ABSTRACT
Long noncoding RNAs (lncRNAs) are involved in the regulation of skeletal muscle development. In the present study, differentially expressed lncRNAs were identified from RNA-seq data derived from myoblasts and myotubes. We conducted studies to elucidate the function and molecular mechanism of action of Linc-smad7 during skeletal muscle development. Our findings show that Linc-smad7 is upregulated during the early phase of myoblasts differentiation. In in vitro studies, we showed that overexpression of Linc-smad7 promoted the arrest of myoblasts in G1 phase, inhibited DNA replication, and induced myoblast differentiation. Our in vivo studies suggest that Linc-smad7 stimulates skeletal muscle regeneration in cardiotoxin-induced muscle injury. Mechanistically, Linc-smad7 overexpression increased smad7 and IGF2 protein levels. On the contrary, overexpression of miR-125b reduced smad7 and IGF2 protein levels. Results of RNA immunoprecipitation analysis and biotin-labeled miR-125b capture suggest that Linc-smad7 could act as a competing endogenous RNA (ceRNA) for miRNA-125b. Taken together, our findings suggest that the novel noncoding regulator Linc-smad7 regulates skeletal muscle development.
Introduction
Skeletal muscle is derived from somites during embryonic development [Citation1,Citation2], which is a highly orchestrated process [Citation3,Citation4] that involves various developmental stages of myoblast proliferation, differentiation and, eventually, fusion into multinuclear myotubes and myofibers [Citation5]. These developmental stages are mainly determined by a spectrum of myogenic regulatory factors (MRFs) comprising MyoD [Citation6], myogenic factor 5 (Myf5) [Citation7], myogenin [Citation8], and MRF4 [Citation9], as well as the myocyte enhancer factor 2 (MEF2) family [Citation10]. In addition, during injury-induced skeletal muscle regeneration, in order to repair the damaged muscle, satellite cells are activated and undergo a process that is similar to that of skeletal muscle formation in the embryonic stage [Citation11–Citation13], with an analogous gene regulation mode. Therefore, muscle injury regeneration models are a feasible way of studying gene function during skeletal muscle development in vivo [Citation14]. Extensive in vitro studies of the function of the genes involved in skeletal muscle development have also been performed by culturing the C2C12 murine myoblast cell line, which can reproduce the muscle development process [Citation15].
Long noncoding RNAs (lncRNAs) are a class of endogenous RNAs with little or no protein-coding capacity [Citation16] that are regulated through a complex and diverse regulatory mechanism [Citation17] and are involved in various biological processes, including cell growth, differentiation, and development [Citation18]. It is noteworthy that a small number of lncRNAs have been characterized to regulate myogenesis, including positive regulators, such as MUNC [Citation19], Linc-RAM [Citation20], Lnc-mg [Citation21], LncMyoD [Citation22], Lnc-MD1 [Citation23], Linc-YY1 [Citation24], lncRNA-Dum [Citation15], and certain negative regulators, such as Sirt1 antisense (AS) lncRNAs [Citation25] and Lnc-31 [Citation26]. These studies have shown that lncRNAs have a variety of regulatory functions in epigenetic dynamics and gene expression, and also serve as a molecular sponge for miRNAs, hindering the inhibitory effects of miRNA on the expression of their target genes. However, the regulatory functions of a large number of lncRNAs during skeletal muscle development are still unclear. Therefore, the purpose of our work was to explore the role of lncRNAs in the regulation of myoblast growth, differentiation, and skeletal muscle regeneration.
In this study, we focused on the regulatory function(s) of the long intergenic noncoding RNA smad7 (Linc-smad7) during myogenesis. Linc-smad7 is a novel transcript of lncRNA-smad7 that has been described in a study of murine breast cancer [Citation27]. Interestingly, in transcriptome sequencing data of C2C12 [Citation22], the number of reads for Linc-smad7 is significantly higher in myotubes than in myoblasts, which suggests that Linc-smad7 might be involved in myogenesis. In this study, we observed that expression of Linc-smad7 in C2C12 promotes cell cycle exit and myoblast differentiation in vitro. Likewise, expression of Linc-smad7 in injury-induced muscles promotes skeletal muscle regeneration in vivo. Mechanistically, Linc-smad7 functions by enhancing the expression of the neighboring gene smad7, acting as a molecular sponge to adsorb miR-125b. We therefore describe the functions of a novel lncRNA transcript that regulates myoblasts growth, differentiation, and skeletal muscle regeneration.
