ABSTRACT
The Barcelona Conference on Epigenetics and Cancer (BCEC) entitled “Epigenetic Mechanisms in Health and Disease” was held in Barcelona, October 26-26, 2017. The 2017 BCEC was the fifth and last edition of a series of annual conferences organized as a joint effort of five leading Barcelona research institutes together with B-Debate. This edition was organized by Albert Jordan from the Molecular Biology Institute of Barcelona (IBMB-CSIC) and Marcus Bushbeck from the Josep Carreras Leukaemia Research Institute (IJC). Jordi Bernués, Marian Martínez-Balbás, and Ferran Azorín were also part of the scientific committee. In 22 talks and 51 posters, researchers presented their latest results in the fields of histone variants, epigenetic regulation, and chromatin 3D organization to an audience of around 250 participants from 16 countries. This year, a broad number of talks focused on the epigenetic causes and possible related treatments of complex diseases such as cancer. Participants at the 2017 BCEC elegantly closed the series, discussing progress made in the field of epigenetics and highlighting its role in human health and disease.
Introduction
The BCEC series was conceived with the aim of bringing together scientists from the fields of epigenetics and cancer to share recent findings and to translate knowledge from basic research into the development of new tools for diagnosis and treatment. This interdisciplinary forum has been successfully organized since 2013 as a joint effort of five leading Barcelona institutes in collaboration with the B-Debate initiative of BioCat, supported by the Foundation Obra Social La Caixa (). These institutes are the Biomedical Campus Bellvitge with its Program of Epigenetics and Cancer Biology (PEBC), the Center of Genomic Regulation (CRG), the Institute for Biomedical Research (IRB), the Molecular Biology Institute of Barcelona – Spanish Research Council (IBMB-CSIC) and the Institute of Predictive and Personalized Medicine of Cancer (IMPPC). The IMPPC is now the Program for Predictive and Personalized Medicine of Cancer (PMPPC) at the Institute Germans Trias i Pujol (IGTP), which joined the project in 2016, together with the Josep Carreras Leukemia Research Institute (IJC). The last 2017 BCEC conference was co-organized by B-Debate, the IBMB-CSIC and the IJC. The most relevant content from the 4 previous BCEC editions has been summarized in meeting reports [Citation1–Citation4]. For additional information, please visit our website http://www.bdebate.org/en/debate-series/barcelona-conference-epigenetics-and-cancer-bcec.
Figure 1. BCEC 2017 was the last of five editions that started on 2013 as a joint effort of five Barcelona research institutes and B-Debate.
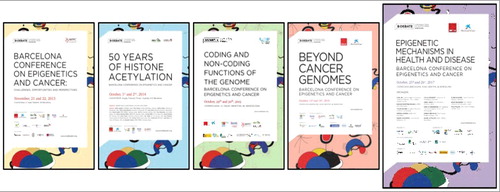
Chromatin is regulated by several epigenetic mechanisms whose alteration results in a variety of complex diseases. The last edition of the BCEC focused on cancer but also included other complex pathologies, all of them discussed from an epigenetics perspective. Here, we provide a brief report of the main results presented at the conference, organized into 4 main topics: Epigenetics in the 3D chromatin structure, Epigenetic mechanisms: histone variants, histone modifications, and DNA methylation, Genomic instability, and Epigenetics and cancer.
Epigenetics in the 3D chromatin structure
In the last years, knowledge in the epigenetics field has increased exponentially thanks to the availability of a myriad of types of genome-wide data [Citation5]. Apart from the extensively studied linear condition of the epigenome, the tridimensional chromatin organization and its consequences are nowadays analyzed at length by experts in the field. Every human cell contains approximately two meters of DNA packaged inside the nucleus. This huge amount of DNA can fit in this organelle due to its intense folding. This folding gives rise to chromosome territories and frequently interacting chromatin called topological associated domains (TADs) [Citation6]. Within TADs the specific localization of DNA will determine the contacts between enhancers and promoters, and this leads to specific gene expression programs [Citation7]. During this B-Debate, the relationships between the chromatin fiber and its 3D-conformation, especially in the context of stem cells, were addressed. In the following lines, we will point out to the main conclusions of the invited speakers.
It is known, that during the switch between naïve and primed pluripotency in embryonic stem cells (ESC) many epigenetic changes occur. These changes can be triggered in vitro by using media supplemented with MEK and GSK3 kinase inhibitors (2i, naïve state) or media supplemented with serum and LIF (primed state) [Citation7]. Hendrik Stunnenberg (University of Radboud) explores the epigenetic profiles in ESC during the transition between naïve and primed pluripotency. During his talk, he explained that pluripotency factors are equally expressed in both cell states; however, lineage specific factors and bivalent promoters only appear in the primed state [Citation8]. Another difference between the mentioned ESCs is the duration of the cell cycle, in the case of the 2i condition, the G1 phase is longer and the G2 phase shorter. Interestingly, in the serum+LIF treated cells, a number of interactions between chromosomes appear. These interactions were called extreme long-range interactions (ELRI) and involve H3K27me3-marked loci. Except for these ELRI, Professor Stunnenberg remarked that the 3D chromatin structure was similar between the two ESC states.
Chromatin modifying enzymes permit the activation and repression of genes. In this context, one of the most studied repressors is the Polycomb Group (PcG) of proteins. Two main PcG complexes operate on the genome: PRC1 and PRC2. These complexes exert their repressive functions over many developmental loci by depositing H3K27me3 (PRC2) [Citation9] and compacting chromatin (PRC1) [Citation10]. Wendy Bickmore (University of Edinburgh), well-known for being one of the researchers that has shed light into the mechanisms of nuclear organization, addressed the role of the different PcG members in the regulation of chromatin compaction. By performing experiments using a mutant version of RING1B that is unable of interacting with E2 UBCH5C and ablates the capacity of RING1B to act as an E3 ligase in vitro (RING1B I53A), she demonstrated how the ubiquitin-ligase catalytic activity of the PRC1 subunit RING1B is dispensable for chromatin compaction in mouse embryonic stem cells (mESC) [Citation11]. Furthermore, Professor Bickmore showed that loci repressed by PRC2 are clustered by PRC1. Using the 2i vs. serum+LIF ESC model she demonstrated that Polycomb is redistributed in naïve pluripotency generating DNA decompaction and ultimately nuclear re-organization. This is due to the lack of DNA methylation and not to the naïve state per se. This implies that DNA methylation impacts nuclear organization even though it occurs through PRC proteins.
Commitment of ESC to the neural lineage is essential to give rise to the different nervous system cell subtypes. The neural stem cells (NSC) in vitro system allows the study of developmental mechanisms without the limitation of cell number [Citation12]. Marian Martínez-Balbás (Molecular Biology Institute of Barcelona) has long been studying the epigenetic mechanisms that occur upon different signaling pathways in the neural context [Citation13–Citation15]. In her talk, Dr. Martínez-Balbás analyzed the role of the histone demethylase JMJD3 in the activation of NSC enhancers. She demonstrated that TGFβ-signaling induces the activation of a subset of enhancers related to neural development. Upon TGFβ treatment, the effector of the pathway SMAD3 recruits the histone demethylase JMJD3 and by coordinating the activity of JMJD3 and the chromatin remodeler CHD8, promotes the transcription of enhancer RNAs, which correlates with gene activation. Notably, the proneural factor ASCL1 was demonstrated to be essential in the targeting of SMAD3 to the enhancers. One of the most elegant results that she presented was a neuronal differentiation experiment performed with wild type and Neurog2-enhancer CRISPR-Cas9-engineered NSC. Interestingly, NSC uniquely lacking the enhancer were not able to differentiate into neurons, concluding that TGF-responsive enhancers through contacts with promoters are essential for neuronal commitment.
