Abstract
Beckwith–Wiedemann syndrome (BWS) is an imprinting disorder that can be prenatally suspected or diagnosed based on established clinical guidelines. Molecular confirmation is commonly performed on amniocytes. The possibility to use fresh (CVF) and cultured (CVC) chorionic villi has never been investigated. To verify whether CVF and CVC are reliable sources of DNA to study fetal methylation, we used pyrosequencing to test the methylation level of a number of differentially methylated regions (DMRs) at several imprinted loci (ICR1, ICR2, H19, PWS/AS-ICR, GNASXL, GNAS1A, ZAC/PLAGL1, and MEST) and at non-imprinted MGMT and RASSF1A promoters. We analyzed these regions in 19 healthy pregnancies and highlighted stable methylation levels between CVF and CVC at ICR1, ICR2, GNASXL, PWS/AS-ICR, and MEST. Conversely, the methylation levels at H19 promoter, GNAS1A and ZAC/PLAGL1 were different in CVC compared to fresh CV. We also investigated ICR1 and ICR2 methylation level of CVF/CVC of 2 BWS-suspected fetuses (P1 and P2). P1 showed ICR2 hypomethylation, P2 showed normal methylation at both ICR1 and ICR2. Our findings, although limited to one case of BWS fetus with an imprinting defect, can suggest that ICR1 and ICR2, but not H19, could be reliable targets for prenatal BWS diagnosis by methylation test in CVF and CVC. In addition, PWS/AS-ICR, GNASXL, and MEST, but not GNAS1A and ZAC/PLAGL1, are steadily hemimethylated in CV from healthy pregnancies, independently from culture. Thus, prenatal investigation of genomic imprinting in CV needs to be validated in a locus-specific manner.
Abbreviations
ART | = | Assisted Reproductive Technologies |
AS | = | Angelman Syndrome |
BWS | = | Beckwith-Wiedemann Syndrome |
CV | = | Chorionic Villi |
CVC | = | Cultured Chorionic Villi |
CVF | = | Fresh Chorionic Villi |
DMR | = | Differentially Methylated Regions |
ICR | = | Imprinting Control Region |
IUGR | = | Intra-Uterine Growth Restriction |
MMD | = | Multilocus Methylation Defect |
PWS | = | Prader-Willi Syndrome |
SGA | = | Small for Gestational Age |
SRS | = | Silver Russell Syndrome |
UPD | = | Uniparental Disomy |
US | = | Ultra Sound |
Introduction
Genomic imprinting is a mammalian epigenetic phenomenon that results in monoallelic gene expression determined by the parental origin of the allele.Citation1 Almost all imprinted genes are organized in clusters and have differences in their CpG site-methylation profile at key regulatory elements.Citation2
The role of DNA methylation in gene expression is well characterized at the imprinted locus 11p15. This cluster comprises 2 distinct domains regulated by the imprinting control regions 1 (ICR1) and 2 (ICR2).Citation3 Genetic and epigenetic defects at this cluster are associated with Beckwith–Wiedemann syndrome (BWS, OMIM: #130650) and Silver–Russell syndrome (SRS, OMIM: #180860), which are growth disorders with opposite phenotypes. In healthy individuals, the paternally derived ICR1 allele is methylated and the maternal one is unmethylated; at ICR2, the opposite methylation pattern is observed. BWS may arise from one of the following mechanisms: hypomethylation at ICR2 (50–60% of cases); hypermethylation at ICR1 (2–8% of cases); mosaic segmental paternal uniparental disomy (UPD) (10–20% of cases); and mutations of the maternal CDKN1C allele (5–10% of cases).Citation4 Conversely, SRS is associated with hypomethylation of ICR1 (35–50% of cases), duplication of the maternal 11p15.5 (unknown frequency), maternal UPD of chromosome 7 (about 10% of the cases), and deletions/duplications of the same chromosome (rare).Citation5
In the last few years, several groups Citation6-8 have demonstrated that DNA methylation defects in imprinting disorders can involve multilocus methylation defects (MMDs) of imprinted loci. For example, Azzi et al. first demonstrated that 9.5% of SRS and 24% of BWS have MMDs at additional DMRs other than 11p15.5. Citation8 No significant differences in clinical features exist between BWS patients with multilocus or single-locus methylation defect.Citation8