Results
Profile of linc-smad7 expression in myocytes and other tissues
In an attempt to identify a functional lncRNA associated with myogenesis, we examined RNA-seq data of differentially expressed lncRNAs during different stages of C2C12 differentiation [Citation22]. A lncRNA located near the smad7 gene (called lncRNA-smad7), which was differentially expressed and enriched in myotubes, had been identified and described to present various splice types in a previous study [Citation27]. In the current study, we focused on the characterization of a novel splice variant of this transcript, Linc-smad7. Using UCSC Genome Browser software, we established that the Linc-smad7 sequence was different from the sequence of two previously known transcripts, lncRNA-smad7-v1 and lncRNA-smad7-v2, and consisted of four exons (). Coding Potential Calculator software (http://cpc.cbi.pku.edu.cn/) was employed to predict the coding potential of Linc-smad7, which was found to be weak (). To verify the differential expression inferred from the RNA-seq data, we analyzed the expression levels of lncRNA-smad7-v1, lncRNA-smad7-v2, Linc-smad7, and total lncRNA-smad7 during myoblast proliferation and differentiation (). The levels of expression of different lncRNA-smad7 transcripts showed a trend of increase during the early stages of differentiation; the expression levels were higher during myoblast differentiation than during myoblast proliferation, corroborating the RNA-seq data. Therefore, we studied Linc-smad7 function in myogenesis. In addition, we quantified the expression levels of markers of muscle differentiation (MyoD, myogenin, and MyHC) during myoblast proliferation and differentiation (Supplementary Fig. 1). The expression levels of the mentioned markers during myoblast development met a priori predictions based on the findings of previous reports [Citation5] and suggested that we are able to accurately quantify Linc-smad7 expression levels as well. To further explore the expression levels of Linc-smad7 in vivo, we quantified its expression in mouse hind-limb muscle tissue during different growth stages. The expression levels of Linc-smad7 showed a trend of increase during the developmental stages (). Moreover, its expression levels were higher in skeletal muscle than in other tissues examined in this study (heart, liver, spleen, lung, kidney, brain, and fat) (), supporting the view that Linc-smad7 might have a regulatory function during myogenesis.
Figure 1. Expression of Linc-smad7 in muscle cells and other mouse tissues. A. Linc-smad7 is a novel splicing variant of lncRNA-smad7. Boxes indicate exons; arrows indicate the direction of transcription. B. Coding potential of Linc-smad7 predicted by Coding Potential Calculator. C. Expression level of lncRNA-smad7-v1 during C2C12 myoblast proliferation and differentiation. D. Expression level of lncRNA-smad7-v2 during myoblast proliferation and differentiation. E. Expression level of Linc-smad7 during myoblast proliferation and differentiation. F. Expression level of total lncRNA-smad7 transcripts during myoblast proliferation and differentiation. G. Expression level of Linc-smad7 in mouse hind limb muscle. X-axis indicates mouse age. H. Expression level of Linc-smad7 in heart, liver, spleen, lung, kidney, brain, fat, and skeletal muscle tissues. Data are presented as mean ± SEM. n = 3.
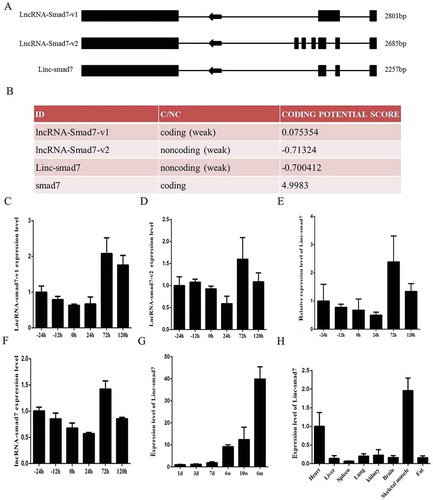
Linc-smad7 inhibits myoblast growth
Linc-smad7 expression levels decreased during myoblast proliferation (), suggesting that Linc-smad7 could be involved in the regulation of cell growth. We used RNA interference to reduce the levels of Linc-smad7 in C2C12 myoblast cells (). Using flow cytometry analysis, we observed that the number of cells in G1 phase was lower in siRNA-treated cells than that in the control group (). In addition, interference of Linc-smad7 with siRNA was associated with enhanced DNA replication, as shown by the EdU proliferation assay in . On the other hand, overexpression of Linc-smad7 using an overexpression plasmid (see Materials and Methods; ) was associated with an increase in the number of cells in G1 phase () and reduced DNA replication (). Taken together, these results support the idea that Linc-smad7 inhibits C2C12 myoblast growth.
