ABSTRACT
Maternal smoking during pregnancy may affect newborn DNA methylation (DNAm). However, little is known about how these associations vary by a newborn’s sex and/or maternal nutrition. To fill in this research gap, we investigated epigenome-wide DNAm associations with maternal smoking during pregnancy in African American mother-newborn pairs. DNAm profiling in cord (n = 379) and maternal blood (n = 300) were performed using the Illumina HumanMethylation450 BeadChip array. We identified 12 CpG sites whose DNAm levels in cord blood were associated with maternal smoking, at a false discovery rate <5%. The identified associations in the GFI1 gene were more pronounced in male newborns than in females (P = 0.002 for maternal smoking × sex interaction at cg18146737). We further observed that maternal smoking and folate level may interactively affect cord blood DNAm level at cg05575921 in the AHRR gene (P = 5.0 × 10−4 for interaction): compared to newborns unexposed to maternal smoking and with a high maternal folate level (>19.2 nmol/L), the DNAm level was about 0.03 lower (P = 3.6 × 10−4) in exposed newborns with a high maternal folate level, but was 0.08 lower (P = 1.2 × 10−8) in exposed newborns with a low maternal folate level. Our data suggest that adequate maternal folate levels may partly counteract the impact of maternal smoking on DNAm. These findings may open new avenues of inquiry regarding sex differences in response to environmental insults and novel strategies to mitigate their intergenerational health effects through optimization of maternal nutrition.
Introduction
Early-life adverse environmental exposures, including maternal smoking during pregnancy, have shown long-lasting impacts on the development of complex human diseases in later life, such as preterm birth [Citation1], low birthweight [Citation2], obesity [Citation3], asthma [Citation4,Citation5], and neurodevelopmental disorders [Citation6–Citation8]. Despite significant adverse effects, the rate of maternal smoking remains high, and about 12% of newborns in the US are exposed to maternal smoking during pregnancy [Citation9]. Thus, understanding potential mechanisms by which maternal smoking during pregnancy affects child health and identifying safe and effective interventions will have significant public health implications.
There is increasing evidence that maternal smoking may affect a child’s global and gene-specific DNA methylation (DNAm) profiles as early as in utero [Citation10–Citation21]. An increasing number of genes have been identified for which the DNAm levels at specific CpG sites in newborns were significantly associated with maternal smoking during pregnancy, some of which – such as those encoding Aryl-hydrocarbon receptors repressor (AHRR), growth factor independent 1 transcriptional repressor (GFI1), and cytochrome P450, family 1, member A1 (CYP1A1) – have been reported by multiple studies [Citation10–Citation19,Citation21]. Such maternal smoking-related methylation changes may persist throughout childhood [Citation22,Citation23], adolescence [Citation12], and even adulthood, which may be independent of active smoking in the offspring[Citation24]. These identified methylation changes not only may serve as biomarkers that reflect past exposures to maternal smoking during pregnancy [Citation25] but also at least partially mediate the adverse effects of maternal smoking on child health, such as low birthweight [Citation14] and asthma (or chronic lung disease) [Citation26].
Our study sought to address several important gaps in the field. To our knowledge, the relevant available genome-wide scale reports have predominantly focused on white populations [Citation11–Citation16,Citation18,Citation19], with a few focused on multi-ethnic populations [Citation10,Citation23,Citation24] and Asian populations [Citation21]. It is still less clear whether these findings can be extended to other minority populations. Sex differences exist and are reflected in DNAm levels in certain gene regions [Citation13,Citation27], as well as in susceptibility to childhood diseases that are associated with maternal smoking during pregnancy [Citation28,Citation29]. It is possible that there are some sex-specific DNAm markers for maternal smoking that may account for sex-specific susceptibility to childhood diseases. However, only a limited number of studies have been conducted to explore sex-specific DNAm changes in response to maternal smoking, with inconsistent findings [Citation13,Citation16,Citation27,Citation30]. As an example, Murphy et al. reported that maternal smoking during pregnancy significantly altered methylation levels of the differentially methylated region (DMR) in the insulin-like growth factor 2 (IGF2) gene, which was more pronounced in male offspring [Citation27]. In comparison, Bouwland-Both et al. demonstrated that associations between maternal smoking and DNAm in the IGF2 DMR were more profound among newborn girls than boys [Citation16].
Although smoking is a preventable environmental exposure, approximately 50% of all smokers will continue smoking after becoming pregnant [Citation31]. Given that a high proportion of smokers are unable to quit during pregnancy even with cessation counseling, it is critical to identify modifiable factors that may mitigate the adverse effects of maternal smoking on child health. A recent study suggested that nutritional factors may partly modify the influence of maternal smoking during pregnancy on the newborn epigenome [Citation32]. Folate is critical to fetal growth and development because it is an important component in the one-carbon metabolism pathway and provides methyl groups for a range of biochemical reactions, including DNAm [Citation33]. Although periconceptional folic acid supplementation and food fortification are recommended in the US, both NHANES data [Citation34] and our own data [Citation35] have showed considerable variation in folate levels among pregnant women. Furthermore, according to the Centers for Disease Control (CDC), about a quarter of women have insufficient folate levels during pregnancy [Citation36] to prevent neural tube defects. Although there is increasing evidence that maternal smoking and maternal folate level [Citation37,Citation38], respectively, can affect the developing epigenome, few studies have evaluated how these two factors jointly affect the newborn epigenome.
