thereafter, 1 μl of 32P-labeled duplex probes (20,000 cpm) was added to each reaction mix and incubated for 20–25 min at RT. The reaction mixtures were electrophoresed for 1–2 h at 100 V at 12°C in 0.5 x TBE and dried gels were exposed to an MS autoradiography film (Sigma-Aldrich) for 7–10 h at –80°C.
Chromatin immunoprecipitation (ChIP)
ChIP and sequential ChIP experiments on 2–3 × 106 N6 cells and PVN punches from 2- to 3-month old mice were performed as described elsewhere.Citation25 ChIP-qPCR values were normalized relative to the IgG-antibody control (fold enrichment). Relative enrichment was calculated by normalization of fold-enrichment data against the average fold-enrichment of saline-treated control mice. Primers used for ChIP-qPCR analysis are listed in Table S4.
Immunohistochemistry, image acquisition
Brains of 3-month-old mice were PFA-fixed and sucrose cryopreserved. Cryostat sections (30 μm) were thawed, re-fixed in 4% PFA and blocked (5% donkey normal serum, 5% BSA and 0.2% Triton X-100). Primary antibodies were applied for 16 h at 12°C and secondary antibodies for 2 h at room temperature. DAPI staining (10 μg ml−1, Sigma-Aldrich) was performed for 2 min. Primary and secondary antibodies used for triple-staining of GR, Avp and Crh are listed in Table S5. Images were obtained with an Olympus IX81-FV1000 laser-scanning confocal microscope; images (1,024 × 1,024 pixels) were adjusted uniformly for brightness and contrast using FV10-ASW 2.0 software (Olympus).
Antibodies, YY1-specific antisera, immunoblotting
Antibodies are listed in Table S5. YY1 antisera (acc: NP_033563) were raised by Pineda Antibody-Service by injecting New Zealand White rabbits with a GST-conjugated peptide corresponding to the amino acids 1–54. Characterization of YY1-specific antisera was performed by transfection of pRK7-FLAG-YY1 or an equal amount of filling plasmid into LLC-PK1 cells.Citation25 Whole cell protein extracts (20 μg) were tested either with monoclonal FLAG-specific M2 antibody (Sigma-Aldrich) or with YY1-specific antibodies (custom-made, CM1, CM2; H-414 X Santa Cruz Biotechnology, Heidelberg, Germany). Performance of YY1-specific antiserum (CM1) in ChIP experiments was characterized in N44 cells by using the commercial YY1-specific antibody (H-414 X), a negative IgG control, positive and negative control primer sets for verification of enrichment.
Whole cell extracts (50 μg) from PVN punches (2 mice per condition) were immunoblotted and tested with the commercial YY1-specific antibody (H-414 X) or an antibody against β-actin (D6A8 Cell Signaling, Frankfurt/Main, Germany), which served as a loading control.
Insulation assay
Constructs linearized by SalI digest (0.5 μg) were electroporated into 5 × 10Citation6 K562 cells [ATCC CCL-243]. pNI and pNI-CD plasmidsCitation44 served as controls. After 24 h recovery in Improved MEM zinc option (Life Technologies) cells were plated in 0.3% soft agar with 0.9 mg ml−1 G418 sulfate (Merck Millipore, Schwalbach, Germany). Viable colonies were stained with methylthiazolyldiphenyl-tetrazolium (MTT) after 2 weeks and counted. Each construct was tested in duplicate in 4 separate experiments using pooled plasmid DNA derived from 2 independent plasmid preparations.
In silico analysis, statistics
Emboss CpGplot (http://www.ebi.ac.uk/Tools/emboss/cpgplot/) was used to identify CGIs.Citation25 Transcription Element Search System (TESS) was used to predict the YY1 binding site covering CpG3 (http://www.cbil.upenn.edu/cgi-bin/tess/tess).