Remarkably, not only the proteins that mediate contacts between genomic regions impact nuclear organization, post-translational modifications of structural proteins might also affect the 3D-structure of the genome. CTCF and Cohesin complex control the functional architecture of the mammalian genome [Citation16]. Kunal Rai (MD Anderson Cancer Center, University of Texas) studies how epigenetic mechanisms influence cancer progression. Concretely, his lab is interested in melanoma tumor progression [Citation17]. Along his short talk he showed the results of a screening in which he found that HDAC8 histone deacetylase has prometastatic roles. Interestingly, the deacetylation of histones by HDAC8 does not seem to be the driving force in metastasis. However, HDAC8 deacetylates SMC3, a subunit of the Cohesin complex. By doing this, HDAC8 is impacting the high-order structure of the chromatin and thus promoting melanoma metastasis. Another interesting aspect of the 3D-organization is how insulators participate in transcriptional regulation, being essential to avoid disease [Citation18]. Addressing this topic, the short talk given by Lluís Montoliu (National Centre for Biotechnology, CNB, Madrid) shed light into the mechanisms leading to albinism. Along his talk, he presented an in vivo functional analysis of regulatory elements of the mouse Tyrosinase (Tyr) gene, which encodes for the key enzyme in melanin synthesis. He summarized previous work using transgenes carrying constructs containing the mouse Tyr gene and neighboring regions, which enabled the discovery of key regulatory elements [Citation19–Citation21]. Further analysis in the endogenous Tyr locus using CRISPR-Cas9 technology showed that deletion of Tyr 5’ boundary element, a control region with insulator properties, causes a decrease in Tyr expression, thus leading to albino phenotypes [Citation22].
Even though most of the talks in this B-Debate dealt with transcription, DNA replication, an essential process that ensures the duplication of the genetic material during the cell cycle, was also discussed [Citation23]. Alexander Mazo (Thomas Jefferson University, Philadelphia) was selected for a short talk in which he led the attention into the importance of the nascent post-replicative chromatin [Citation24,Citation25]. In his talk, Professor Mazo explained that stem cells differentiation requires de-condensation of the nascent chromatin, as the presence of H3K27me3 prevents the binding of lineage specific transcription factors. One application of this discovery is the manipulation of the post-replicative chromatin with epigenetic inhibitors in order to avoid transcription factor binding. Concretely, promoting H3K27me3 acquisition by inhibiting H3K27me3 demethylases JMJD3 and UTX prevents Myocardin-related transcription factor A (MRFTA) binding, thus preventing myofibroblast differentiation and ultimately fibrosis during cataract surgery. Another application of epigenetics in in the treatment of pathological processes was discussed in a short talk by Guillaume Filion (CRG, Barcelona). HIV virus integration and latency is epigenetically modulated. Dr. Guillaume Filion presented the barcoded HIV ensembles (B-HIVE) method to identify chromosomal locations of individual proviruses and track their transcription. Interestingly, HIV proviruses are not randomly inserted in the genome and are mainly found in active genes, promoters, and enhancers. Those with high expression coincide with active TADs, independently of their genic or intergenic location [Citation26]. In addition, provirus-activating drugs, such as bromodomain or histone deacetylase inhibitors can be easily compared by the B-HIVE method by knowing their specific subset of activated proviruses: different drugs activate different subsets of proviruses. In this way, even new drugs that would act through the same epigenetic mechanism could be discovered.
Epigenetic mechanisms: Histone variants, histone modifications, and DNA methylation
Chromatin can be functionally specialized by the action of several epigenetic mechanisms. Among them, histones variants have been the subject of an increasing number of studies since their misregulation is associated with several complex diseases [Citation27]. The 2017 BCEC covered progress made in the functional characterization of certain histone variants, including H3 variant CENP-A, which specializes the chromatin at centromeres and is required for kinetochore assembly [Citation28]; H3.3 variant, which has been proposed to participate in the epigenetic transmission of active chromatin states [Citation29]; H2A.Z variant, implicated in establishing transcriptional competence and in counteracting heterochromatic silencing [Citation28]; and some isoforms of the linker histone H1, the most divergent histone protein, with somatic and germline variants specifically involved in different processes such as transcriptional regulation, cell cycle regulation, heterochromatic silencing, genome stability, and zygotic genome activation [Citation30–Citation36]. Several talks also highlighted the importance of cross-talk between different epigenetic mechanisms including histone variants, histone posttranslational modifications (PTMs), DNA methylation, and long noncoding RNAs (lncRNAs). The following section summarizes the main results presented on this field.
DNA methylation is a well-established epigenetic mark involved in gene regulation and genome stability [Citation37]. Several de novo DNA methyltransferase (DNMT) isoforms exist that could play important roles during development and disease. During his short talk, Tuncay Baubec (University of Zurich, Switzerland) demonstrated that the long isoform of DNMT3A, DNMT3A1, co-localizes with the epigenetic mark H3K27me3 at CpG island promoters, whereas the shorter DNMT3A2 isoform or the paralog DNMT3B do not display this preference. Furthermore, DNMT3A1 shows a site-specific co-localization with 5-hydroxymethylcytosine (5hmC) deposition at CpG island shores. A deep analysis revealed that DNMT3A1 binding coincides with DNA methylation turnover: DNMT3A1 is preferentially recruited at bivalent CpG islands to counteract the activity of TET enzymes and to demarcate the transition between methylated and unmethylated CpGs at the borders of CpG islands. Dr. Baubec discussed that DNMT3A1 recruitment plays a role in de novo DNA methylation at CpG island shores with further implications on H3K27me3 distribution and H3K27me3-mediated regulation of developmental genes [Citation38].
During mitosis, chromosomes acquire a highly packaged structure and become functionally inert; when this process ends, chromosomes rapidly decondense to allow regulated access to functional elements, leading to regular genomic functions, such as transcription. In his talk, Amos Tanay (The Weizmann Institute, Israel) explained his studies of single cell Hi-C, which have the aim of deeply understand chromosome conformation at the single cell level in order to avoid cell heterogeneity. In this way, Dr. Tanay analyzed the impact of the cell cycle on the 3D-chromatin organization. TADs are controlled by distinct cell cycle dynamics: mitotic nuclei tend to be enriched in contacts around 2 to 12 Mb, whereas proliferating nuclei are enriched in contacts of less than 1 Mb. Therefore, by looking at the genomic contacts distribution of each nucleus and combining it with replication analysis, a map is generated in which G1 cell cycle phase can be clearly differentiated from S phase as replication is gaining strength [Citation39]. In the second part of the talk, unpublished data about single cell methylomes analysis was shown. Briefly, this data seems to indicate that there is a general maintenance of cis-epigenetic memory as there are many epialleles that become very frequent in a single cell population analysis. Nevertheless, during embryogenesis, DNA methylation seems to act as a poor cis-transmitter of epigenetic memory due to its dynamic characteristics.