To fill these aforementioned research gaps, we studied the epigenome-wide DNAm associations with maternal smoking during pregnancy (referred as ‘maternal smoking’ in this study, as defined in the Methods) in African American mother-newborn pairs from the Boston Birth Cohort (BBC), using DNAm measured in both cord and maternal blood collected at delivery. Sex stratified analyses and tests for interactions were performed to identify sex-specific DNAm changes in response to maternal smoking. We further studied whether maternal folate levels could alleviate the effects of maternal smoking on the newborn epigenome.
Results
Population characteristics
The current study included 39 newborns exposed to maternal smoking and 300 unexposed newborns after data quality control steps. Compared with unexposed newborns, exposed newborns were more likely to experience high levels of maternal lifetime stress (P = 0.011), had less-educated mothers (P = 0.010), had shorter gestational length (P = 0.044), and had lower birthweight (P = 0.005). Other factors, including maternal age at delivery, pre-pregnancy body mass index (BMI), household annual income, alcohol drinking during pregnancy, and newborn sex, were comparable between the two groups (all P > 0.05, ).
Table 1. Characteristics of 339 African American mother-infant pairs from the Boston Birth Cohort.
Epigenome-wide association study in newborns
At a false discovery rate (FDR) <5%, we identified 12 CpG sites at the auto-chromosome, including 7 in the GFI1 gene, 4 in the AHRR gene and 1 in the Prion Protein (PRNP) gene, with significantly altered methylation levels in newborns exposed to maternal smoking compared to unexposed newborns (Supplementary Fig. S1, and ). Nine out of the 12 CpG sites (7 in the GFI gene and 2 in the AHRR gene) were hypomethylated and the other 3 were hypermethylated (2 in the AHRR gene and 1 in the PRNP gene) in the newborns exposed to maternal smoking, with the magnitude of DNAm difference between the exposed and unexposed newborns ranging from 0.01 to 0.09 ( and Supplementary Fig S2). Our sensitivity analyses further showed that the identified associations at the 12 CpG sites remained largely unchanged after removing very preterm newborns or removing all preterm newborns (Supplementary Table S1).
Table 2. Genome-wide DNA methylation associations with maternal smoking during pregnancy in 339 newborns and in 252 mothers from the Boston Birth Cohort.
Maternal-newborn DNAm correlation
For the 12 significant CpG sites identified in this study, we further tested whether such maternal smoking-associated DNAm changes in the newborns also existed in the maternal blood. As shown in and Supplementary Fig S2, maternal DNAm of 7 CpG sites in the GFI1 gene and of 4 CpG sites in the AHRR gene were all significantly associated with smoking during pregnancy, with the same direction of associations that we observed in cord blood. We also observed that there were significant mother-newborn DNAm correlations at these sites, with correlation coefficients varying from 0.17 to 0.59, which was significantly higher than the average correlations for all CpG sites (correlation coefficient: 0.11; standard deviation: 0.14) analyzed in this study (Supplementary Fig S3). The correlation coefficients at these 12 CpG sites may vary by maternal smoking status, since they were higher at some sites (cg05575921 and cg21161138) but lower at other sites (cg09662411 and cg10399789) in 12 mother-infant pairs exposed to maternal smoking compared to 77 unexposed pairs (Supplementary Table S2).
Sex-specific DNAm associations with maternal smoking
In the sex-stratified epigenome-wide association tests, 5 CpG sites (4 in the GFI1 gene and 1 in the AHRR gene) were significantly associated with maternal smoking at an FDR <5% in 193 male newborns (22 exposed vs. 171 unexposed), all of which overlapped with the significant CpG sites that we identified in the total sample. No genome-wide significant associations were found in 146 female newborns ().
Figure 1. Mirror-Manhattan plot for sex-specific epigenome-wide associations of cord blood DNA methylation levels with maternal smoking during pregnancy. The upper panel is the plot in males and the lower panel is the plot in females. The gray line represents the epigenome-wide significance threshold with false discovery rate (FDR) = 5% and the gray dotted line represents the epigenome-wide significance threshold after Bonferroni correction.
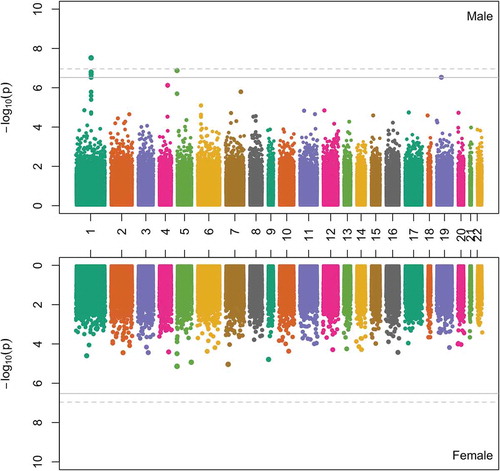
When focusing on the 12 top CpG sites identified in the total sample, we observed that, at the 7 CpG sites in the GFI1 gene, there were significant maternal smoking-DNAm associations in males but not in females (). The maternal smoking × sex interaction effects were FDR-significant or marginally significant at the 5 CpG sites in the GFI1 gene, with cg18146737 (P = 0.003 for interaction, FDR = 0.04) as the top CpG site. In comparison, the associations between maternal smoking and DNAm at the other 5 CpG sites (in the AHRR and PRNP genes) were comparable in males and in females, with no significant interactions by sex ().