BWS can be prenatally suspected, or provisionally diagnosed, applying the guidelines proposed by Williams et al.Citation9 They set up a diagnostic scheme based on the identification of at least 2 major findings in ultrasound (US) examination (i.e., an abdominal wall defect, macroglossia, or macrosomia), or one major and 2 minor findings (i.e., nephromegaly/dysgenesis, adrenal cytomegaly, aneuploidy/abnormal loci, or polyhydramnios).Citation9 Molecular confirmation of BWS is essential for an accurate prenatal counseling that considers the possibility of recurrence and the postnatal risk of additional BWS manifestations such as childhood cancers. BWS patients have increased risk of developing certain embryonic tumors: Wilms’ tumor, adrenocortical carcinoma, hepatoblastoma, gonadoblastoma, rhabdomyosarcoma, and neuroblastoma.Citation9 In particular, 28.6% and 17.3% of the patients with hypermethylation at ICR1 and 11p15 UPD, respectively, develop Wilms’ tumors during infancy. By contrast, the prevalence of childhood cancers in patients with hypomethylated ICR2 or CDKN1C mutations is 3.1% and 8.8%, respectively.Citation10,11 The risk of BWS recurrence in the same family depends on the genetic/epigenetic defect in the proband: the risk is low in absence of genomic rearrangements (deletions/duplications) and in the presence of single or multilocus methylation defects and UPD. On the contrary, the recurrence risk may be as high as 50% in those with genomic defects or CDKN1C mutations. These data support the need for an early and accurate molecular diagnosis of BWS to the tailored surveillance of patients.
In contrast to BWS, SRS diagnosis is mainly performed after birth in infants with growth restriction, hypoglycemia, body-asymmetry, triangular facial appearance, and clinodactyly.Citation12 During prenatal life, the main US manifestation is intrauterine growth restriction, which is an unspecific condition. Therefore, prenatal molecular investigation is rarely prompted only by fetal morphology.
Beside the presence of morphological fetal defects, molecular prenatal testing for imprinting diseases can be prompted by the finding of fetal/placental karyotypes that may predispose to UPD formation (i.e., Robertsonian and reciprocal translocations, structural rearrangements, and confined placental mosaicism involving chromosomes with imprinted genes).
Moreover, imprinting disorders are possibly associated with assisted reproductive technologies (ART);Citation13,14 concerning BWS, it seems to be more frequent in ART than in spontaneous conceptions.Citation15
For prenatal methylation analyses, both chorionic villi (CV) and amniocytes are eligible DNA sources.Citation11,16 CV sampling offers the advantage of an earlier diagnosis than amniocentesis with comparable risks,Citation17 even if it should be considered that the methylation of the placenta is generally lower than that of the embryo.Citation16,18 Furthermore, when an imprinting disease is suspected after CV karyotyping, the left over cultured villi could represent a unique and easily available source of fetal DNA for methylation analysis during the first trimester of pregnancy. For this reason, it is important to ascertain whether villi culturing influences the methylation pattern.
The aim of our study was to assess the feasibility of prenatal diagnosis (PD) of methylation defects, in particular BWS, in fresh (CVF) and cultured CV (CVC). We analyzed normal and pathological pregnancies to investigate CV methylation patterns at both imprinted and non-imprinted regions, as well as the effect of culturing on methylation profile. In particular, we evaluated ICR1, ICR2, the H19 promoter (11p15.5), PWS/AS-ICR (15q11–q13), GNASXL and GNAS1A (20q13.32), ZAC/PLAG1 (6q24) and MEST (7q32) imprinted loci, and the promoters of the non-imprinted MGMT and RASSF1 genes.
Results
CV do not modify the methylation pattern at ICR1 and ICR2 during culturing
Since BWS is mainly linked to imprinting defects at chromosome cluster 11p15.5, we first investigated the methylation status at ICR1, ICR2, and H19 promoter in 19 normal pregnancies. Mean ICR1 and ICR2 methylation percentages were 45.38 ± 1.77% and 44.32 ± 1.84% in CVF, respectively, and 45.04 ± 1.81% and 43.67 ± 2.10% in CVC, respectively (). These results demonstrate that CVF and CVC methylation levels at these imprinted control regions are very similar (P = 0.457 and P = 0.176 for ICR1 and ICR2, respectively), indicating that the culturing of CV does not modify ICR1 and ICR2 physiological methylation pattern.