The interaction of lncRNA with chromatin has been described as another epigenetic mechanism, which modulates gene expression. Single-stranded RNA from lncRNA form triple-helix structures with the major groove of double-stranded DNA, via the so-called Hoogsteen bonds. Triplexes have been frequently found in regulatory regions, especially at promoters, and several reports have analyzed functional roles of lncRNA modulating chromatin structure by recruiting Polycomb proteins or regulating gene-specific methylation (among others, [Citation40–Citation43]). Shortly, Rodrigo Maldonado (University of Regensburg, Germany) showed that nucleosomes stabilize triplex formation, mainly through histone tails, especially through the histone H3 tail. In addition, triplexes are preferentially located in the linker DNA adjacent to nucleosomes and genome-wide analyses revealed that they are enriched in active chromatin. Regarding the functions exerted by histone variants, epigenetic specification of centromeres is critical for organismal viability, as it ensures the accurate segregation of sister chromatids. Centromeres are specified by the centromere-specific histone CENP-A. However, the mechanisms that regulate CENP-A deposition are not well understood [Citation44]. In this regard, Alison Pidoux (University of Edinburgh, UK) presented work performed in Schizosaccharomyces about the intrinsic properties of centromere DNA to promote the establishment of CENP-A chromatin. She first summarized previous work indicating that it is not the centromere DNA sequence what is attracting CENP-A but the particular environment that this centromere DNA creates [Citation45–Citation47]. To understand the properties of the central core centromere DNA and if these properties are conserved, they generated minichromosomes containing S. octosporus centromere DNA core regions in order to analyze their segregation function in S. pombe. By this elegant approach, they demonstrated that central core regions exhibit cross-species functionality that does not rely on sequence conservation. In addition, they studied the nucleosome occupancy and the histone turnover of an ectopic central core region assembled in H3 chromatin. The results presented suggest that central core DNA confers dynamic properties on the H3 chromatin resulting in continual turnover and low nucleosome occupancy that promote the establishment of a CENP-A landscape. Following with the epigenetics of the centromere, Sylvia Erhardt (ZMBH, Heidelberg, Germany) also presented work about CENP-A, this time on the model organism Drosophila. Using stem cells of the Drosophila midgut (ISCs), which undergo asymmetrical cell division, she showed that CENP-A and the essential non-histone protein CENP-C are differentially incorporated to centromeres in stem cells. These observations were done in a genetic system where newly synthesized proteins can be labeled differentially from already existing CENP-A protein by using a short heat shock. Upon asymmetrical cell division, newly synthesized and pre-existing CENP-A are segregated differentially, in accordance with an epigenetic mark responsible for maintaining stem cells properties. She also observed that, in addition to CENP-A and CENP-C, other centromere components are required for ISCs proliferation and also have a role in post-mitotic cells and cells undergoing endoreplication. Such results pointed to a new non-centromeric role for kinetochore components, especially for the centromeric-specific histone variant CENP-A.
The EMBO keynote lecturer, Carl Wu (John Hopkins Hospital, Baltimore, USA), discussed the highly conserved histone variant H2A.Z, which has a key role in transcription regulation by its incorporation at eukaryotic enhancer and promoter regions. How histone H2A.Z is selectively incorporated into promote-flanking nucleosomes genome-wide and how approximately 40% specific amino acids dictate its function are outstanding questions. Dr. Wu showed that budding yeast H2A.Z-H2B replacement is a step-wise process catalyzed by SWR1, a 14-component, 1 MDa chromatin remodeler. In an ATP-dependent process, SWR1 mediates H2A-H2B dimer eviction and incorporation of H2A.Z-H2B followed by a second dimer exchange [Citation48,Citation49]. Thus, SWR1 has two substrates: canonical nucleosomes and the variant H2A.Z dimer. In addition, he showed that the Swc2/YL1 subunit, within the SWR1 complex, is responsible for nucleosome binding and also for the capture of H2A.Z-H2B dimers for histone exchange [Citation50]. Also, domain-swapping experiments revealed the H2A and H2A.Z amino acids responsible for SWR1 activation. Free DNA found at nucleosome-free regions in promoters dominates SWR1 recruitment, which preferentially binds long-liker nucleosomes with increased affinity for acetylated dinucleosomes. SWR1 binding and ATPase activity does not change nucleosome position but apparently alters nucleosomal DNA/histone conformation to disrupt histone-DNA and histone-histone contacts [Citation51]. Another remaining question is how H2A.Z variant is evicted after its incorporation. To answer that, Carl Wu developed a new approach to detect protein dynamics using single-molecule, real-time fluorescence imaging in live yeast cells. SWR1 and H2A.Z were fused to HaloTag and the frequency of slow and fast diffusive states and their duration were assessed in several mutant strains of budding yeast. Double conditional mutants of SWR1 and the RNA polymerase machinery suggest that RNA polymerase II, rather than chromatin remodelers like INO80 or SWR1 itself, has an important role in the eviction of H2A.Z. The link between H2A.Z eviction and RNA polymerase II activity has intriguing implications for the presence of cryptic unstable RNAs at promoter regions genome-wide. Further experiments, using live-cell imaging of other HaloTag fusions, are being performed to reveal the intrinsic dynamics and epigenetic regulation of the RNA polymerase II preinitiation complex.
The deposition and removal of specific histone variants along the genome provides chromatin with an effective additional level of regulation. Consequently, many reports are linking misexpression of histone variants with tumorigenesis [Citation33,Citation52]. Eran Meshorer (Hebrew University of Jerusalem) works on the role of histone variants H1.0 and H3.3 in the context of development and cancer. During his talk, Dr Meshorer explained two different projects in which the variation in the dose of a specific histone variant was correlated to cancer status. By performing a massive ChIP-seq experiment in which the main goal was to monitor H3.3 turnover, his results demonstrated that genes expressed in ESC carried a hyper dynamic H3.3-containing nucleosome at the -1 position that surprisingly moves to the +1 position upon differentiation [Citation53]. In the second part of his talk, Dr. Meshorer, in collaboration with Dr. Paola Scaffidi (Crick Institute, UK), analyzed the expression levels of histone H1.0 variant in the different cell types present in tumors. He observed that cancer stem cells (CSCs) have less H1.0 and the absence of the variant is related to cancer cell differentiation and poor prognosis. The mechanism behind this characteristic is that H1.0 might be repressing the oncogenic program. The absence of H1.0 leads to a destabilization of nucleosome-DNA interactions in GC poor regions and this remodeler activity generates open chromatin regions that might trigger the activation of the contained oncogenic genes [Citation54]. All together, Dr. Meshorer delineated two different mechanisms by which histone variants contribute to cell malfunction.
H1 histones are less conserved than core histones and most species contain multiple H1 variants that play redundant as well as specific functions during development and differentiation [Citation33]. Drosophila has a low histone H1 complexity with only one somatic (dH1) and one germline specific (dBigH1) variant. Ferran Azorin (Molecular Biology Institute of Barcelona) summarized his studies addressing the functional characterization of Drosophila linker histones. He presented published data related to the role of dBigH1 in preventing premature activation of the zygotic genome during early embryogenesis [Citation35]. He also detailed their recent findings regarding a novel dBigH1 function during spermatogenesis. Specifically, dBigH1 acts as a general silencing factor in spermatocytes, where it represses Bam, a key translational regulator that dictates the switch from spermatogonial proliferation to spermatocyte differentiation. Bam, in turn, also represses dBigH1 during spermatogonial divisions. This double-repressor mechanism is crucial for the correct progression into spermatocyte differentiation [Citation55]. In the second part of his talk, he presented their recent findings on the essential contribution of dH1 to the maintenance of genomic stability [Citation30].
Genomic instability
Proper establishment and maintenance of the epigenetic landscape is crucial to avoid high rates of mutations and chromosomal rearrangements. Genomic instability is triggered by deregulation of critical cellular processes, such as DNA replication, DNA repair, and S-phase checkpoints. Another source of instability comes from highly transcribed DNA regions or fragile sites [Citation56]. Consequently, proper chromatin structure and function is important to control those processes that, when deregulated, can lead to age-related diseases or cancer [Citation57–Citation59]. Among all genomic regions, heterochromatin must be properly controlled, due to its role in repressing repetitive sequences and the inactive X chromosome, its control of telomeres and centromeres, and its tough prone-error DNA replication [Citation60,Citation61]. Thus, great efforts are focused in elucidating the epigenetic causes of genomic instability and implicated proteins or processes.