Table 3. Sex-specific associations between maternal smoking during pregnancy and cord blood DNA methylation level from the Boston Birth Cohort.
Maternal folate level, maternal smoking, and cord blood dnam level
There were 289 newborns with available data on maternal folate levels, whose population characteristics were comparable to that of the total 339 newborns (Supplementary Table S3). In these 289 newborns, maternal folate level itself showed no associations with DNAm levels at the 12 top CpG sites identified in the total sample (data not shown). We then stratified all the newborns into four subgroups based on maternal smoking status (exposed vs. unexposed) and maternal folate levels (classified as ‘low’ if folate within the 1st quartile or ≤19.2 nmol/L; compared to ‘high’ if folate in the 2nd–4th quartiles or >19.2 nmol/L, as detailed in the Methods) to explore the combined associations between maternal smoking and maternal folate level. Using the unexposed newborns with high maternal folate levels as the reference group (n = 192), we found that DNAm level at cg05575921 was comparable in the unexposed newborns with low maternal folate level (n = 67), or about 0.03 lower (P = 3.6 × 10−4) in the exposed newborns with high maternal folate level (n = 21). In comparison, DNAm level at this site was the lowest in the exposed newborns with low maternal folate level (n = 9), which was about 0.08 lower (P = 1.2 × 10−8) than the reference group. A significant maternal smoking × folate interaction was further observed at this CpG site (P = 2.1 × 10−3, FDR = 0.03, ). Similarly, at the 7 CpG sites in the GFI1 gene, the effect sizes for the maternal smoking-DNAm associations were about 2 times higher in newborns with low maternal folate levels than in their counterparts with high maternal folate levels, although the maternal smoking × folate interactions were statistically insignificant ( and ). Similar trends were also observed when the analyses were limited to only males (given that the associations between maternal smoking and GFI1 DNAm were significant in males but not in females as shown in ) ( and Supplementary Table S4).
Table 4. Joint and interactive associations between maternal smoking exposure and maternal plasma folate level on cord blood DNA methylation levels at the top 12 CpG sites.
Additional analyses on the reported candidate CpGs
The recent meta-analysis by Joubert et al. [Citation10] reported 3,932 CpG sites for maternal smoking after cell type adjustment, which included the 11 CpG sites in the GFI1 and AHRR genes as identified in our sample, while 3,853 of the other reported sites were also available in our sample. When focusing on these reported sites, we found that 643 sites tended to be associated with maternal smoking in our sample at a nominal P < 0.05. About 67 CpG sites (located in 39 genes such as MYO1G, CYP1A1, NKAPL, and ZBTB38, Supplementary Table S5) remained to be significantly associated with maternal smoking at FDR <0.05 (after adjusting for 3,864 tests), with the same direction of associations as reported by Joubert et al.
In the sex-stratified analyses, we found that another three CpG sites, located in the KDM5B, RASGEF1A, and TIGD7 genes, were significantly associated with maternal smoking in males (FDR <0.05). However, no such associations were identified in females. The maternal smoking × sex interactions at these three sites (at a nominal P = 0.004–0.02 for interaction), and at the other 67 CpG sites that were validated in this study were no longer significant after FDR correction (Supplementary Table S5). In subgroup analyses stratified by both maternal smoking status and maternal folate levels (Supplementary Table S6), we observed that the effect size of maternal smoking on DNAm at some sites (such as cg15073784, cg12803068, and cg18892212) tended to be higher in newborns with low maternal folate levels than in their counterparts with high maternal folate levels; however, no significant interactions were identified between maternal smoking and maternal folate levels.
Discussion
In this epigenome-wide association study of US African American mother-newborn pairs, we demonstrated that 12 CpG sites, located in the GFI1 (7 sites), AHRR (4 sites), and PRNP (1 site) genes, were differentially methylated in the cord blood of newborns exposed to maternal smoking compared to unexposed newborns. Similar smoking-related changes in the AHRR and GFI1 genes were also noticed in maternal whole blood. We further identified that the maternal smoking-related DNAm changes in the GFI1 gene, but not in the AHRR gene, were sex dependent. These changes in the GFI1 gene were more pronounced in boys than in girls, suggesting that a sex-specific mechanism may exist in relation to methylation changes of some genes due to adverse in utero exposures. Furthermore, we found that a higher maternal folate level may partly attenuate the adverse effects of maternal smoking on the newborn DNAm profile. Taking these findings together, this study not only confirmed previous findings about the significant impact of maternal smoking on the newborn epigenome, but it also suggested that the male fetus may be more vulnerable to some maternal smoking-related DNAm alternations than the female fetus, and that an adequate maternal folate level may partly counteract such maternal smoking-related DNAm changes.
Our study, among African Americans, confirmed that maternal smoking was genome-wide significantly associated with cord blood DNAm changes in the GFI1 and AHRR genes, which is consistent with findings from other studies in white populations [Citation11–Citation13,Citation16] or in studies with mixed ethnicities [Citation10,Citation23,Citation24]. A recent meta-analysis by Joubert et al. identified thousands of CpG sites that were differentially methylated in cord blood in relation to maternal smoking [Citation10], most of which had a small effect size (i.e. <5% DNAm change). We successfully replicated almost all of those CpG sites with reported effect sizes ≥5% DNAm change, and 67 sites with reported effect sizes <5% DNAm change. These replicated CpG sites included those that have been reported by multiple studies (such as CpG sites in the MYO1G, CYP1A1, and FRMD4A genes) [Citation10–Citation14,Citation21,Citation23]. Such findings by our group and others suggest that smoking-related DNAm changes in cord blood are shared by different ethnic populations. We also identified a novel maternal smoking-related CpG site located on the PRNP gene. This gene provides instructions for making a protein called prion protein (PrP), which is active in the brain and several other tissues [Citation39–Citation41]. There are no previous studies reporting the relationships between maternal smoking and DNAm in the PRNP gene. Given a limited sample size in this study, we could not exclude the possibility that our finding in the PRNP gene may be spurious. Another possibility is that the identified association in the PRNP gene may be specific to African Americans. Thus, further replication is needed in other populations.