Figure 1. Quantitative CpG methylation analysis of ICR1, ICR2 and H19 in CVF and CVC from normal pregnancies. Symbols represent mean methylation percentage of at least two independent experiments for each sample. Black full squares symbolize fresh chorionic villi (CVF); empty triangles cultured chorionic villi (CVC). Mean percentage value is calculated on a different number of CpG sites analyzed at each locus. White boxes report mean methylation level ± SD calculated for all the analyzed CVF and CVC. Loci that maintain stable methylation values (ICR1 and ICR2) in CVF and CVC are grouped together in a full-line square.
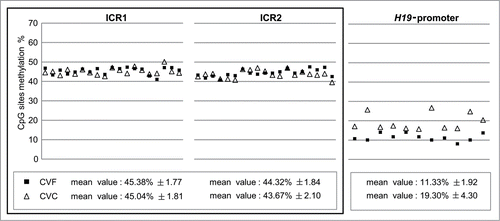
In contrast, we found that the H19 promoter was hypomethylated in both CVF (11.33 ± 1.92%) and CVC (19.30 ± 4.30%), compared to the expected profile for an imprinted locus. This result is consistent with H19 promoter methylation pattern in third trimester healthy pregnancies, previously identified by our groupCitation16. In addition, the H19 mean methylation levels in CVC were higher than those in CVF (P = 0.0005) (), indicating an effect of CV culture on H19 promoter methylation.
Methylation analysis in fresh and cultured CV requires gene-specific validation
Given the results obtained at 11p15.5 imprinted DMRs, to ascertain the feasibility of methylation analyses at other imprinted loci, we extended our study to 5 additional imprinted loci: GNASXL and GNAS1A (20q13.32), PWS/AS-ICR (15q11–q13), ZAC/PLAG1 (6q24) and MEST (7q32) (). As control genes, we studied the methylation profile of non-imprinted MGMT and RASSF1 gene promoters.
Figure 2. Quantitative CpG methylation analysis of imprinted and non-imprinted loci in CVF and CVC from normal pregnancies. The following loci were analyzed: (A) GNASXL, PWS/AS-ICR, MEST; (B) GNAS1A, ZAC/PLAG1; (C) MGMT and RASSF1. Symbols represent mean methylation percentage of at least two independent experiments for each sample. Black full squares symbolize fresh chorionic villi (CVF); empty triangles cultured chorionic villi (CVC). Mean percentage value is calculated on a different number of CpG sites analyzed at each locus. White boxes report mean methylation level ± SD calculated for all the analyzed CVF and CVC. Loci that maintain stable methylation values (GNASXL, PWS/AS-ICR, MEST) in CVF and CVC are grouped together in a full-line square.
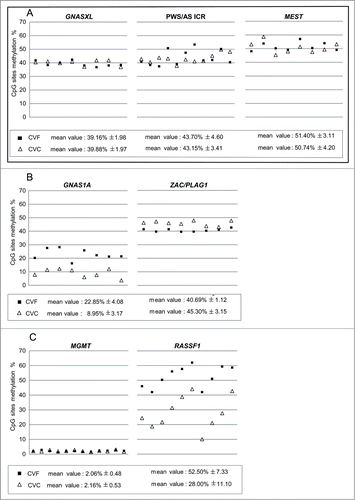
We found that GNASXL, PWS/AS-ICR, and MEST were stably hemimethylated in both CVF (GNASXL: 39.16% ± 1.98, PWS/AS-ICR: 43.70% ± 4.60, MEST: 51.40% ± 3.11) and CVC (GNASXL: 39.88% ± 1.97, PWS/AS-ICR: 43.15% ± 3.41, MEST: 50.74% ± 4.20) (). These results highlight that, at these DMRs, CV methylation pattern is not influenced by cell culturing (GNASXL: P = 0.48; PWS/AS-ICR: P = 0.766, MEST: P = 0.73). In addition, the methylation values at all these DMRs were as expected for imprinted loci and similar to those found in peripheral lymphocytes from healthy donors (data not shown).
On the contrary, GNAS1A imprinted gene was severely hypomethylated in CVF and CVC (CVF: 22.85% ± 4.08; CVC: 8.95% ± 3.17) and exhibited methylation instability after culture (P = 2.44486E-06) (). Similarly, ZAC/PLAG1 methylation was not stable after culture (P = 0.0016) but showed CVF and CVC methylation values in accordance with an imprinted locus (CVF: 40.69% ± 1.12, CVC: 45.30% ± 3.15) (). GNAS1A and ZAC/PLAG1 were hemimethylated in peripheral lymphocytes from healthy donors (data not shown).