Dr. Azorín contributed to this challenging field by studying the role of dH1 in preventing R-loops-induced DNA damage in heterochromatin. He showed that dH1 depletion induces an increase in γH2Av levels and a high incidence of DNA breaks and sister-chromatid exchanges. This increased γH2Av is due to the accumulation of DNA:RNA hybrids (R-loops) and occurs preferentially at heterochromatic elements, which are derepressed upon dH1 depletion [Citation30]. Dr. Azorín reasoned that these defects, which induce JNK-mediated apoptosis, are specific of dH1 depletion since they are not observed when heterochromatin silencing is relieved by HP1a depletion. Apart from linker histone dH1, Tousled like kinases 1 and 2 (TLK1/2), which facilitate core histones H3/H4 deposition by phosphorylating the chaperone ASF1 have been shown to have a role in preventing genomic instability. Shortly explained by Travis Stracker (IRB, Barcelona, Spain), TLK1/2 have been previously demonstrated to have higher activity in S-phase, being regulated by the ATR-Chk1 pathway and linked to DNA repair and transcription. He explained that TLK1/2 depletion compromises histone deposition, leading to genomic instability due to replication stress, DNA damage, and decondensation of heterochromatic regions. These events result in the increased transcription of some repetitive regions, telomere lengthening, and the triggering of an antiviral response.
Specific histone modifications also play a role in the maintenance of genomic stability. Histone H3K9 methylation is a conserved mark that correlates broadly with gene repression and is highly enriched in repetitive elements [Citation60]. Susan Gasser (Friedrich Miescher Institute for Biomedical Research, Switzerland) provided an extensive overview on the role of this heterochromatic mark in stabilizing repetitive elements in C. elegans. Her team has taken advantage of the fact that C. elegans has only two nonredundant H3K9 histone methyltransferases (HMTs), and that mutants lacking both H3K9-HMTs are completely devoid of H3K9 methylation. She showed that the met-2 set-25 double mutants developed temperature-dependent loss of fertility. This defect, linked to germline apoptosis, is caused by DNA damage. RNA-seq data indicated that loss of H3K9 methylation causes a de-repression of genes and repetitive elements [Citation62]. Professor Gasser elaborated on the link between the aberrant transcription of repetitive elements and the DNA damage generated. Concretely, met-2 set-25 double mutants accumulated R-loops, an important source of replication stress. Moreover, they found R-loop enrichment in regions containing accumulation of insertions and deletions at repetitive sequences [Citation62]. In the last part of her talk, Professor Gasser discussed recent unpublished data related to other pathways acting synergistically with H3K9 HMTs. They performed an RNAi-based screen to identify genes inducing synthetic lethality in combination with H3K9-HMTs mutants. Among the positive hits they found the BRCA1 complex. BRCA1 depletion alone induced a specific de-repression of tandem repeats, which is concomitant with a decrease of H2A ubiquitination and a mild decrease of H3K9me2. The results Dr. Gasser presented suggest that BRCA1 acts synergistically with met-2 to ensure the stability of repeat-rich genomes, most specifically by suppressing the transcription of simple repeats.
Histone acetylation is crucial for DNA damage response (DDR) and loss of functional DDR is another well-known source of genomic instability. In this regard, Kyle Miller (University of Texas, USA) summarized his work on the characterization of epigenetic readers of this mark involved in DDR. His group used a technique based on laser microirradiation to study protein dynamics of human proteins containing the primary reader domain for acetyl-lysines, the Bromodomain (BRD). They found that around one third of the 42 human BRD proteins are recruited to DNA damage sites. He then presented the functional characterization of ZMYND8. Among ZMYND8 interactors they found members of the NuRD chromatin remodeling complex. NuRD complex is targeted to damaged sites and its core member, CHD4, participates in double-strand break (DSB) repair by homologous recombination (HR) [Citation63,Citation64]. In addition, he discussed recently published data exploring the functional relationship with another ZMYND8 interactor, the KDM5A demethylase. They found that KDM5A-dependent H3K4me3 demethylation within chromatin near DSB sites is crucial for subsequent ZMYND8-NuRD recruitment [Citation65]. Finally, Dr. Miller discussed a challenging ongoing project that aims to characterize the BRD interactome by generating a collection of stable cell lines carrying all the genes encoding for BRD proteins. They will subsequently identify new protein interactors by immunoprecipitation-mass spectrometry (IP-MS). Undoubtedly, this data would be very useful to understand how the inhibition of some BRD proteins, a strategy used in cancer therapy, affects the function of these DDR pathways.
Epigenetics and cancer
One of the best-known epigenetic marks is DNA methylation, which was already demonstrated in the 1940s to be differentially present in normal vs. tumor tissue [Citation66,Citation67]. It has long been known that hypermethylation of CpG islands in promoter regions of tumor suppressor genes might be appearing at early stages of many cancers. This hypermethylation can potentially impair the expression of genes involved in different basic cell functions, such as DNA repair, proliferation, or apoptosis, among others [Citation66,Citation68,Citation69]. During the development of a neoplasm, the degree of genomic DNA hypomethylation increases as the lesion progresses from a benign to an invasive state [Citation66,Citation68]. In addition, not only DNA methylation but also histone variants and histone modifications should be taken into account when a tumor appears. As mentioned in previous sections, disruption of the 3D-chromatin structure, the epigenetic machinery, or the mechanisms involved in the maintenance of genome stability can lead to malignant cellular transformation. Specifically, along this section we will summarize the talks that focused on current efforts in the field aiming to elucidate potential roles and applications of epigenetics in the diagnosis, progression, and treatment of many cancer types.
Germinal center (GC) B-cells are characterized by rapid proliferation and somatic hypermutation, and a majority of B-cell neoplasms, including diffuse large B-cell lymphoma (DLBCL), originate from the GC reaction. Wendy Béguelin (Weill Cornell Medical College, USA) presented results that contribute to understand the epigenetic landscape of GC-derived lymphomas. It has been widely reported that GC B cells display high upregulation of EZH2, a core component of PRC2. In 2013, Dr. Wendy Béguelin described that EZH2 represses genes related to B-cell differentiation and cell cycle checkpoint, and how EZH2 deletion results in the inhibition of GC formation and functions [Citation70]. More recently, she reported that the action of EZH2 in driving GCs and lymphoma precursor lesions required the cooperation of transcriptional repressor BCL6 to recruit a non-canonical PRC1 complex, which is formed with the chromodomain protein CBX8. CBX8 binds to H3K27me3 at bivalent promoters and contributes to stabilize this complex. Moreover, with these studies she also described the malignant effect of both BCL6 and EZH2 in the development of DLBCL and how the anti-lymphoma activity was enhanced by targeting these proteins in a combinatorial approach [Citation71]. During her talk, she has also demonstrated that EZH2 mediates GC formation through repression of p21, a cyclin-dependent kinase inhibitor, by using a 3D B-cell follicular organoid system that mimics the GC reaction [Citation72].