For the genome-wide significant CpG sites in the GFI1 and AHRR genes identified in this study, we observed that their DNAm levels in maternal blood (collected at delivery) were also significantly altered in smoking mothers compared to non-smokers, with the same association directions as we observed in cord blood. The mother-newborn DNAm correlations at these CpG sites were all stronger than the average correlation level for all of the studied epigenome-wide CpG sites. The correlation coefficients at these sites may vary by maternal smoking status, which indicates that maternal smoking may affect inherited methylation patterns. However, due to a limited sample size (n = 89 pairs) and due to inconsistent findings for different CpG sites in the same gene, our findings regarding the influence of maternal smoking on the maternal-newborn correlations require further validation.
To date, it remains largely unknown whether the adverse effect of maternal smoking on the newborn epigenome differs by newborn sex. Richmond et al. [Citation13] showed that the smoking-associated methylation change at the AHRR gene (cg05575921) was larger in girls than in boys, while the methylation change at CYP1A1 (cg05549655) was larger in boys than in girls, with significant interactions with sex at both sites. However, they did not find consistent sex-specific associations for the other CpG sites in the same gene regions, providing no strong evidence for sex-specific associations. In comparison, Novakovic et al. [Citation30] found no significant sex difference in the associations between DNAm of the AHRR gene and maternal smoking. Our study in this African American population also showed no significant sex difference in associations between maternal smoking and DNAm level in the AHRR gene or in the PRNP gene. However, we demonstrated that the smoking-related methylation changes in the GFI1 gene were all more pronounced in male newborns than in females, with significant maternal smoking × sex interactions effects at these sites. Our data also indicate that sex-specific associations may exist at some other previously reported methylation sites, as shown in Supplementary Table S5. Taken together, our data suggest that males and females may react differently at certain sites (but not all) when exposed to maternal smoking, and that the offspring’s sex should be an included variable and its distribution within the study population should be considered in future DNAm studies. These findings, if confirmed, may open up new inquiries regarding sex differences in response to environmental insults. It will also be interesting to further explore whether these sex-specific methylation markers for maternal smoking can partly explain the sex-specific susceptibility to some childhood diseases.
Smoking status and folate level in mothers are two strong biological candidates that may impact the developing epigenome [Citation42], yet few studies have evaluated the extent to which DNAm in the offspring is modified by these two exposures jointly. Our study indicated that maternal folate level, a critical factor in the one-carbon-cycle and donor for the methyl-group, may attenuate the adverse effects of maternal smoking on the newborn epigenome, with a significant maternal smoking × folate interaction on DNAm levels at cg05575921 in the AHRR gene. Similarly, a study in young adults showed that methylenetetrahydrofolate reductase (MTHFR) gene methylation level may moderate the impact of active smoking on DNAm at AHRR in African Americans, which also suggests that individual differences in the one-carbon-cycle plays a role in modifying the adverse effects of smoking on the epigenome [Citation43]. Although the underlying mechanism is still unclear, these identified interaction associations are biologically plausible and have been tested in animal studies. There is a mouse study that demonstrated that the mutation of folate metabolism could modify epigenetic instability and transgenerational effects in development [Citation44]. Evidence from another mouse study suggested that maternal dietary supplementation with folic acid may counteract the DNA hypomethylating effect of prenatal bisphenol exposure on the offspring’s epigenome [Citation45,Citation46]. We further propose that a higher level of folate, a key source of the one carbon group used to methylate DNA, may lead to better maintenance of methylation levels in newborns and thus partly counteract the DNA hypomethylating effects of maternal smoking. These findings, if they can be further validated in a larger study population, will have significant clinical implications: they may indicate that mothers who fail to quit smoking can still partly alleviate the adverse effects of maternal smoking on their newborns by taking sufficient folate during pregnancy.
This study had several limitations. First, the BBC is a cohort enriched by low birthweight and preterm births, and thus caution is needed when generalizing our findings to populations with different characteristics. To ensure that our study findings were robust, we performed sensitivity analyses and demonstrated that our findings remained unchanged when limiting to term newborns. Second, information about maternal smoking was self-reported by the mothers via questionnaire and there was no data about maternal cotinine levels. We also lacked data on maternal secondhand smoke exposure during pregnancy. Third, DNAm levels at the identified sites were not validated using other technology such as pyrosequencing, and no functional study was conducted. However, most of our findings were consistent with previous research except for one novel gene identified in this study. Fourth, questions remain regarding how to interpret small DNAm differences, mostly those <5%. It is possible that the small changes that we identified in cord blood may reflect larger changes in a specific cell type. Fifth, the sample size of this study was relatively small, and thus, may have been underpowered to identify novel CpG sites with modest effects. Our findings, especially those based on subgroup analyses and interaction tests, need further validation in another larger independent population, and/or via meta-analyses of multiple other datasets. Finally, DNAm is one of several epigenetic mechanisms that work in conjunction to regulate gene expression, and as such, further work is needed to explore other epigenetic mechanisms, such as histone modifications in the presence of maternal smoking.