Quantitative data were analyzed using SPSS software (IBM, Munich, Germany). All numerical data were expressed as mean ± SEM (standard error of the mean). Statistical evaluation was basically performed with analyses of variance (ANOVAs or MANOVAs) followed by univariate F-tests for testing simple effects or contrasts. By multivariate variance analyses main or interaction effects were tested about significance with Wilks multivariate F-tests or averaged F-tests, the last one especially in cases of small samples. GR expression data at different ages and fold-induction data on GR target genes were transformed by using artan-transformation to keep variance homogeneity. Two-tailed paired or unpaired student's t test was used to compare mean differences. One sample t-test was used to compare means to normalized controls of in vitro experiments. An α = 0.05 was accepted as nominal level of significance. All post hoc tests were performed at an adjusted (according to Bonferroni) level of significance, in order to keep the type I error lower or equal to α.
Disclosure of Potential Conflicts of Interest
No potential conflicts of interest were disclosed.
Supplemental_Material.docx
Download MS Word (3.8 MB)Acknowledgments
We gratefully acknowledge the support of A Varga and his team for help with animal care, A Yassouridis for statistical advice and analysis. We thank T Shenk (Princeton University) for providing us plasmids, pGEX2T-YY1 and pCMV-YY1, G Felsenfeld and A West (University of Glasgow) for the insulation vectors pNI and pNI-CD, and C Plank (Technical University of Munich) for K563 cells. We also thank H Gainer (NIH, Bethesda) for the Avp-specific antibody, PS 41.
Supplemental Material
Supplemental data for this paper can be accessed on the publisher's website.
References
- Heim C, Binder EB. Current research trends in early life stress and depression: review of human studies on sensitive periods, gene-environment interactions, and epigenetics. Exp Neurol 2012; 233:102-111; PMID:22101006; http://dx.doi.org/10.1016/j.expneurol.2011.10.032
- Lupien SJ, McEwen BS, Gunnar MR, Heim C. Effects of stress throughout the lifespan on the brain, behaviour and cognition. Nat Rev Neurosci 2009; 10:434-445; PMID:19401723; http://dx.doi.org/10.1038/nrn2639
- Murgatroyd C, Wu Y, Bockmühl Y, Spengler D. Genes learn from stress: how infantile trauma programs us for depression. Epigenetics 2010; 5:194-199; PMID:20339319; http://dx.doi.org/10.4161/epi.5.3.11375
- Hoffmann A, Spengler D. DNA memories of early social life. Neuroscience 2014; 264:64-75; PMID:22575695; http://dx.doi.org/10.1016/j.neuroscience.2012.04.003
- de Kloet ER, Joels M, Holsboer F. Stress and the brain: from adaptation to disease. Nat Rev Neurosci 2005; 6:463-475; PMID:15891777; http://dx.doi.org/10.1038/nrn1683
- McCormick JA, Lyons V, Jacobson MD, Noble J, Diorio J, Nyirenda M, Weaver S, Ester W, Yau JL, Meaney MJ, et al. 5′-heterogeneity of glucocorticoid receptor messenger RNA is tissue specific: differential regulation of variant transcripts by early-life events. Mol Endocrinol 2000; 14:506-517; PMID:10770488; http://dx.doi.org/10.1210/mend.14.4.0438