Erythropoiesis is a dynamic process regulated by several factors, such as changes in cell surface protein expression, progressive hemoglobinization, and nuclear condensation. As it occurs in other cell types, erythrocyte formation is regulated by distinct transcription factors and signaling pathways. The importance of erythropoietin receptor (EpoR) signaling through Janus kinase 2 (JAK2) tyrosine kinase and STAT5A/B in the regulation of red blood cell production has been reported [Citation73]. Florian Heidel (Leibniz Institute on Aging, Jena University Hospital, Germany) explained how Plcg1, which is directly activated by phosphorylation of Jak-dependent kinases in leukemia development, plays a key role in erythroid differentiation, as its inactivation completely blocks this process. Moreover, with the aim of identifying Plcg-related molecules involved in the regulation of erythroid differentiation, changes in expression and methylation of several genes were evaluated. H2afy2 gene, which codifies for the histone variant macroH2A2 (mH2A2), became the top Plcg1 downstream effector. In this way, inactivation of mH2A2 expression also caused the depletion of erythroid maturation, mimicking the effect of lack of Plcg1. These results point to Plcg1 and mH2A2 as members of a potential non-canonical erythropoietin signaling pathway essential for erythroid differentiation [Citation74]. On the other hand, Professor Florian Heidel demonstrated that Plcg1 is highly expressed in the presence of the oncogene AML1-ETO. Moreover, Plcg1 is a direct target of AML1-ETO, as it is required for the self-renewal capacity leukemia stem cells (LSCs) in vitro and in vivo. In addition, by analyzing transcriptomes, he postulated that loss of Plcg1 results in the differentiation of LSCs containing AML1-ETO oncogene ex vivo.
The arrival of single cell technologies enabled the study of the impact of cell-to-cell variability in driving phenotypic variation [Citation75]. Pernette Verschure (University of Amsterdam, the Netherlands) gave a short talk with the central topic based on the importance of single-cell epigenetics and transcription dynamics. The Verschure group noted that transcription repression follows an all-or-none trend. Using reporter cassette-containing cells enabling epigenetic modulation of chromatin and visualization of transcription dynamics in single cells, they found that transcription repression proceeds after a relatively long transition period. The decision to become repressed is highly dependent on the epigenetic chromatin structure in individual cells. When the epigenetic threshold to become repressed is passed, transcripts are rapidly dropped from the active gene with a half-life that is similar in all cells. Moreover, Professor Verschure presented a novel therapy-specific mechanism underlying estrogen receptor positive breast cancer acquired resistance, a study performed in the context of EU international consortium entitled EpiPredict focusing on the role of epigenetic regulation in the development of resistance to therapy in breast cancer. The researchers discovered that activation of one specific gene (CYP19A1) switches on estrogen production in tumor cells such that the cells stop responding to the hormonal therapy and patients develop metastatic breast cancer. Verschure et al. managed to microscopically identify the disrupted cells using single molecule RNA FISH, a method that enables to visualize single cells at a resolution level of a few molecules. These findings suggest that an as-yet unknown mechanism is behind the development of early-phase aromatase inhibitor resistance [Citation76].
The study of DNA methylation has attracted many researchers trying to elucidate its changing pattern in many neoplasias. The methylome (the set of nucleic acid methylation modifications in an organism's genome or particular cell) presents interesting variability from healthy individuals to patients [Citation37]. In this regard, Manel Esteller (Bellvitge Biomedical Research Institute, Barcelona), mainly known for his studies of DNA methylation and cancer, discussed his latest results in this field. Interestingly, he observed intratumor methylome changes, showing a more dynamic and adapting process in the invasive front. Indeed, Professor Manel Esteller indicated that by knowing the DNA methylation status of a metastatic cell, its primary tumor can be inferred, a key point in medical diagnosis and patient survival [Citation77]. Several cancer drugs targeting epigenetic proteins involved in DNA methylation, histone acetylation and methylation are already in the market [Citation78]. Others, such as bromodomain (BET) inhibitors, are in clinical trials. Bromodomain-containing proteins bind to acetylated lysine residues of histones, recruiting specific transcription factors and/or chromatin structure regulators. Specifically, treatment with the BET JQ1 in mice and human luminal breast cancer cell lines was shown to reduce tumor size and volume, increasing survival [Citation79]. Knowing that the epigenetic landscape is specifically dysregulated in every tumour and that specific multidrug prescription is crucial for prognosis, professor Esteller presented a study analyzing genome-wide DNA methylation, mutations and amplifications and deletions of 1,000 cell lines and 11,200 patients screened against 265 compounds, providing a powerful tool for cancer pharmacogenomics [Citation80]. Intriguingly, Professor Esteller pointed to another key theme in studying cancer beyond DNA: the epitranscriptome, which describes the chemical modifications of RNA that are found altered in cancer. Epitranscriptomics adds a new layer to the complex study of cancer, paving new paths for the future of cancer molecular medicine [Citation81].
Conclusions
The 5th BCEC entitled Epigenetic Mechanisms in Health and Disease was the last of these annual series that, during 5 years, gathered pioneering scientific experts of the chromatin field. The BCEC series have been a remarkable privilege and a chance to discuss with worldwide experts the latest and novel discoveries of epigenetics as an essential mechanism in normal cell function and its key implication in many diseases such as cancer. Barcelona hosts a compelling research community in epigenetics; hence, from their very beginning, these series have been a great success with more than 1,000 participants and 100 speakers, offering young researchers the opportunity to approach leaders in the field and where strategic decisions could also be pondered. Without doubt, BCEC has contributed to reinforce epigenetic research in our country ( and ).
Figure 2. Some of the speakers, organizers, and chairs from the fifth edition of BCEC, entitled “Epigenetic Mechanisms in Health and Disease”, held in Barcelona, October 25–26, 2017.
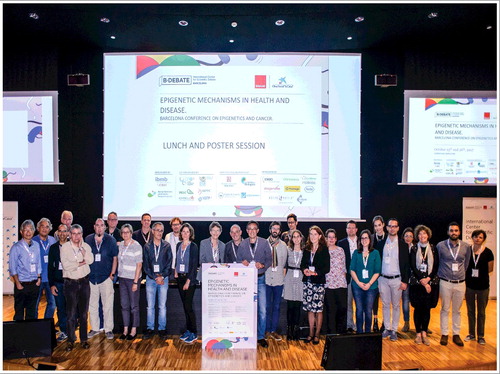
Whether BCEC series will endure either in their present format or in an alternative arrangement is not clear; in any case, the continuity of BCEC series would be valuable. We highlight the necessity of scientific debate in order to rigorously solve biomedical challenges and hope that the ever-increasing epigenetics field keeps on developing knowledge and tools to improve future medicine.
Disclosure of potential conflicts of interest
No potential conflicts of interest were disclosed.
Acknowledgments
We want to acknowledge personnel from B-Debate, Biocat and la Caixa foundation for their contribution to the organization of BCEC series, with a special mention to Marta Soler and María Ruiz for their enormous contribution to BCEC2017.
Additional information
Funding
References
- Corujo D, Mas G, Malinverni R, et al. Barcelona conference on epigenetics and cancer 2015: Coding and non-coding functions of the genome. Epigenetics. 2016;11(1):95–100. doi:10.1080/15592294.2015.1131377. PubMed PMID: 26996885; PubMed Central PMCID: PMC4846135.
- Dumbovic G, Biayna J, Font B, et al. Barcelona conference on epigenetics and cancer 2016 – beyond cancer genomes. Epigenetics. 2017 Mar 4;12(3):238–245. doi:10.1080/15592294.2017.1281503. PubMed PMID: 28121228; PubMed Central PMCID: PMC5406209.
- Palau A, Perucho M, Esteller M, et al. First barcelona conference on epigenetics and Cancer. Epigenetics. 2014 Mar;9(3):468–475. doi:10.4161/epi.27759. PubMed PMID: 24413145; PubMed Central PMCID: PMC4053465.
- Perez-Salvia M, Simo-Riudalbas L, Ausio J, et al. Barcelona conference on epigenetics and Cancer: 50 years of histone acetylation. Epigenetics. 2015;10(5):446–451. doi:10.1080/15592294.2015.1039222. PubMed PMID: 25942103; PubMed Central PMCID: PMC4622646.