In summary, we confirmed significant epigenome-wide associations between maternal smoking and cord blood DNAm at multiple sites (including the GFI1 and AHRR genes) in African American mother-infant pairs, which are consistent with other published findings in white populations, suggesting that these smoking-related DNAm changes are shared across both whites and African Americans. Furthermore, we demonstrated that the adverse effect of maternal smoking on the newborn epigenome may be sex dependent at certain genes and may be partly mitigated by a sufficient maternal folate level. Future studies are needed to explore whether the sex-specific DNAm markers for maternal smoking may explain the sex-specific susceptibility of smoking-related child health conditions, and whether a sufficient maternal folate level could counteract the adverse effects of maternal smoking on child health. If confirmed, such findings will greatly contribute to our understanding of how maternal smoking during pregnancy affects child health and sex differences, and provide targets for the prevention and treatment of smoking-related childhood diseases.
Methods
The boston birth cohort (BBC) and the study population
This study included 379 African American newborns and 300 African American mothers from the Boston Birth Cohort (BBC). The parent study cohort was initiated in 1998 with a rolling enrollment in Boston, MA, as detailed elsewhere [Citation2]. Briefly, we invited those mothers who delivered singleton live preterm or low birthweight (<2,500 grams) newborns, or mothers who delivered term and normal birthweight newborns to join the cohort. Pregnancies that were a result of in vitro fertilization, multiple gestations (e.g., twins, triplets), fetal chromosomal abnormalities or major birth defects were excluded from the parent study. After giving written informed consent, the enrolled participants completed a questionnaire to obtain information on maternal characteristics, lifestyle and diet. A maternal blood sample was obtained within 24–72 hours after delivery and a cord blood sample was obtained at delivery. The study protocol was approved by the Institutional Review Boards of Boston University Medical Center, the Ann & Robert H. Lurie Children’s Hospital of Chicago, and the Johns Hopkins Bloomberg School of Public Health.
Definition of maternal smoking and covariates
Maternal variables during pregnancy, such as maternal smoking during pregnancy, alcohol consumption during pregnancy and maternal psychosocial stress, were collected based on a standard maternal questionnaire, as has been reported previously [Citation47]. Specifically, for smoking status, the mothers were asked the following questions: ‘1) In the six months before you found out you were pregnant, did you smoke/use tobacco?’; 2) ‘Did you smoke/use tobacco in the first three months of pregnancy?’; 3) ‘Did you smoke/use tobacco in the middle three months of pregnancy?’; and 4) ‘Did you smoke/use tobacco in the last three months of pregnancy?’ We defined ‘maternal smoking during pregnancy’ (referred to as ‘maternal smoking’ in this study) as those mothers who answered ‘yes’ to question 1 and/or 2, as well as ‘yes’ to question 3 and/or 4; and defined ‘non-smoking’ mothers as those mothers who answered ‘no’ to all of the questions. Mothers who answered ‘yes’ to questions 1 and/or 2, and ‘no’ to question 3 and 4 were categorized as ‘quitters’ and were excluded from the study due to a limited number of mothers in this subgroup.
Measurement of maternal plasma folate level
Total folate levels in maternal plasma were measured at a commercial laboratory using chemiluminescent immunoassay on a MAGLUMI 2000 Analyzer (Snibe Co., Ltd, Shenzhen, China) [Citation35]. Rigorous quality control/quality assurance procedures were applied as reported previously [Citation35], and the inter-assay coefficient of variance was <4%.
Methylation measurements in cord blood from newborns and quality control steps
Genomic DNA, isolated from EDTA-treated peripheral white blood cells, were diluted to 50–100 ng/uL and shipped to the Center for Genetic Medicine, Northwestern University Feinberg School of Medicine for methylation profiling. About 0.5 μg of genomic DNA was first bisulfite-converted using the EZ-96 DNA Methylation™ Gold Kit, and DNAm levels at each site were then measured using the Illumina Infinium HumanMethylation450 BeadChip (450K), according to the manufacturer’s instructions. For each sample, a raw intensity file (.idat) was processed.
Using the R/Bioconductor package ‘minfi’, [Citation48] we performed several quality control steps as we reported previously [Citation49]. After examining the 450K control probes to assess bisulfite conversion, extension, hybridization, staining, and others, we plotted the median for both Meth and Unmeth intensity signals for each array to identify poorly performing arrays, and then checked for sex discrepancies by comparing self-reported sex against data-derived sex values. Ten samples were removed from further analyses because they were identified as outliers with a median log2 intensity value <10.5 and/or had sex inconsistency. At the locus level, we removed 670 loci that had a detection p-value (a measure of probe performance) >0.01 in more than 10% of the samples; 17,541 loci that had an annotated single nucleotide polymorphism (minor allele frequency >0.01 in African Americans) at the measured and neighboring locus (±1 bp depending on probe strand orientation) and/or were previously reported to be cross-reactive [Citation50]; and 11,648 loci on the X-chromosome or Y-chromosome. These filters resulted in high quality DNAm data for 455,737 sites among 369 samples for subsequent analyses. After removing 27 samples from newborns whose mothers quit smoking during pregnancy and 3 with missing data on maternal smoking, we focused our analyses on 339 cord blood samples with available maternal smoking information.