Figure 2. Effect of Linc-smad7 on myoblast growth. A. Expression level of Linc-smad7 24 h after transfection of C2C12 with siLinc-smad7. NC: control cells. siRNA: si-Linc-smad7-treated cells. B. Flow cytometry analysis after transfection with siLinc-smad7 (n = 2). C. Histogram bar graph summarizing the flow cytometry analysis showing the number of cells in each cell cycle phase. D. EdU proliferation assay showing the effect of siLinc-smad7 on myoblasts performed 24 h after transfection. The red fluorescent cells are in the S phase of mitosis; the blue fluorescent cells (nuclei stained with Hoechst 33,342) represent all the cells. E. Histogram bar graph showing the ratio of EdU-positive cells in NC and siLinc-sma7-trated cells. F. Expression level of Linc-smad7 in myoblasts transfected with the Linc-smad7 expression plasmid (pcDNA3.1) and NC. G. Flow cytometry analysis performed 24 h after transfection with Linc-smad7 expression plasmid. H. Histogram bar graph summarizing the flow cytometry analysis in (G). I. EdU proliferation assay performed 24 h after transfection with Linc-smad7 expression plasmid. J. Histogram bar graph showing the ratio of EdU-positive cells in NC and cells transfected with the Linc-smad7 myoblasts Results are presented as the mean ± SEM. n = 3. *P < 0.05; **P < 0.01.
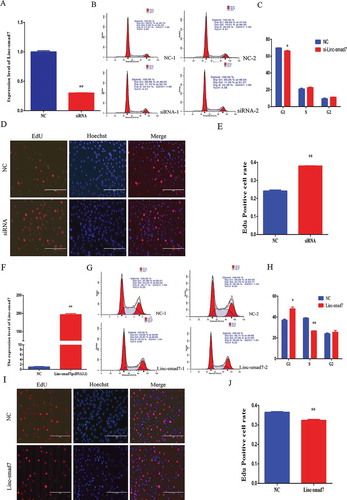
Linc-smad7 promotes myoblast differentiation
Myoblasts exit the cell cycle during their differentiation process, which is considered a key specific step in myoblast differentiation. The above results indicated that Linc-smad7 was essential for cell cycle withdrawal. This prompted the question of whether Linc-smad7 might act as a myogenic regulator during myoblast differentiation. Again, we used siRNA against Linc-smad7 and a Linc-smad7 overexpression vector to test the involvement of Linc-smad7 in myoblast differentiation. We observed that interference of Linc-smad7 was associated with a decline in the mRNA and protein levels of the myogenic markers MyoD, myogenin, and MyHC, as compared to their respective levels in the control group (). By immunofluorescence staining, we observed that the knockdown of Linc-smad7 reduced the number of nuclei in myosin-positive myotubes (). However, overexpression of Linc-smad7 enhanced the mRNA and protein levels of MyoD, myogenin, and MyHC (). In addition, the number of nuclei in myosin-positive cells was higher in cells transfected with the Linc-smad7 overexpression plasmid than in the control group (). Hence, our results suggest that Linc-smad7 promotes myoblast differentiation.
Figure 3. Effect of Linc-smad7 on myoblast differentiation. A. mRNA level of myogenic marker genes MyoD, MyoG, and MyHC 120 h after transfection of C2C12 cells with si-Linc-smad7. B. Western blot of C2C12 cells 120 h after transfection with si-Linc-smad7 using antibodies against MyoD, MyoG, and MyHC. C. Histogram bar graph after Western blot signal quantification indicating the protein level of myogenic markers after transfection with si-Linc-smad7. D. Immunofluorescence staining for myosin 120 h after transfection with si-Linc-smad7. E. Number of nuclei in myosin-positive myotubes 120 h after transfection with si-Linc-smad7. F-J. Idem as in (A-E) but after transfection with Linc-smad7 expression plasmid. Data are represented as mean ± SEM. *P < 0.05, **P < 0.01.
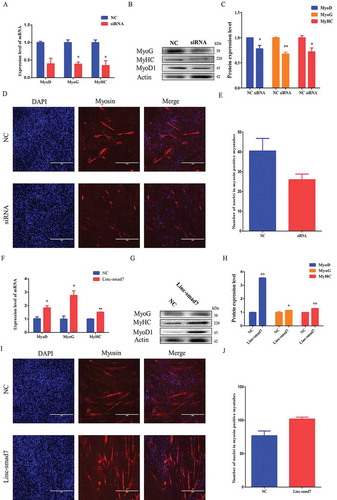
Linc-smad7 promotes skeletal muscle regeneration
In vivo experiments were essential to further verify the function of Linc-smad7 in skeletal muscle development. First, we injected Cardiotoxin (CTX) into the tibialis anterior (TA) muscle of mice to induce muscle damage. On the next day, the expression of the marker gene Pax7, a key gene for the activation of satellite cells[Citation28], was significantly higher in the CTX-injected group than that in the control group, indicating that the model of muscle damage was successfully established (). Twelve hours after CTX injection, we experimentally increased Linc-smad7 expression levels by transfecting a Linc-smad7 overexpression plasmid (see Materials and Methods). Seven days after the skeletal muscle injury, the expression level of Linc-smad7 was considerably induced ()), and MyoD, MyoG, MyHC, smad7, and IGF2 protein levels were also higher in transfected cells than in the control (). Hematoxylin and eosin (HE) staining and immunofluorescence staining with anti-myosin antibody suggested that substantial muscle fiber repair had occurred in the treatment group, where the numbers of muscle fibers were higher than in the control group (, Supplementary Fig.2A). In contrast, injured TA treated with interference RNA against Linc-smad7, the levels of Linc-smad7 were reduced () and the protein levels of myogenesis markers were also lowered (). Muscle fiber integrity was deteriorated in the si-Linc-smad7 group compared to the control group (, Supplementary Fig.2B).