Finally, concerning the non-imprinted investigated loci, MGMT promoter was unmethylated in both CVF and CVC (CVF: 2.06 ± 0.48%, CVC: 2.16 ± 0.53%), without statistically significant differences before and after CV culture (P = 0.605) (). Methylation values of the exon 1 CpG sites of the RASSF1 gene were 52.50 ± 7.33% in CVF and 28.00 ± 11.10% in CVC (), indicating that the methylation levels at this locus were consistently different after culture (P = 3.37 × 10−7).
Prenatal diagnosis of suspected BWS can be performed in fresh as well as in cultured CV
Given that the CV methylation levels of ICR1 and ICR2 were consistent with those expected for imprinted loci and stably maintained throughout culture in healthy pregnancies, we analyzed these regions in CVF and CVC from 2 fetuses (P1 and P2) with US anomalies suggestive for BWS.
At 11p15.5 imprinted region P1 had normal methylation at ICR1 and hypomethylation at ICR2 in both CVF (ICR1: 48.92 ± 2.13%; ICR2: 17.63 ± 0.88%) and CVC (ICR1: 45.25 ± 2.63%; ICR2: 16.13 ± 0.18%) (). Autopsy confirmed the presence of omphalocele. Hematoxylin/eosin staining of the placenta highlighted stem villi hyperplasia, focal vesicle-like villi and abnormal vascularization, thus supporting the US evidence for mesenchymal dysplasia (). ICR2 hypomethylation was also present in all the fetal specimens obtained from P1 autopsy, including skin (15.5 ± 1.00%), inner intestine (11.0 ± 1.41%), kidney (15.75 ± 0.96%), and intestine protruding from omphalocele (8.75 ± 1.71%); of note, the latter sample had the lowest methylation value ().
Figure 3. BWS-suspected pregnancies (P1 and P2) molecular results. (A) Histograms of the quantitative CpG methylation analysis performed at ICR1, ICR2, GNASXL, PWS/AS-ICR and MEST imprinted loci on P1 fetus and at ICR1 and ICR2 on P2 BWS-suspected case. Black bars represent CVS samples; gray bars CVC samples. (B) Hematoxylin/eosin staining of P1 placenta showing hypercellularity (fibroblasts overgrowth), enlargement of stem villi, hypervascularized villi (chorangiosis) with focal dilatation, branching, and sinusoid formation (H&E, 10x). (C) Table reporting P1 mean methylation percentage ± SD at ICR1, ICR2, GNASXL, PWS/AS-ICR and MEST in CVF, CVC, skin, inner intestine, kidney and intestine protruding from omphalocele.
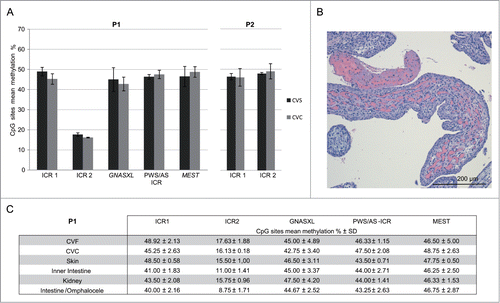
Since in P1 the molecular results confirmed the clinical diagnosis of BWS, to explore the possibility of MMDs, we carried out methylation analysis in CVF and CVC of imprinted loci (GNASXL, PWS/AS-ICR and MEST) that showed stable methylation in CV. We observed normal methylation values at all the investigated loci in both CVF (GNASXL: 45.00% ± 4.89; PWS/AS-ICR: 46.33% ± 1.15; MEST: 46.50% ± 5.00) and in CVC (GNASXL: 42.75% ± 3.40; PWS/AS-ICR: 47.50% ± 2.08 and MEST: 48.75% ± 2.63) (GNASXL: P = 0.53; PWS/AS-ICR: P = 0.43; MEST: P = 0.45). These methylation values were also confirmed in fetal autoptic tissues (), allowing us to exclude MMDs.