With the ‘minfi’ package [Citation48], we applied a stratified quantile normalization procedure to the raw methylation data, and obtained normalized beta values (β, ranging from 0–1 for 0 to 100% methylated) for each cord blood sample. To account for potential batch effects, β and corresponding M values (logit-transformed β) were ComBat-transformed [Citation51] using the ‘sva’ package [Citation52] with the array number as the surrogate for the batches. ComBat-transformed M values were utilized for downstream statistical analyses, since they were reported as being superior to β values for the identification of differential methylation [Citation53]. ComBat-transformed β values were used for plotting purposes since they are more intuitive.
Methylation measurements in maternal blood and quality control steps
The procedure for genome-wide methylation profiling, data cleaning, and processing in maternal blood samples, similar to that described above for cord blood samples, has been reported elsewhere [Citation49]. These procedures led to 290 maternal samples for subsequent analyses [Citation49]. For the current study, we removed 26 mothers who quit smoking during pregnancy and 12 with missing data on smoking during pregnancy, leaving the focus of our analyses on 252 maternal samples.
Cell composition estimation
Using the estimateCellCounts() function in the ‘minfi’ package, [Citation48] the distribution of each cell type, including CD4+, CD8 + T cells, B cells, monocytes, granulocytes, natural killer cells, and nucleated red blood cells (specific to cord blood), was inferred for each cord blood [Citation54–Citation56] and maternal sample [Citation54,Citation55], respectively, based on external cord blood and adult reference DNAm signatures of the constituent cell type from Illumina HumanMethylation450 BeadChips.
Data analyses
Identification of differentially methylated sites
To identify differentially methylated sites in cord blood that were associated with maternal smoking, we fit a linear regression model with the ComBat-transformed M value at each site as the outcome and maternal smoking (1 = exposed, 0 = unexposed) as the independent variable, adjusting for potential confounders including maternal age, maternal pre-pregnancy BMI category, maternal education level, maternal lifetime stress, gestational age, newborn sex, and cell composition. The false discovery rate (FDR) was estimated to correct for multiple testing, with an FDR <0.05 as the significance cutoff. For the identified significant hit, sensitivity analyses were conducted to test whether the associations with maternal smoking varied after removing very preterm or all preterm newborns. We further tested whether these identified CpG sites were also differentially methylated in maternal blood using the same analytical methods as described above adjusting for the same covariates except newborn sex. All analyses were performed using the ‘limma’ package [Citation57].
Mother-newborn methylation correlation at the identified methylation sites
In this study, there were 89 mother-newborn pairs whose DNAm levels in both maternal blood and cord blood were measured within the same plates, to minimize batch effects. Within these 89 mother-newborn pairs, the correlation coefficients between the cord blood methylation levels and the maternal methylation levels at the identified CpG sites were calculated based on Pearson correlation analysis. P values representing the significance of these correlation coefficients were estimated based on 1010 permutations.
Sex-specific analyses
We performed epigenome-wide cord blood DNAm association tests with maternal smoking in 193 male newborns and 146 female newborns, separately, using similar methods, as described above applying the ‘limma’ package [Citation57]. For CpG sites whose DNAm in cord blood were associated with maternal smoking either in all of the enrolled newborns or in sex-specific groups, we explored maternal smoking × sex interactions by adding an interaction term into the regression models. P values for interactions were calculated based on the likelihood ratio test with one degree of freedom by comparing the null model without the interaction term with the full model with the interaction term.
Modifying effects of maternal folate level
For the identified maternal smoking- related CpG sites in newborns, we then tested whether these associations were modified by maternal folate level, in a subset of 289 newborns with available data on maternal folate level. We first classified maternal folate level into four groups based on quartile (1st, 2nd, 3rd, and 4th quartile). Since the association between maternal smoking and cord blood DNAm at each CpG site was comparable across the 2nd–4th quartile, we then grouped maternal folate level into low (1st quartile, folate level ≤ 19.2 nmol/L) vs. high (2nd–4th quartile, or folate level >19.2 nmol/L) folate strata, and classified all newborns into four subgroups based on maternal smoking status (exposed vs. unexposed) and folate level (low vs. high). DNAm levels at the identified CpG sites were then compared among these four subgroups. Interaction tests between maternal smoking and maternal folate level (low vs. high) were then analyzed using the same methods as described above for maternal smoking × sex interactions.
Supplemental Material
Download MS Word (1.4 MB)Acknowledgments
We thank all of the study participants for their support and help with the study, and Tami R. Bartell for her contribution in editing and revising the manuscript. We are also grateful for the dedication and hard work of the field team at the Department of Pediatrics, Boston University School of Medicine, and for the help and support of the obstetric nursing staff at Boston Medical Center.
Disclosure statement
No potential conflict of interest was reported by the authors.
Supplementary Material
Supplemental data for this article can be accessed here.
Additional information
Funding
References
- Jaddoe VW, Troe EJ, Hofman A, et al. Active and passive maternal smoking during pregnancy and the risks of low birthweight and preterm birth: the Generation R Study. Paediatr Perinat Epidemiol. 2008;22:162–171.
- Wang X, Zuckerman B, Pearson C, et al. Maternal cigarette smoking, metabolic gene polymorphism, and infant birth weight. JAMA. 2002;287:195–202.
- Oken E, Levitan EB, Gillman MW. Maternal smoking during pregnancy and child overweight: systematic review and meta-analysis. Int J Obes (Lond). 2008;32:201–210.