- Bockmühl Y, Murgatroyd CA, Kuczynska A, Adcock IM, Almeida OFX, Spengler D. Differential regulation and function of 5′-untranslated GR-exon 1 transcripts. Mol Endocrinol 2011; 25:1100-1110; PMID:21527501; http://dx.doi.org/10.1210/me.2010-0436
- Vandevyver S, Dejager L, Libert C. Comprehensive overview of the structure and regulation of the glucocorticoid receptor. Endocr Rev 2014; 35:671-693; PMID:24937701; http://dx.doi.org/10.1210/er.2014-1010
- Weaver IC, Cervoni N, Champagne FA, D'Alessio AC, Sharma S, Seckl JR, Dymov S, Szyf M, Meaney MJ. Epigenetic programming by maternal behavior. Nat Neurosci 2004; 7:847-854; PMID:15220929; http://dx.doi.org/10.1038/nn1276
- Romens SE, McDonald J, Svaren J, Pollak SD. Associations between early life stress and gene methylation in children. Child Dev 2014; 86:303-9; PMID:25056599; http://dx.doi.org/10.1111/cdev.12270
- Hompes T, Izzi B, Gellens E, Morreels M, Fieuws S, Pexsters A, Schops G, Dom M, van Bree R, Freson K, et al. Investigating the influence of maternal cortisol and emotional state during pregnancy on the DNA methylation status of the glucocorticoid receptor gene (NR3C1) promoter region in cord blood. J Psychiatr Res 2013; 47:880-891; PMID:23566423; http://dx.doi.org/10.1016/j.jpsychires.2013.03.009
- Mulligan CJ, D'Errico NC, Stees J, Hughes DA. Methylation changes at NR3C1 in newborns associate with maternal prenatal stress exposure and newborn birth weight. Epigenetics 2012; 7:853-857; PMID:22810058; http://dx.doi.org/10.4161/epi.21180
- Oberlander TF, Weinberg J, Papsdorf M, Grunau R, Misri S, Devlin AM. Prenatal exposure to maternal depression, neonatal methylation of human glucocorticoid receptor gene (NR3C1) and infant cortisol stress responses. Epigenetics 2008; 3:97-106; PMID:18536531; http://dx.doi.org/10.4161/epi.3.2.6034
- Radtke KM, Ruf M, Gunter HM, Dohrmann K, Schauer M, Meyer A, Elbert T. Transgenerational impact of intimate partner violence on methylation in the promoter of the glucocorticoid receptor. Transl Psychiatry 2011; 1:e21; PMID:22832523; http://dx.doi.org/10.1038/tp.2011.21
- Labonte B, Yerko V, Gross J, Mechawar N, Meaney MJ, Szyf M, Turecki G. Differential glucocorticoid receptor exon 1(B), 1(C), and 1(H) expression and methylation in suicide completers with a history of childhood abuse. Biol Psychiatry 2012; 72:41-48; PMID:22444201; http://dx.doi.org/10.1016/j.biopsych.2012.01.034
- McGowan PO, Sasaki A, D'Alessio AC, Dymov S, Labonte B, Szyf M, Turecki G, Meaney MJ. Epigenetic regulation of the glucocorticoid receptor in human brain associates with childhood abuse. Nat Neurosci 2009; 12:342-348; PMID:19234457; http://dx.doi.org/10.1038/nn.2270
- Martín-Blanco A, Ferrer M, Soler J, Salazar J, Vega D, Andión O, Sanchez-Mora C, Arranz MJ, Ribases M, Feliu-Soler A, et al. Association between methylation of the glucocorticoid receptor gene, childhood maltreatment, and clinical severity in borderline personality disorder. J Psychiatr Res 2014; 57:34-40; PMID:25048180; http://dx.doi.org/10.1016/j.jpsychires.2014.06.011
- Perroud N, Paoloni-Giacobino A, Prada P, Olié E, Salzmann A, Nicastro R, Guillaume S, Mouthon D, Stouder C, Dieben K, et al. Increased methylation of glucocorticoid receptor gene (NR3C1) in adults with a history of childhood maltreatment: a link with the severity and type of trauma. Transl Psychiatry 2011; 1:e59; PMID:22832351; http://dx.doi.org/10.1038/tp.2011.60