- Consortium EP. An integrated encyclopedia of DNA elements in the human genome. Nature. 2012 Sep 6;489(7414):57–74. doi:10.1038/nature11247. PubMed PMID: 22955616; PubMed Central PMCID: PMC3439153.
- Rowley MJ, Corces VG. The three-dimensional genome: principles and roles of long-distance interactions. Curr Opin Cell Biol. 2016 Jun;40:8–14. doi:10.1016/j.ceb.2016.01.009. PubMed PMID: 26852111; PubMed Central PMCID: PMC4887315.
- Andrey G, Mundlos S. The three-dimensional genome: regulating gene expression during pluripotency and development. Development. 2017 Oct 15;144(20):3646–3658. doi:10.1242/dev.148304. PubMed PMID: 29042476.
- Atlasi Y, Stunnenberg HG. The interplay of epigenetic marks during stem cell differentiation and development. Nat Rev Genet. 2017 Nov;18(11):643–658. doi:10.1038/nrg.2017.57. PubMed PMID: 28804139.
- Di Croce L, Helin K. Transcriptional regulation by Polycomb group proteins. Nat Struct Mol Biol. 2013 Oct;20(10):1147–1155. doi:10.1038/nsmb.2669. PubMed PMID: 24096405.
- Shao Z, Raible F, Mollaaghababa R, et al. Stabilization of chromatin structure by PRC1, a Polycomb complex. Cell. 1999 Jul 9;98(1):37–46. doi:10.1016/S0092-8674(00)80604-2. PubMed PMID: 10412979.
- Illingworth RS, Moffat M, Mann AR, et al. The E3 ubiquitin ligase activity of RING1B is not essential for early mouse development. Gene Dev. 2015 Sep 15;29(18):1897–1902. doi:10.1101/gad.268151.115. PubMed PMID: 26385961; PubMed Central PMCID: PMC4579347.
- Conti L, Cattaneo E. Neural stem cell systems: physiological players or in vitro entities? Nat Rev Neurosci. 2010 Mar;11(3):176–187. doi:10.1038/nrn2761. PubMed PMID: 20107441..
- Fueyo R, Garcia MA, Martinez-Balbas MA. Jumonji family histone demethylases in neural development. Cell Tissu Res. 2015 Jan;359(1):87–98. doi:10.1007/s00441-014-1924-7. PubMed PMID: 24950624.
- Akizu N, Estaras C, Guerrero L, et al. H3K27me3 regulates BMP activity in developing spinal cord. Development. 2010 Sep 1;137(17):2915–2925. doi:10.1242/dev.049395. PubMed PMID: 20667911.
- Estaras C, Akizu N, Garcia A, et al. Genome-wide analysis reveals that Smad3 and JMJD3 HDM co-activate the neural developmental program. Development. 2012;139(15):2681–2691. doi:10.1242/dev.078345. PubMed PMID: 22782721.
- Merkenschlager M, Nora EP. CTCF and cohesin in genome folding and transcriptional gene regulation. Annu Rev Genomics Hum Genet. 2016 Aug 31;17:17–43. doi:10.1146/annurev-genom-083115-022339. PubMed PMID: 27089971.
- Fiziev P, Akdemir KC, Miller JP, et al. Systematic Epigenomic analysis reveals chromatin states associated with melanoma progression. Cell Rep. 2017 Apr 25;19(4):875–889. doi:10.1016/j.celrep.2017.03.078. PubMed PMID: 28445736; PubMed Central PMCID: PMC5473172.
- Ali T, Renkawitz R, Bartkuhn M. Insulators and domains of gene expression. Curr Opin Genet Dev. 2016 Apr;37:17–26. doi:10.1016/j.gde.2015.11.009. PubMed PMID: 26802288.
- Ganss R, Montoliu L, Monaghan AP, et al. A cell-specific enhancer far upstream of the mouse tyrosinase gene confers high level and copy number-related expression in transgenic mice. The EMBO J. 1994 Jul 01;13(13):3083–3093. PubMed PMID: 8039502; PubMed Central PMCID: PMC395199.
- Giraldo P, Montoliu L. Artificial chromosome transgenesis in pigmentary research. Pigm Cell Res. 2002 Aug;15(4):258–264. doi:10.1034/j.1600-0749.2002.02030.x. PubMed PMID: 12100491.
- Montoliu L, Umland T, Schutz G. A locus control region at -12 kb of the tyrosinase gene. The EMBO J. 1996 Nov 15;15(22):6026–6034. PubMed PMID: 8947025; PubMed Central PMCID: PMC452424.
- Seruggia D, Fernandez A, Cantero M, et al. Functional validation of mouse tyrosinase non-coding regulatory DNA elements by CRISPR-Cas9-mediated mutagenesis. Nucleic Acids Res. 2015 May 26;43(10):4855–4867. doi:10.1093/nar/gkv375. PubMed PMID: 25897126; PubMed Central PMCID: PMC4446435.
- MacAlpine DM, Almouzni G. Chromatin and DNA replication. Cold Spring Harbor Perspect Biol. 2013 Aug 1;5(8):a010207. doi:10.1101/cshperspect.a010207. PubMed PMID: 23751185; PubMed Central PMCID: PMC3721285.
- Petruk S, Cai J, Sussman R, et al. Delayed accumulation of H3K27me3 on Nascent DNA Is essential for recruitment of transcription factors at early stages of stem cell differentiation. Mol Cell. 2017 Apr 20;66(2):247–257 e5. doi:10.1016/j.molcel.2017.03.006. PubMed PMID: 28410996; PubMed Central PMCID: PMC5412717.
- Petruk S, Mariani SA, De Dominici M, et al. Structure of nascent chromatin is essential for hematopoietic lineage specification. Cell Rep. 2017 Apr 11;19(2):295–306. doi:10.1016/j.celrep.2017.03.035. PubMed PMID: 28402853; PubMed Central PMCID: PMC5408750.
- Chen HC, Martinez JP, Zorita E, et al. Position effects influence HIV latency reversal. Nat Struct Mol Biol. 2017 Jan;24(1):47–54. doi:10.1038/nsmb.3328. PubMed PMID: 27870832.
- Skene PJ, Henikoff S. Histone variants in pluripotency and disease. Development. 2013 Jun;140(12):2513–2524. doi:10.1242/dev.091439. PubMed PMID: 23715545.
- Henikoff S, Smith MM. Histone variants and epigenetics. Cold Spring Harbor Perspect Biol. 2015 Jan 5;7(1):a019364. doi:10.1101/cshperspect.a019364. PubMed PMID: 25561719; PubMed Central PMCID: PMC4292162.
- Szenker E, Ray-Gallet D, Almouzni G. The double face of the histone variant H3.3. Cell Res. 2011 Mar;21(3):421–434. doi:10.1038/cr.2011.14. PubMed PMID: 21263457; PubMed Central PMCID: PMC3193428.
- Bayona-Feliu A, Casas-Lamesa A, Reina O, et al. Linker histone H1 prevents R-loop accumulation and genome instability in heterochromatin. Nat Commun. 2017 Aug 18;8(1):283. doi:10.1038/s41467-017-00338-5. PubMed PMID: 28819201; PubMed Central PMCID: PMC5561251.
- Izquierdo-Bouldstridge A, Bustillos A, Bonet-Costa C, et al. Histone H1 depletion triggers an interferon response in cancer cells via activation of heterochromatic repeats. Nucleic Acids Res. 2017 Nov 16;45(20):11622–11642. doi:10.1093/nar/gkx746. PubMed PMID: 28977426.
- Millan-Arino L, Islam AB, Izquierdo-Bouldstridge A, et al. Mapping of six somatic linker histone H1 variants in human breast cancer cells uncovers specific features of H1.2. Nucleic Acids Res. 2014 Apr;42(7):4474–4493. doi:10.1093/nar/gku079. PubMed PMID: 24476918; PubMed Central PMCID: PMC3985652.