- Zacharasiewicz A. Maternal smoking in pregnancy and its influence on childhood asthma. ERJ Open Res. 2016; 2(3). pii: 00042-2016.
- Gilliland FD, Li YF, Peters JM. Effects of maternal smoking during pregnancy and environmental tobacco smoke on asthma and wheezing in children. Am J Respir Crit Care Med. 2001;163:429–436.
- Obel C, Linnet KM, Henriksen TB, et al. Smoking during pregnancy and hyperactivity-inattention in the offspring–comparing results from three Nordic cohorts. Int J Epidemiol. 2009;38:698–705.
- Langley K, Rice F, van den Bree MB, et al. Maternal smoking during pregnancy as an environmental risk factor for attention deficit hyperactivity disorder behaviour. A review. Minerva Pediatr. 2005;57:359–371.
- Talbott EO, Arena VC, Rager JR, et al. Fine particulate matter and the risk of autism spectrum disorder. Environ Res. 2015;140:414–420.
- Tong VT, Dietz PM, Morrow B, et al. Trends in smoking before, during, and after pregnancy–pregnancy risk assessment monitoring system, United States, 40 sites, 2000-2010. MMWR Surveill Summ. 2013;62:1–19.
- Joubert BR, Felix JF, Yousefi P, et al. DNA Methylation in newborns and maternal smoking in pregnancy: genome-wide consortium meta-analysis. Am J Hum Genet. 2016;98:680–696.
- Joubert BR, Haberg SE, Nilsen RM, et al. 450K Epigenome-wide scan identifies differential DNA methylation in newborns related to maternal smoking during pregnancy. Environ Health Perspect. 2012;120:1425–1431.
- Lee KW, Richmond R, Hu P, et al. Prenatal exposure to maternal cigarette smoking and DNA methylation: epigenome-wide association in a discovery sample of adolescents and replication in an independent cohort at birth through 17 years of age. Environ Health Perspect. 2015;123:193–199.
- Richmond RC, Simpkin AJ, Woodward G, et al. Prenatal exposure to maternal smoking and offspring DNA methylation across the lifecourse: findings from the Avon Longitudinal Study of Parents and Children (ALSPAC). Hum Mol Genet. 2015;24:2201–2217.
- Kupers LK, Xu X, Jankipersadsing SA, et al. DNA methylation mediates the effect of maternal smoking during pregnancy on birthweight of the offspring. Int J Epidemiol. 2015;44:1224–1237.
- Markunas CA, Xu Z, Harlid S, et al. Identification of DNA methylation changes in newborns related to maternal smoking during pregnancy. Environ Health Perspect. 2014;122:1147–1153.
- Bouwland-Both MI, van Mil NH, Tolhoek CP, et al. Prenatal parental tobacco smoking, gene specific DNA methylation, and newborns size: the Generation R study. Clin Epigenetics. 2015;7:83.
- Chhabra D, Sharma S, Kho AT, et al. Fetal lung and placental methylation is associated with in utero nicotine exposure. Epigenetics. 2014;9:1473–1484.
- Harlid S, Xu Z, Panduri V, et al. CpG sites associated with cigarette smoking: analysis of epigenome-wide data from the Sister Study. Environ Health Perspect. 2014;122:673–678.
- Ivorra C, Fraga MF, Bayon GF, et al. DNA methylation patterns in newborns exposed to tobacco in utero. J Transl Med. 2015;13:25.
- Maccani JZ, Koestler DC, Houseman EA, et al. Placental DNA methylation alterations associated with maternal tobacco smoking at the RUNX3 gene are also associated with gestational age. Epigenomics. 2013;5:619–630.
- Miyake K, Kawaguchi A, Miura R, et al. Association between DNA methylation in cord blood and maternal smoking: the Hokkaido study on environment and children’s health. Sci Rep. 2018;8:5654.
- Breton CV, Siegmund KD, Joubert BR, et al. Prenatal tobacco smoke exposure is associated with childhood DNA CpG methylation. PLoS One. 2014;9:e99716.
- Ladd-Acosta C, Shu C, Lee BK, et al. Presence of an epigenetic signature of prenatal cigarette smoke exposure in childhood. Environ Res. 2016;144:139–148.
- Tehranifar P, Wu HC, McDonald JA, et al. Maternal cigarette smoking during pregnancy and offspring DNA methylation in midlife. Epigenetics. 2018;13:129–134.
- Reese SE, Zhao S, Wu MC, et al. DNA Methylation score as a biomarker in newborns for sustained maternal smoking during pregnancy. Environ Health Perspect. 2017;125:760–766.
- Tan SX, Hu RC, Liu JJ, et al. Methylation of PRDM2, PRDM5 and PRDM16 genes in lung cancer cells. Int J Clin Exp Pathol. 2014;7:2305–2311.
- Murphy SK, Adigun A, Huang Z, et al. Gender-specific methylation differences in relation to prenatal exposure to cigarette smoke. Gene. 2012;494:36–43.
- Mandy W, Chilvers R, Chowdhury U, et al. Sex differences in autism spectrum disorder: evidence from a large sample of children and adolescents. J Autism Dev Disord. 2012;42:1304–1313.
- Hartley SL, Sikora DM. Sex differences in autism spectrum disorder: an examination of developmental functioning, autistic symptoms, and coexisting behavior problems in toddlers. J Autism Dev Disord. 2009;39:1715–1722.