- Dammann G, Teschler S, Haag T, Altmüller F, Tuczek F, Dammann RH. Increased DNA methylation of neuropsychiatric genes occurs in borderline personality disorder. Epigenetics 2011; 6:1454-1462; PMID:22139575; http://dx.doi.org/10.4161/epi.6.12.18363
- Yehuda R, Daskalakis NP, Lehrner A, Desarnaud F, Bader HN, Makotkine I, Flory JD, Bierer LM, Meaney MJ. Influences of maternal and paternal PTSD on epigenetic regulation of the glucocorticoid receptor gene in holocaust survivor offspring. Am J Psychiatry 2014; 171:872-880; PMID:24832930; http://dx.doi.org/10.1176/appi.ajp.2014.13121571
- Vukojevic V, Kolassa I, Fastenrath M, Gschwind L, Spalek K, Milnik A, Heck A, Vogler C, Wilker S, Demougin P, et al. Epigenetic modification of the glucocorticoid receptor gene is linked to traumatic memory and post-traumatic stress disorder risk in genocide survivors. J Neurosci 2014; 34:10274-10284; PMID:25080589; http://dx.doi.org/10.1523/JNEUROSCI.1526-14.2014
- Labonté B, Azoulay N, Yerko V, Turecki G, Brunet A. Epigenetic modulation of glucocorticoid receptors in posttraumatic stress disorder. Transl Psychiatry 2014; 4:e368; PMID:24594779; http://dx.doi.org/10.1038/tp.2014.3
- McGowan PO, Suderman M, Sasaki A, Huang TC, Hallett M, Meaney MJ, Szyf M. Broad epigenetic signature of maternal care in the brain of adult rats. PLoS One 2011; 6:e14739; PMID:21386994; http://dx.doi.org/10.1371/journal.pone.0014739
- Suderman M, McGowan PO, Sasaki A, Huang TC, Hallett MT, Meaney MJ, Turecki G, Szyf M. Conserved epigenetic sensitivity to early life experience in the rat and human hippocampus. Proc Natl Acad Sci U S A 2012; 109(Suppl 2):17266-17272; PMID:23045659; http://dx.doi.org/10.1073/pnas.1121260109
- Murgatroyd C, Patchev AV, Wu Y, Micale V, Bockmuhl Y, Fischer D, Holsboer F, Wotjak CT, Almeida OF, Spengler D. Dynamic DNA methylation programs persistent adverse effects of early-life stress. Nat Neurosci 2009; 12:1559-1566; PMID:19898468; http://dx.doi.org/10.1038/nn.2436
- Wu Y, Patchev AV, Daniel G, Almeida OF, Spengler D. Early-life stress reduces DNA methylation of the Pomc gene in male mice. Endocrinology 2014; 155:1751-1762; PMID:24506071; http://dx.doi.org/10.1210/en.2013-1868
- Storer CL, Dickey CA, Galigniana MD, Rein T, Cox MB. FKBP51 and FKBP52 in signaling and disease. Trends Endocrinol Metab 2011; 22:481-490; PMID:21889356; http://dx.doi.org/10.1016/j.tem.2011.08.001
- Anacker C, Cattaneo A, Musaelyan K, Zunszain PA, Horowitz M, Molteni R, Luoni A, Calabrese F, Tansey K, Gennarelli M, et al. Role for the kinase SGK1 in stress, depression, and glucocorticoid effects on hippocampal neurogenesis. Proc Natl Acad Sci U S A 2013; 110:8708-8713; PMID:23650397; http://dx.doi.org/10.1073/pnas.1300886110
- Duric V, Banasr M, Licznerski P, Schmidt HD, Stockmeier CA, Simen AA, Newton SS, Duman RS. A negative regulator of MAP kinase causes depressive behavior. Nat Med 2010; 16:1328-1332; PMID:20953200; http://dx.doi.org/10.1038/nm.2219
- Lang F, Strutz-Seebohm N, Seebohm G, Lang UE. Significance of SGK1 in the regulation of neuronal function. J Physiol (Lond) 2010; 588:3349-3354; PMID:20530112; http://dx.doi.org/10.1113/jphysiol.2010.190926