P2 displayed normal methylation levels at ICR1 and ICR2 in both CVF (ICR1: 46.38 ± 1.59%; ICR2: 47.88 ± 0.53%) and CVC (ICR1: 46.00 ± 4.32%; ICR2: 49.00 ± 3.77%) (). Since P2 was negative for 11p15.5 methylation defects, as second step of the diagnostic flowchart, CDKN1C sequencing was performed. We found a heterozygous missense mutation, c.491C>T (p.Pro164Leu), located within exon 1 encoding for the PAPA domain of the CDKN1C protein. This variant, not previously described, was inherited from the mother and confirmed in the fetus (data not shown). PolyPhen 2 (www.genetics.bwh.harvard.edu/pph2/) functional prediction suggested that it was benign.
Discussion
Prenatal molecular diagnosis of imprinting disorders is crucial in presence of fetal anomalies, particular fetal chromosomal abnormalities or a positive family history. The prenatal test for BWS, starting from the methylation analysis at 11p15.5, is usually performed on amniocytes. However, molecular tests on CV could be performed earlier in the pregnancy, and CV could be cultured to provide DNA for additional molecular investigations.
In normal pregnancies, we explored by pyrosequencing the feasibility of methylation analysis of CVF and CVC at different imprinted (ICR1, ICR2, H19 promoter, GNASXL, GNAS1A, ZAC/PLAGL1 and MEST) and non-imprinted (MGMT and RASSF1) loci. We also investigated 2 fetuses in which BWS was suspected because of the presence of omphalocele.
We found a high degree of stability in the methylation levels between CVF and CVC at ICR1, ICR2, GNASXL, PWS/AS-ICR and MEST. Furthermore, these regions were imprinted in CV, indicating that they did not undergo the genome-wide wave of demethylation characteristic of the placenta. We therefore surmise that these DMRs can be reliable targets for PD using CV.
Conversely, the imprinted locus H19, despite its chromosome position at 11p15.5 cluster, was hypomethylated and instable after CV culturing, in agreement with our previous observations.Citation16 The same trend was observed for GNAS1A imprinted gene that was hypomethylated in both CVF and CVC, with CVC showing a significant reduction of methylation. ZAC/PLAG1 imprinted locus was hemimethylated in CVF, as expected, but this pattern was not maintained after culture, where it was observed a slight, but significant, increased methylation. Thus, our data suggest that the methylation analysis of H19, GNAS1A and ZAC/PLAG1 promoters is not reliable for PD.
We also evaluated the effect of culturing on the methylation of the non-imprinted genes MGMT and RASSF1. MGMT is a housekeeping gene,Citation19 therefore its promoter is unmethylated. This pattern was present in CVF and maintained also after culture. Conversely, RASSF1 methylation is not maintained in CVC. Chiu et al.Citation20 analyzed the RASSF1 methylation profile in CVF by a semi-quantitative approach and found it to be hypermethylated compared to fetal tissues. Here, we confirmed this observation in CVF. After cell culture, the methylation consistently decreased by about 20% in each sample. This could be ascribed to culture conditions or to cell composition. Indeed, CVF samples are mainly composed of 2 different cell types: cytotrophoblasts and mesenchymal (stromal) cells. During culturing, only the mesenchymal cells expand; thus the methylation level can change if the mesenchymal cells have a different profile.
Taken together, our experiments in normal pregnancies highlight that some imprinted loci can be hypomethylated in fresh CV and that the methylation levels can vary after culturing in a locus-specific manner. Among the investigated regions, methylation at the DMRs ICR1, ICR2, GNASXL, PWS/AS-ICR and MEST seems not to be influenced by cell culture.
Our conclusions are strengthened by the methylation analyses of the BWS case P1, in which we found ICR2 hypomethylation in CVF as well as in CVC and in the tissues analyzed after abortion. In this case we also tested GNASXL, PWS/AS-ICR and MEST DMRs and excluded the MMD condition. Although in this case it was possible to perform PD for BWS using fresh and cultured CV, our observations essentially derived from one affected fetus only. In addition, since several imprinting disorders are mosaic, it is feasible that the placental and fetal tissues may have different methylation profile. Therefore, additional cases are needed to better define the possibility to detect methylation defects in placenta of suspected BWS fetuses.