- Millan-Arino L, Izquierdo-Bouldstridge A, Jordan A. Specificities and genomic distribution of somatic mammalian histone H1 subtypes. Biochimica et biophysica acta. 2016 Mar;1859(3):510–519. doi:10.1016/j.bbagrm.2015.10.013. PubMed PMID: 26477490.
- Perez-Montero S, Carbonell A, Azorin F. Germline-specific H1 variants: the “sexy” linker histones. Chromosoma. 2016 Mar;125(1):1–13. doi:10.1007/s00412-015-0517-x. PubMed PMID: 25921218.
- Perez-Montero S, Carbonell A, Moran T, et al. The embryonic linker histone H1 variant of Drosophila, dBigH1, regulates zygotic genome activation. Dev Cell. 2013 Sep 30;26(6):578–590. doi:10.1016/j.devcel.2013.08.011. PubMed PMID: 24055651.
- Sancho M, Diani E, Beato M, et al. Depletion of human histone H1 variants uncovers specific roles in gene expression and cell growth. PLoS Genet. 2008 Oct;4(10):e1000227. doi:10.1371/journal.pgen.1000227. PubMed PMID: 18927631; PubMed Central PMCID: PMC2563032.
- Li E, Zhang Y. DNA methylation in mammals. Cold Spring Harbor Perspect Biol. 2014 May 1;6(5):a019133. doi:10.1101/cshperspect.a019133. PubMed PMID: 24789823; PubMed Central PMCID: PMC3996472.
- Manzo M, Wirz J, Ambrosi C, et al. Isoform-specific localization of DNMT3A regulates DNA methylation fidelity at bivalent CpG islands. The EMBO J. 2017 Dec 1;36(23):3421–3434. doi:10.15252/embj.201797038. PubMed PMID: 29074627; PubMed Central PMCID: PMC5709737.
- Nagano T, Lubling Y, Varnai C, et al. Cell-cycle dynamics of chromosomal organization at single-cell resolution. Nature. 2017 Jul 5;547(7661):61–67. doi:10.1038/nature23001. PubMed PMID: 28682332; PubMed Central PMCID: PMC5567812.
- Mercer TR, Dinger ME, Mattick JS. Long non-coding RNAs: insights into functions. Nat Rev Genet. 2009 Mar;10(3):155–159. doi:10.1038/nrg2521. PubMed PMID: 19188922.
- Mondal T, Subhash S, Vaid R, et al. MEG3 long noncoding RNA regulates the TGF-beta pathway genes through formation of RNA-DNA triplex structures. Nat Commun. 2015 Jul 24;6:7743. doi:10.1038/ncomms8743. PubMed PMID: 26205790; PubMed Central PMCID: PMC4525211.
- Postepska-Igielska A, Giwojna A, Gasri-Plotnitsky L, et al. LncRNA Khps1 regulates expression of the proto-oncogene SPHK1 via Triplex-mediated changes in chromatin structure. Mol Cell. 2015 Nov 19;60(4):626–636. doi:10.1016/j.molcel.2015.10.001. PubMed PMID: 26590717.
- O'Leary VB, Ovsepian SV, Carrascosa LG, et al. PARTICLE, a Triplex-forming long ncRNA, Regulates locus-specific methylation in response to low-dose irradiation. Cell Rep. 2015 Apr 21;11(3):474–485. doi:10.1016/j.celrep.2015.03.043. PubMed PMID: 25900080.
- Buscaino A, Allshire R, Pidoux A. Building centromeres: home sweet home or a nomadic existence? Curr Opin Genet Dev. 2010 Apr;20(2):118–126. doi:10.1016/j.gde.2010.01.006. PubMed PMID: 20206496.
- Catania S, Pidoux AL, Allshire RC. Sequence features and transcriptional stalling within centromere DNA promote establishment of CENP-A chromatin. PLoS Genet. 2015 Mar;11(3):e1004986. doi:10.1371/journal.pgen.1004986. PubMed PMID: 25738810; PubMed Central PMCID: PMC4349457.
- Folco HD, Pidoux AL, Urano T, et al. Heterochromatin and RNAi are required to establish CENP-A chromatin at centromeres. Science. 2008 Jan 04;319(5859):94–97. doi:10.1126/science.1150944. PubMed PMID: 18174443; PubMed Central PMCID: PMC2586718.
- Kagansky A, Folco HD, Almeida R, et al. Synthetic heterochromatin bypasses RNAi and centromeric repeats to establish functional centromeres. Science. 2009 Jun 26;324(5935):1716–1719. doi:10.1126/science.1172026. PubMed PMID: 19556509; PubMed Central PMCID: PMC2949999.
- Luk E, Ranjan A, Fitzgerald PC, et al. Stepwise histone replacement by SWR1 requires dual activation with histone H2A.Z and canonical nucleosome. Cell. 2010 Nov 24;143(5):725–736. doi:10.1016/j.cell.2010.10.019. PubMed PMID: 21111233.
- Ranjan A, Mizuguchi G, FitzGerald PC, et al. Nucleosome-free region dominates histone acetylation in targeting SWR1 to promoters for H2A.Z replacement. Cell. 2013 Sep 12;154(6):1232–1245. doi:10.1016/j.cell.2013.08.005. PubMed PMID: 24034247; PubMed Central PMCID: PMC3815578.
- Liang X, Shan S, Pan L, et al. Structural basis of H2A.Z recognition by SRCAP chromatin-remodeling subunit YL1. Nat Struct Mol Biol. 2016 Apr;23(4):317–323. doi:10.1038/nsmb.3190. PubMed PMID: 26974124.
- Ranjan A, Wang F, Mizuguchi G, et al. H2A histone-fold and DNA elements in nucleosome activate SWR1-mediated H2A.Z replacement in budding yeast. eLife. 2015 Jun 27;4:e06845. doi:10.7554/eLife.06845. PubMed PMID: 26116819; PubMed Central PMCID: PMC4508883.
- Buschbeck M, Hake SB. Variants of core histones and their roles in cell fate decisions, development and cancer. Nat Rev Mol Cell Biol. 2017 May;18(5):299–314. doi:10.1038/nrm.2016.166. PubMed PMID: 28144029.
- Schlesinger S, Kaffe B, Melcer S, et al. A hyperdynamic H3.3 nucleosome marks promoter regions in pluripotent embryonic stem cells. Nucleic Acids Res. 2017 Dec 1;45(21):12181–12194. doi:10.1093/nar/gkx817. PubMed PMID: 29036702; PubMed Central PMCID: PMC5716099.
- Torres CM, Biran A, Burney MJ, et al. The linker histone H1.0 generates epigenetic and functional intratumor heterogeneity. Science. 2016 Sep 30;353(6307). pii: aaf1644. doi:10.1126/science.aaf1644. PubMed PMID: 27708074; PubMed Central PMCID: PMC5131846.
- Carbonell A, Pérez-Montero S, Climent-Cantó P, et al. The Germline linker histone dBigH1 and the translational regulator bam form a repressor loop essential for male germ stem cell differentiation. Cell Rep. 2017;21(11):3178–3189. doi:10.1016/j.celrep.2017.11.060. PubMed PMID: 29241545.
- Aguilera A, Gomez-Gonzalez B. Genome instability: a mechanistic view of its causes and consequences. Nat Rev Genet. 2008 Mar;9(3):204–217. doi:10.1038/nrg2268. PubMed PMID: 18227811.
- Vijg J, Suh Y. Genome instability and aging. Annu Rev Physiol. 2013;75:645–668. doi:10.1146/annurev-physiol-030212-183715. PubMed PMID: 23398157.