- Arteaga MF, Coric T, Straub C, Canessa CM. A brain-specific SGK1 splice isoform regulates expression of ASIC1 in neurons. Proc Natl Acad Sci U S A 2008; 105:4459-4464; PMID:18334630; http://dx.doi.org/10.1073/pnas.0800958105
- Hubler TR, Scammell JG. Intronic hormone response elements mediate regulation of FKBP5 by progestins and glucocorticoids. Cell Stress Chaperones 2004; 9:243-252; PMID:15544162; http://dx.doi.org/10.1379/CSC-32R.1
- Tchen CR, Martins JRS, Paktiawal N, Perelli R, Saklatvala J, Clark AR. Glucocorticoid regulation of mouse and human dual specificity phosphatase 1 (DUSP1) genes: unusual cis-acting elements and unexpected evolutionary divergence. J Biol Chem 2010; 285:2642-2652; PMID:19940143; http://dx.doi.org/10.1074/jbc.M109.037309
- Irizarry RA, Ladd-Acosta C, Wen B, Wu Z, Montano C, Onyango P, Cui H, Gabo K, Rongione M, Webster M, et al. The human colon cancer methylome shows similar hypo- and hypermethylation at conserved tissue-specific CpG island shores. Nat Genet 2009; 41:178-186; PMID:19151715; http://dx.doi.org/10.1038/ng.298
- Ziller MJ, Gu H, Müller F, Donaghey J, Tsai LT, Kohlbacher O, de Jager PL, Rosen ED, Bennett DA, Bernstein BE, et al. Charting a dynamic DNA methylation landscape of the human genome. Nature 2013; 500:477-481; PMID:23925113; http://dx.doi.org/10.1038/nature12433
- Breslin MB, Vedeckis WV. The human glucocorticoid receptor promoter upstream sequences contain binding sites for the ubiquitous transcription factor, Yin Yang 1. J Steroid Biochem Mol Biol 1998; 67:369-381; PMID:10030686; http://dx.doi.org/10.1016/S0960-0760(98)00138-1
- He Y, Casaccia-Bonnefil P. The Yin and Yang of YY1 in the nervous system. J Neurochem 2008; 106:1493-1502; PMID:18485096; http://dx.doi.org/10.1111/j.1471-4159.2008.05486.x
- Joo M, Wright JG, Hu NN, Sadikot RT, Park GY, Blackwell TS, Christman JW. Yin Yang 1 enhances cyclooxygenase-2 gene expression in macrophages. Am J Physiol Lung Cell Mol Physiol 2007; 292:L1219-26; PMID:17220375; http://dx.doi.org/10.1152/ajplung.00474.2006
- Caretti G, Di Padova M, Micales B, Lyons GE, Sartorelli V. The Polycomb Ezh2 methyltransferase regulates muscle gene expression and skeletal muscle differentiation. Genes Dev 2004; 18:2627-2638; PMID:15520282; http://dx.doi.org/10.1101/gad.1241904
- Son H, Kim J, Hahn Y, Seo S. Negative regulation of JAK2 by H3K9 methyltransferase G9a in leukemia. Mol Cell Biol 2012; 32:3681-3694; PMID:22801367; http://dx.doi.org/10.1128/MCB.00673-12
- Yang WM, Yao YL, Sun JM, Davie JR, Seto E. Isolation and characterization of cDNAs corresponding to an additional member of the human histone deacetylase gene family. J Biol Chem 1997; 272:28001-28007; PMID:9346952; http://dx.doi.org/10.1074/jbc.272.44.28001
- Ko CY, Hsu HC, Shen CW, Wang JM. Epigenetic silencing of CCAAT/enhancer-binding protein delta activity by YY1/polycomb group/DNA methyltransferase complex. J Biol Chem 2008; 283:30919-30932; PMID:18753137; http://dx.doi.org/10.1074/jbc.M804029200
- Chaturvedi C, Somasundaram B, Singh K, Carpenedo RL, Stanford WL, Dilworth FJ, Brand M. Maintenance of gene silencing by the coordinate action of the H3K9 methyltransferase G9a/KMT1C and the H3K4 demethylase Jarid1a/KDM5A. Proc Natl Acad Sci U S A 2012; 109:18845-18850; PMID:23112189; http://dx.doi.org/10.1073/pnas.1213951109