P1, like the majority of BWS individuals conceived after ART, had methylation defects.Citation21 The severity of the hypomethylation at the affected locus and the persistence of the methylation anomaly in multiple anatomic sites suggest that the defect was on the maternally inherited 11p15 allele and already present in the oocyte. This methylation defect could be a consequence of the ovarian stimulation during ART procedures. Finally, P1 also presented with placental mesenchymal dysplasia, which is reported in 19% of BWS cases.Citation22 This clinical feature is related, in more than half of cases, to other pregnancy complications, including intrauterine growth restriction and/or fetal death, preterm delivery, and spontaneous preterm labor.Citation22
P2 did not have primary ICR1/ICR2 methylation defects in either CVF or CVC. CDKN1C sequencing revealed a maternally inherited missense variant within exon 1 that has not been previously described and might be associated with BWS.
In summary, we showed that prenatal investigation of BWS on fresh and cultured cells of first trimester CV can be reliable because the methylation profile at ICR1 and ICR2 is maintained in the placenta and unaffected by culture. Despite this, the methylation analysis of imprinted genes in fresh and in cultured CV requires caution because the imprinting signatures in the placenta can be different from the fetal compartment and can vary after culturing in a locus-specific manner.
Patients and Methods
Population
Samples included in the study were 19 first-trimester CV from singleton pregnancies without prenatal signs of imprinting disorders, recruited at the Obstetric Unit of Policlinico Mangiagalli Hospital (Milan, Italy) from 2013 to 2014, after an informed consent was obtained. In 18 cases the indication for PD was the advanced maternal age; in one case it was a positive Bi-Test, a first trimester non-invasive screening to identify pregnancies at risk for fetal aneuploidies.Citation23 Mean gestational age at CV sampling was 11 weeks gestation (wg). Eighteen conceptions were spontaneous; one was from in vitro fertilization (IVF). The karyotype analyses did not indicate chromosomal anomalies. We analyzed both CVF and CVC from the residual biological material used for the cytogenetics. CVC were obtained after about 21 days of culture, using standard procedures and conditions.Citation24
In addition, we investigated CVF and CVC from two pregnancies (P1 and P2) in which the suspicion of BWS arose from US investigation. P1 (12th wg) presented with both mesenchymal dysplasia and omphalocele. The pregnancy was voluntarily aborted at 14th wg, and skin, kidney, inner intestine, and intestine protruding from the omphalocele were also analyzed for their methylation pattern. Conception was obtained by IVF.
P2 (12th wg), from the Pediatric Unit of Papa Giovanni XXIII Hospital (Bergamo, Italy), had a giant isolated omphalocele, with liver and stomach herniation. Pregnancy was voluntarily terminated at 18th wg. Conception was spontaneous.
DNA methylation analysis
Genomic DNA from CVF, CVC, and fetal fragments was isolated using QIAamp DNA Mini Kit (Qiagen) and quantified by the NanoDrop ND1000 spectrophotometer (NanoDrop Technologies). Sodium bisulfite conversion of DNA (500 ng) was performed using the EZ DNA Methylation-Gold Kit (Zymo Research Corporation). PCRs were carried out using 20 ng of bisulfite-converted DNA and 10 pmol of forward and reverse primers, one of them biotinylated. Quantitative DNA methylation analyses were performed using a Pyro Mark ID instrument (Biotage) in the PSQ HS 96 System (Biotage), with the PyroGold SQA reagent kit (Biotage). Raw data were analyzed using the Q-CpG software v1.0.9 (Biotage), which calculates the ratio of converted to unconverted cytosines at each CpG, giving the percentage of methylation. Each sample was run in duplicate, and the methylation value was considered reliable when the SD between the two independent experiments was below 5%. The given methylation value is the result of the mean methylation levels of different CpG sites at each locus. Results are shown as the mean of the analyzed CpG sites ± SD. The methylation levels were compared using the Student's t-test.
We investigated the methylation of the following imprinted and non-imprinted loci (Supplemental file 1): ICR1, ICR2, and the H19 promoter, located at the imprinted region 11p15.5Citation11,16, PWS/AS-ICR, located at the imprinted region 15q11-q13Citation25; the promoter regions of MGMT at 10q26; RASSF1A gene, located at the non-imprinted locus 3p21.3; the DMRs GNASXL and GNAS1A at 20q13.32, MEST at 7q32.2 and ZAC/PLAG1 at 6q24.2. Genomic positions, primer sequences, PCR and pyrosequencing conditions are detailed in Supplemental file 1.
Disclosure of Potential Conflicts of Interest
No potential conflicts of interest were disclosed.