- Negrini S, Gorgoulis VG, Halazonetis TD. Genomic instability–an evolving hallmark of cancer. Nat Rev Mol Cell Biol. 2010 Mar;11(3):220–228. doi:10.1038/nrm2858. PubMed PMID: 20177397.
- Nair N, Shoaib M, Sorensen CS. Chromatin dynamics in genome stability: Roles in suppressing endogenous DNA Damage and facilitating DNA repair. Int J Mol Sci. 2017 Jul 10;18(7). pii: E1486. doi:10.3390/ijms18071486. PubMed PMID: 28698521; PubMed Central PMCID: PMC5535976.
- Padeken J, Zeller P, Gasser SM. Repeat DNA in genome organization and stability. Curr Opin Genet Dev. 2015 Apr;31:12–19. doi:10.1016/j.gde.2015.03.009. PubMed PMID: 25917896.
- Carone DM, Lawrence JB. Heterochromatin instability in cancer: from the Barr body to satellites and the nuclear periphery. Semin Cancer Biol. 2013 Apr;23(2):99–108. doi:10.1016/j.semcancer.2012.06.008. PubMed PMID: 22722067; PubMed Central PMCID: PMC3500402.
- Zeller P, Padeken J, van Schendel R, et al. Histone H3K9 methylation is dispensable for Caenorhabditis elegans development but suppresses RNA:DNA hybrid-associated repeat instability. Nature Genet. 2016 Nov;48(11):1385–1395. doi:10.1038/ng.3672. PubMed PMID: 27668659.
- Pan MR, Hsieh HJ, Dai H, et al. Chromodomain helicase DNA-binding protein 4 (CHD4) regulates homologous recombination DNA repair, and its deficiency sensitizes cells to poly(ADP-ribose) polymerase (PARP) inhibitor treatment. J Biol Chem. 2012 Feb 24;287(9):6764–6772. doi:10.1074/jbc.M111.287037. PubMed PMID: 22219182; PubMed Central PMCID: PMC3307306.
- Gong F, Chiu LY, Cox B, et al. Screen identifies bromodomain protein ZMYND8 in chromatin recognition of transcription-associated DNA damage that promotes homologous recombination. Genes Dev. 2015 Jan 15;29(2):197–211. doi:10.1101/gad.252189.114. PubMed PMID: 25593309; PubMed Central PMCID: PMC4298138.
- Gong F, Clouaire T, Aguirrebengoa M, et al. Histone demethylase KDM5A regulates the ZMYND8-NuRD chromatin remodeler to promote DNA repair. J Cell Biol. 2017 Jul 03;216(7):1959–1974. doi:10.1083/jcb.201611135. PubMed PMID: 28572115; PubMed Central PMCID: PMC5496618.
- Esteller M. Epigenetics in cancer. New England J Med. 2008 Mar 13;358(11):1148–1159. doi:10.1056/NEJMra072067. PubMed PMID: 18337604.
- Avery OT, Macleod CM, McCarty M. Studies on the chemical nature of the substance inducing transformation of pneumococcal types: Induction of transformation by a desoxyribonucleic acid fraction isolated from Pneumococcus type III. Oswald Theodore Avery (1877-1955). Clin Orthop Relat Res. 2000 Oct;(379 Suppl):S3–S8. PubMed PMID: 11039746.
- Baylin SB, Ohm JE. Epigenetic gene silencing in cancer – a mechanism for early oncogenic pathway addiction? Nat Rev Cancer. 2006 Feb;6(2):107–116. doi:10.1038/nrc1799. PubMed PMID: 16491070.
- Ehrlich M. DNA hypomethylation in cancer cells. Epigenomics. 2009 Dec;1(2):239–259. doi:10.2217/epi.09.33. PubMed PMID: 20495664; PubMed Central PMCID: PMC2873040.
- Beguelin W, Popovic R, Teater M, et al. EZH2 is required for germinal center formation and somatic EZH2 mutations promote lymphoid transformation. Cancer Cell. 2013 May 13;23(5):677–692. doi:10.1016/j.ccr.2013.04.011. PubMed PMID: 23680150; PubMed Central PMCID: PMC3681809.
- Beguelin W, Teater M, Gearhart MD, et al. EZH2 and BCL6 Cooperate to Assemble CBX8-BCOR complex to repress bivalent promoters, mediate germinal center formation and lymphomagenesis. Cancer Cell. 2016 Aug 8;30(2):197–213. doi:10.1016/j.ccell.2016.07.006. PubMed PMID: 27505670; PubMed Central PMCID: PMC5000552.
- Beguelin W, Rivas MA, Calvo Fernandez MT, et al. EZH2 enables germinal centre formation through epigenetic silencing of CDKN1A and an Rb-E2F1 feedback loop. Nat Commun. 2017 Oct 12;8(1):877. doi:10.1038/s41467-017-01029-x. PubMed PMID: 29026085; PubMed Central PMCID: PMC5638898.
- Richmond TD, Chohan M, Barber DL. Turning cells red: signal transduction mediated by erythropoietin. Trends Cell Biol. 2005 Mar;15(3):146–155. doi:10.1016/j.tcb.2005.01.007. PubMed PMID: 15752978.
- Schnoder TM, Arreba-Tutusaus P, Griehl I, et al. Epo-induced erythroid maturation is dependent on Plcgamma1 signaling. Cell Death Differ. 2015 Jun;22(6):974–985. doi:10.1038/cdd.2014.186. PubMed PMID: 25394487; PubMed Central PMCID: PMC4423181.
- Bheda P, Schneider R. Epigenetics reloaded: the single-cell revolution. Trends Cell Biol. 2014 Nov;24(11):712–723. doi:10.1016/j.tcb.2014.08.010. PubMed PMID: 25283892.
- Magnani L, Frige G, Gadaleta RM, et al. Acquired CYP19A1 amplification is an early specific mechanism of aromatase inhibitor resistance in ERalpha metastatic breast cancer. Nat Genet. 2017 Mar;49(3):444–450. doi:10.1038/ng.3773. PubMed PMID: 28112739; PubMed Central PMCID: PMC5326683.
- Moran S, Martinez-Cardus A, Sayols S, et al. Epigenetic profiling to classify cancer of unknown primary: a multicentre, retrospective analysis. Lancet Oncol. 2016 Oct;17(10):1386–1395. doi:10.1016/S1470-2045(16)30297-2. PubMed PMID: 27575023.
- Rodriguez-Paredes M, Esteller M. Cancer epigenetics reaches mainstream oncology. Nat Med. 2011 Mar;17(3):330–339. doi:10.1038/nm.2305. PubMed PMID: 21386836.
- Perez-Salvia M, Simo-Riudalbas L, Llinas-Arias P, et al. Bromodomain inhibition shows antitumoral activity in mice and human luminal breast cancer. Oncotarget. 2017 Aug 1;8(31):51621–51629. doi:10.18632/oncotarget.18255. PubMed PMID: 28881673; PubMed Central PMCID: PMC5584274.
- Iorio F, Knijnenburg TA, Vis DJ, et al. A Landscape of pharmacogenomic interactions in Cancer. Cell. 2016 Jul 28;166(3):740–754. doi:10.1016/j.cell.2016.06.017. PubMed PMID: 27397505; PubMed Central PMCID: PMC4967469.
- Llinas-Arias P, Esteller M. Epigenetic inactivation of tumour suppressor coding and non-coding genes in human cancer: an update. Open Biol. 2017 Sep;7(9). pii: 170152. doi:10.1098/rsob.170152. PubMed PMID: 28931650; PubMed Central PMCID: PMC5627056.