- Novakovic B, Ryan J, Pereira N, et al. Postnatal stability, tissue, and time specific effects of AHRR methylation change in response to maternal smoking in pregnancy. Epigenetics. 2014;9:377–386.
- Alshaarawy O, Anthony JC. Month-wise estimates of tobacco smoking during pregnancy for the United States, 2002-2009. Matern Child Health J. 2015;19:1010–1015.
- Shorey-Kendrick LE, McEvoy CT, Ferguson B, et al. Vitamin C prevents offspring DNA methylation changes associated with maternal smoking in pregnancy. Am J Respir Crit Care Med. 2017;196:745–755.
- Fox JT, Stover PJ. Folate-mediated one-carbon metabolism. Vitam Horm. 2008;79:1–44.
- McDowell MA, Lacher DA, Pfeiffer CM, et al. Blood folate levels: the latest NHANES results. NCHS Data Briefs, no. 6. Hyattsville (MD): National Center for Health Statistics; 2008.
- Wang G, Hu FB, Mistry KB, et al. Association between maternal prepregnancy body mass index and plasma folate concentrations with child metabolic health. JAMA Pediatr. 2016;170:e160845.
- News From the Centers for Disease Control and Prevention. Higher folic acid intake needed. JAMA. 2015;313:1094.
- Joubert BR, den Dekker HT, Felix JF, et al. Maternal plasma folate impacts differential DNA methylation in an epigenome-wide meta-analysis of newborns. Nat Commun. 2016;7:10577.
- Amarasekera M, Martino D, Ashley S, et al. Genome-wide DNA methylation profiling identifies a folate-sensitive region of differential methylation upstream of ZFP57-imprinting regulator in humans. FASEB J. 2014;28:4068–4076.
- Glatzel M, Abela E, Maissen M, et al. Extraneural pathologic prion protein in sporadic Creutzfeldt-Jakob disease. N Engl J Med. 2003;349:1812–1820.
- Koperek O, Kovacs GG, Ritchie D, et al. Disease-associated prion protein in vessel walls. Am J Pathol. 2002;161:1979–1984.
- Kovacs GG, Lindeck-Pozza E, Chimelli L, et al. Creutzfeldt-Jakob disease and inclusion body myositis: abundant disease-associated prion protein in muscle. Ann Neurol. 2004;55:121–125.
- Richmond RC, Joubert BR. Contrasting the effects of intra-uterine smoking and one-carbon micronutrient exposures on offspring DNA methylation. Epigenomics. 2017;9:351–367.
- Beach SRH, Lei MK, Ong ML, et al. MTHFR methylation moderates the impact of smoking on DNA methylation at AHRR for African American young adults. Am J Med Genet B Neuropsychiatr Genet. 2017;174:608–618.
- Padmanabhan N, Jia D, Geary-Joo C, et al. Mutation in folate metabolism causes epigenetic instability and transgenerational effects on development. Cell. 2013;155:81–93.
- Dolinoy DC, Huang D, Jirtle RL. Maternal nutrient supplementation counteracts bisphenol A-induced DNA hypomethylation in early development. Proc Natl Acad Sci U S A. 2007;104:13056–13061.
- Barua S, Kuizon S, Chadman KK, et al. Single-base resolution of mouse offspring brain methylome reveals epigenome modifications caused by gestational folic acid. Epigenetics Chromatin. 2014;7:3.
- Wang G, Divall S, Radovick S, et al. Preterm birth and random plasma insulin levels at birth and in early childhood. JAMA. 2014;311:587–596.
- Aryee MJ, Jaffe AE, Corrada-Bravo H, et al. Minfi: a flexible and comprehensive Bioconductor package for the analysis of Infinium DNA methylation microarrays. Bioinformatics. 2014;30:1363–1369.
- Hong X, Sherwood B, Ladd-Acosta C, et al. Genome-wide DNA methylation associations with spontaneous preterm birth in US blacks: findings in maternal and cord blood samples. Epigenetics. 2018;13:163–172.
- Chen YA, Lemire M, Choufani S, et al. Discovery of cross-reactive probes and polymorphic CpGs in the Illumina Infinium HumanMethylation450 microarray. Epigenetics. 2013;8:203–209.
- Johnson WE, Li C, Rabinovic A. Adjusting batch effects in microarray expression data using empirical Bayes methods. Biostatistics. 2007;8:118–127.
- Leek JT, Johnson WE, Parker HS, et al. The sva package for removing batch effects and other unwanted variation in high-throughput experiments. Bioinformatics. 2012;28:882–883.
- Du P, Zhang X, Huang CC, et al. Comparison of Beta-value and M-value methods for quantifying methylation levels by microarray analysis. BMC Bioinformatics. 2010;11:587.
- Jaffe AE, Irizarry RA. Accounting for cellular heterogeneity is critical in epigenome-wide association studies. Genome Biology. 2014;15:R31.
- Houseman EA, Accomando WP, Koestler DC, et al. DNA methylation arrays as surrogate measures of cell mixture distribution. BMC Bioinformatics. 2012;13:86.
- Bakulski KM, Feinberg JI, Andrews SV, et al. DNA methylation of cord blood cell types: applications for mixed cell birth studies. Epigenetics. 2016;11:354–362.
- Smyth GK. Linear models and empirical bayes methods for assessing differential expression in microarray experiments. Stat Appl Genet Mol Biol. 2004;3:1–25.