Authors’ Contributions
MM, ST, and SS: conception and design of the study and writing of the manuscript. LP, NC, LF: laboratory experiments, data interpretation, and writing of the manuscript. RS, SM, SF, SG, FL and AC: materials providing and data interpretation. All authors read and approved the manuscript.
Table_S1.zip
Download Zip (18.9 KB)Acknowledgements
We are thankful to the patients and controls for the participation in this study.
Funding
This work was supported by grants from the Fondazione IRCCS Ca’ Granda, Ospedale Maggiore Policlinico: “Ricerca Corrente” [grant number RC2014/519-02], “Progetto a concorso” (to MM) [grant number PC2014-15/519-03], and by the Ministry of Health: “Ricerca Finalizzata 2011 (to MM) [grant number RF-2011-02347106].
Supplemental Material
Supplemental data for this article can be accessed on the publisher's website.
References
- Peters J. The role of genomic imprinting in biology and disease: an expanding view. Nat Rev Genet 2014; 15:517-30; PMID:24958438; http://dx.doi.org/10.1038/nrg3766
- Deaton AM, Bird A. CpG islands and the regulation of transcription. Genes Dev 2011; 25:1010-22; PMID:21576262; http://dx.doi.org/10.1101/gad.2037511
- Weksberg R, Smith AC, Squire J, Sadowski P. Beckwith-Wiedemann syndrome demonstrates a role for epigenetic control of normal development. Hum Mol Genet 2003; 12(Spec No 1):R61-8; PMID:12668598; http://dx.doi.org/10.1093/hmg/ddg067
- Milani D, Pezzani L, Tabano S, Miozzo M. Beckwith-Wiedemann and IMAGe syndromes: two very different diseases caused by mutations on the same gene. Appl Clin Genet 2014; 7:169-75; PMID:25258553
- Saal HM. Russell-Silver Syndrome. In: GeneReviews®. Pagon RA, Adam MP, Ardinger HH, Bird TD, Dolan CR, Fong CT, Smith RJH, Stephens K, editors. Seattle (WA): University of Washington, Seattle; 2002:1993-2015. Nov 2 [Updated 2011 Jun 2].
- Mackay DJ, Eggermann T, Buiting K, Garin I, Netchine I, Linglart A, de Nanclares GP. Multilocus methylation defects in imprinting disorders. Biomol Concepts 2015; 6:47-57; PMID:25581766; http://dx.doi.org/10.1515/bmc-2014-0037
- Maeda T, Higashimoto K, Jozaki K, Yatsuki H, Nakabayashi K, Makita Y, Tonoki H, Okamoto N, Takada F, Ohashi H, et al. Comprehensive and quantitative multilocus methylation analysis reveals the susceptibility of specific imprinted differentially methylated regions to aberrant methylation in Beckwith-Wiedemann syndrome with epimutations. Genet Med 2014; 16:903-12; PMID:24810686; http://dx.doi.org/10.1038/gim.2014.46
- Azzi S, Rossignol S, Steunou V, Sas T, Thibaud N, Danton F, Le Jule M, Heinrichs C, Cabrol S, Gicquel C, et al. Multilocus methylation analysis in a large cohort of 11p15-related foetal growth disorders (Russell Silver and Beckwith Wiedemann syndromes) reveals simultaneous loss of methylation at paternal and maternal imprinted loci. Hum Mol Genet 2009; 18:4724-33; PMID:19755383; http://dx.doi.org/10.1093/hmg/ddp435
- Williams DH, Gauthier DW, Maizels M. Prenatal diagnosis of Beckwith-Wiedemann syndrome. Prenat Diagn 2005; 25:879-84; PMID:16193463; http://dx.doi.org/10.1002/pd.1155
- Brioude F, Lacoste A, Netchine I, Vazquez MP, Auber F, Audry G, Gauthier-Villars M, Brugieres L, Gicquel C, Le Bouc Y, et al. Beckwith-Wiedemann syndrome: growth pattern and tumor risk according to molecular mechanism, and guidelines for tumor surveillance. Horm Res Paediatr 2013; 80:457-65; PMID:24335096; http://dx.doi.org/10.1159/000355544