- Bell AC, West AG, Felsenfeld G. The protein CTCF is required for the enhancer blocking activity of vertebrate insulators. Cell 1999; 98:387-396; PMID:10458613; http://dx.doi.org/10.1016/S0092-8674(00)81967-4
- Evans AN, Liu Y, Macgregor R, Huang V, Aguilera G. Regulation of hypothalamic corticotropin-releasing hormone transcription by elevated glucocorticoids. Mol Endocrinol 2013; 27:1796-1807; PMID:24065704; http://dx.doi.org/10.1210/me.2013-1095
- Biag J, Huang Y, Gou L, Hintiryan H, Askarinam A, Hahn JD, Toga AW, Dong H. Cyto- and chemoarchitecture of the hypothalamic paraventricular nucleus in the C57BL/6J male mouse: a study of immunostaining and multiple fluorescent tract tracing. J Comp Neurol 2012; 520:6-33; PMID:21674499; http://dx.doi.org/10.1002/cne.22698
- Kadar A, Sanchez E, Wittmann G, Singru PS, Fuzesi T, Marsili A, Larsen PR, Liposits Z, Lechan RM, Fekete C. Distribution of hypophysiotropic thyrotropin-releasing hormone (TRH)-synthesizing neurons in the hypothalamic paraventricular nucleus of the mouse. J Comp Neurol 2010; 518:3948-3961; PMID:20737594; http://dx.doi.org/10.1002/cne.22432
- Wamsteeker Cusulin JI, Fuzesi T, Watts AG, Bains JS. Characterization of corticotropin-releasing hormone neurons in the paraventricular nucleus of the hypothalamus of Crh-IRES-Cre mutant mice. PLoS One 2013; 8:e64943; PMID:23724107; http://dx.doi.org/10.1371/journal.pone.0064943
- Herbeck YE, Gulevich RG, Amelkina OA, Plyusnina IZ, Oskina IN. Conserved methylation of the glucocorticoid receptor gene exon 1(7) promoter in rats subjected to a maternal methyl-supplemented diet. Int J Dev Neurosci 2010; 28:9-12; PMID:19874883; http://dx.doi.org/10.1016/j.ijdevneu.2009.10.004
- Daniels WM, Fairbairn LR, van Tilburg G, McEvoy CR, Zigmond MJ, Russell VA, Stein DJ. Maternal separation alters nerve growth factor and corticosterone levels but not the DNA methylation status of the exon 1(7) glucocorticoid receptor promoter region. Metab Brain Dis 2009; 24:615-627; PMID:19816761; http://dx.doi.org/10.1007/s11011-009-9163-4
- Moser D, Molitor A, Kumsta R, Tatschner T, Riederer P, Meyer J. The glucocorticoid receptor gene exon 1-F promoter is not methylated at the NGFI-A binding site in human hippocampus. World J Biol Psychiatry 2007; 8:262-268; PMID:17853270; http://dx.doi.org/10.1080/15622970701429862
- Mueller BR, Bale TL. Sex-specific programming of offspring emotionality after stress early in pregnancy. J Neurosci 2008; 28:9055-9065; PMID:18768700; http://dx.doi.org/10.1523/JNEUROSCI.1424-08.2008
- Alt SR, Turner JD, Klok MD, Meijer OC, Lakke EA, Derijk RH, Muller CP. Differential expression of glucocorticoid receptor transcripts in major depressive disorder is not epigenetically programmed. Psychoneuroendocrinology 2010; 35:544-556; PMID:19782477; http://dx.doi.org/10.1016/j.psyneuen.2009.09.001
- Klengel T, Mehta D, Anacker C, Rex-Haffner M, Pruessner JC, Pariante CM, Pace TWW, Mercer KB, Mayberg HS, Bradley B. et al. Allele-specific FKBP5 DNA demethylation mediates gene-childhood trauma interactions. Nat Neurosci 2013; 16:33-41; PMID:23201972; http://dx.doi.org/10.1038/nn.3275