- Calvello M, Tabano S, Colapietro P, Maitz S, Pansa A, Augello C, Lalatta F, Gentilin B, Spreafico F, Calzari L, et al. Quantitative DNA methylation analysis improves epigenotype-phenotype correlations in Beckwith-Wiedemann syndrome. Epigenetics 2013; 8:1053-60; PMID:23917791; http://dx.doi.org/10.4161/epi.25812
- Palumbo O, Mattina T, Palumbo P, Carella M, Perrotta CS. A de novo 11p13 microduplication in a patient with some features invoking Silver-Russell Syndrome. Mol Syndromol 2014; 5:11-8; PMID:24550760
- Tee L, Lim DH, Dias RP, Baudement MO, Slater AA, Kirby G, Hancocks T, Stewart H, Hardy C, Macdonald F, et al. Epimutation profiling in Beckwith-Wiedemann syndrome: relationship with assisted reproductive technology. Clin Epigenetics 2013; 5:23; PMID:24325814; http://dx.doi.org/10.1186/1868-7083-5-23
- Chiba H, Hiura H, Okae H, Miyauchi N, Sato F, Sato A, Arima T. DNA methylation errors in imprinting disorders and assisted reproductive technology. Pediatr Int 2013; 55:542-9; PMID:23919517; http://dx.doi.org/10.1111/ped.12185
- Hiura H, Okae H, Chiba H, Miyauchi N, Sato F, Sato A, Arima T. Imprinting methylation errors in ART. Reprod Med Biol 2014; 13:193-202; PMID:25298744; http://dx.doi.org/10.1007/s12522-014-0183-3
- Tabano S, Colapietro P, Cetin I, Grati FR, Zanutto S, Mandò C, Antonazzo P, Pileri P, Rossella F, Larizza L, et al. Epigenetic modulation of the IGF2/H19 imprinted domain in human embryonic and extra-embryonic compartments and its possible role in fetal growth restriction. Epigenetics 2010; 5:313-24; PMID:20418667; http://dx.doi.org/10.4161/epi.5.4.11637
- Oladapo OT. Amniocentesis and chorionic villus sampling for prenatal diagnosis: RHL commentary (last revised: 15 December 2006). The WHO Reproductive Health Library; Geneva: World Health Organization
- Koukoura O, Sifakis S, Spandidos DA. DNA methylation in the human placenta and fetal growth. Mol Med Rep 2012; 5:883-9; PMID:22294146
- Bocangel D, Sengupta S, Mitra S, Bhakat KK. p53-mediated Down-regulation of the Human DNA Repair Gene O6- Methylguanine-DNA Methyltransferase (MGMT) via Interaction with Sp1 Transcription Factor. Anticancer Res 2009; 29:3741; PMID:19846904
- Chiu RW, Chim SS, Wong IH, Wong CS, Lee WS, To KF, Tong JH, Yuen RK, Shum AS, Chan JK, et al. Hypermethylation of RASSF1A in human and rhesus placentas. Am J Pathol 2007; 170:941-50; PMID:17322379; http://dx.doi.org/10.2353/ajpath.2007.060641
- Eroglu A, Layman LC. Role of ART in imprinting disorders. Semin Reprod Med 2012; 30:92-104; PMID:22549709; http://dx.doi.org/10.1055/s-0032-1307417
- Nayeri UA, West AB, Grossetta Nardini HK, Copel JA, Sfakianaki AK. Systematic review of sonographic findings of placental mesenchymal dysplasia and subsequent pregnancy outcome. Ultrasound Obstet Gynecol 2013; 41:366-74; PMID:23239538; http://dx.doi.org/10.1002/uog.12359
- Shiefa S, Amargandhi M, Bhupendra J, Moulali S, Kristine T. First trimester maternal serum screening using biochemical markers PAPP-A and free β-hCG for down syndrome, patau syndrome and edward syndrome. Indian J Clin Biochem 2013; 28:3-12; PMID:24381414; http://dx.doi.org/10.1007/s12291-012-0269-9
- Simoni G, Gimelli G, Cuoco C, Romitti L, Terzoli G, Guerneri S, Rossella F, Pescetto L, Pezzolo A, Porta S, et al. First trimester fetal karyotyping: one thousand diagnoses. Hum Genet 1986; 72:203-9; PMID:3957345.
- White HE, Hall VJ, Cross NC. Methylation-sensitive high-resolution melting-curve analysis of the SNRPN gene as a diagnostic screen for Prader-Willi and Angelman syndromes. Clin Chem 2007; 53:1960-2; PMID:17890436; http://dx.doi.org/10.1373/clinchem.2007.093351