- Patchev AV, Rodrigues AJ, Sousa N, Spengler D, Almeida OFX. The future is now: early life events preset adult behaviour. Acta Physiol (Oxf) 2014; 210:46-57; PMID:23790203; http://dx.doi.org/10.1111/apha.12140
- Goncharova ND. Stress responsiveness of the hypothalamic-pituitary-adrenal axis: age-related features of the vasopressinergic regulation. Front Endocrinol (Lausanne) 2013; 4:26; PMID:23486926; http://dx.doi.org/10.3389/fendo.2013.00026
- Rutter M. Resilience as a dynamic concept. Dev Psychopathol 2012; 24:335-344; PMID:22559117; http://dx.doi.org/10.1017/S0954579412000028
- Russo SJ, Murrough JW, Han M, Charney DS, Nestler EJ. Neurobiology of resilience. Nat Neurosci 2012; 15:1475-1484; PMID:23064380; http://dx.doi.org/10.1038/nn.3234
- Zannas AS, West AE. Epigenetics and the regulation of stress vulnerability and resilience. Neuroscience 2014; 264:157-170; PMID:24333971; http://dx.doi.org/10.1016/j.neuroscience.2013.12.003
- Surjit M, Ganti KP, Mukherji A, Ye T, Hua G, Metzger D, Li M, Chambon P. Widespread negative response elements mediate direct repression by agonist-liganded glucocorticoid receptor. Cell 2011; 145:224-241; PMID:21496643; http://dx.doi.org/10.1016/j.cell.2011.03.027
- Sharma D, Bhave S, Gregg E, Uht R. Dexamethasone induces a putative repressor complex and chromatin modifications in the CRH promoter. Mol Endocrinol 2013; 27:1142-1152; PMID:23671328; http://dx.doi.org/10.1210/me.2013-1079
- Ramamoorthy S, Cidlowski JA. Ligand-induced repression of the glucocorticoid receptor gene is mediated by an NCoR1 repression complex formed by long-range chromatin interactions with intragenic glucocorticoid response elements. Mol Cell Biol 2013; 33:1711-1722; PMID:23428870; http://dx.doi.org/10.1128/MCB.01151-12
- Bessa JM, Ferreira D, Melo I, Marques F, Cerqueira JJ, Palha JA, Almeida, O F X, Sousa N. The mood-improving actions of antidepressants do not depend on neurogenesis but are associated with neuronal remodeling. Mol Psychiatry 2009; 14:764-73, 739; PMID:18982002; http://dx.doi.org/10.1038/mp.2008.119
- Willner P. Chronic mild stress (CMS) revisited: consistency and behavioural-neurobiological concordance in the effects of CMS. Neuropsychobiology 2005; 52:90-110; PMID:16037678; http://dx.doi.org/10.1159/000087097
- Fleige S, Walf V, Huch S, Prgomet C, Sehm J, Pfaffl MW. Comparison of relative mRNA quantification models and the impact of RNA integrity in quantitative real-time RT-PCR. Biotechnol Lett 2006; 28:1601-1613; PMID:16900335; http://dx.doi.org/10.1007/s10529-006-9127-2
- Kim JD, Hinz AK, Choo JH, Stubbs L, Kim J. YY1 as a controlling factor for the Peg3 and Gnas imprinted domains. Genomics 2007; 89:262-269; PMID:17067777; http://dx.doi.org/10.1016/j.ygeno.2006.09.009
- Kim J, Kollhoff A, Bergmann A, Stubbs L. Methylation-sensitive binding of transcription factor YY1 to an insulator sequence within the paternally expressed imprinted gene, Peg3. Hum Mol Genet 2003; 12:233-245; PMID:12554678; http://dx.doi.org/10.1093/hmg/ddg028
- Yang WM, Inouye C, Zeng Y, Bearss D, Seto E. Transcriptional repression by YY1 is mediated by interaction with a mammalian homolog of the yeast global regulator RPD3. Proc Natl Acad Sci U S A 1996; 93:12845-12850; PMID:8917507; http://dx.doi.org/10.1073/pnas.93.23.12845