Figure 4. Linc-smad7 promotes skeletal muscle regeneration. A. HE-staining of tibialis anterior (TA) muscle 48 h after injection of CTX into the muscle. B. Level of Pax7 mRNA in TA 48 h after the injection of CTX. C. Linc-smad7 levels 7 days after transfection of the Linc-smad7 expression plasmid in vivo. D. Western blot detecting protein levels of myogenic-related genes 7 days after transfection of TA with the Linc-smad7 expression plasmid. E. Histogram bar graph of the signal quantification of the Western blot in (D) . F. HE-staining of CTX-injected muscle after transfection of the Linc-smad7 expression plasmid. G-J. Idem as in (C-F) but after transfection of siLinc-smad7. Data are represented as mean ± SEM. n = 3. *P < 0.05, **P < 0.01.
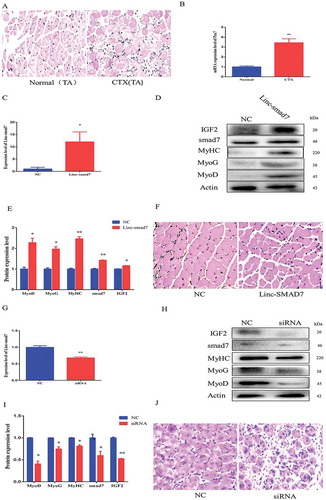
Gene expression profile of injury-induced muscle overexpressing linc-smad7
To further study the involvement of Linc-smad7 in skeletal muscle regeneration, we analyzed RNA-seq data obtained from control and Linc-smad7 overexpression treatment groups and identified differentially expressed genes that were associated with myogenesis. Compared to the control group, 359 differentially expressed genes were identified in the treated group (Supplementary information). More specifically, 121 genes were upregulated, and 238 genes were downregulated (). Kyoto Encyclopedia of Genes and Genomes (KEGG) pathway enrichment analysis revealed that some upregulated genes were involved in the biosynthesis of amino acids pathway ()) and certain downregulated genes were enriched in the TNF signaling pathway (). Gene Ontology (GO) enrichment analysis of upregulated genes identified several ‘biological process’ terms, including ‘skeletal muscle tissue development’ and ‘skeletal muscle organ development’ (). The top terms for downregulated genes were mainly related to ‘immune response’ functions, among biological processes ()). Whereas a few of the upregulated genes (e.g., Srpk3 [Citation29], Actc1 [Citation30]) have a known function in regulating skeletal muscle development, some of the identified downregulated genes (e.g., BMP2 [Citation31],TNF [Citation32]) have been shown to suppress the development of skeletal muscles. Our results suggest that Linc-smad7 has clear effects on skeletal muscle regeneration. To further validate our RNA-seq results, we detected the expression level of several genes using qRT-PCR. The RT-qPCR results were consistent with the data from the RNA-seq: Srpk3, Bin1, Ankrd1, Tomd1, Foxp2, Ctnna3, Adrb2, Actc1 were upregulated; Bmp2, Cxcl9, Cxcl10, TNF, Stc1, IL6 were downregulated ().
Figure 5. Effect of Linc-smad7 on the muscle regeneration transcriptome. A. Volcano plot representing the number of differentially expressed genes after transfection of TA muscles with the Linc-smad7 expression plasmid: 121 upregulated and 238 downregulated genes were identified. B. KEGG pathway scatterplot depicting the number of upregulated genes in pathway enrichment after transfection of the Linc-smad7 expression plasmid. Rich factor refers to the number of differentially expressed genes in the pathway C. KEGG pathway scatterplot showing the number of downregulated genes in pathway enrichment after transfection of the Linc-smad7 expression plasmid. D. GO enrichment analysis of genes that were upregulated 7 days after transfection of TA muscles with Linc-smad7 expression plasmid. The y-axis denotes the top ten enriched biological processes; the x-axis indicates the number of genes. E. GO enrichment analysis of genes that were downregulated after transfection of the Linc-smad7 expression plasmid. F. qRT-PCR of selected genes involved in muscle development and immune response . Data are represented as mean ± SEM. n = 3. *P < 0.05, **P < 0.01.
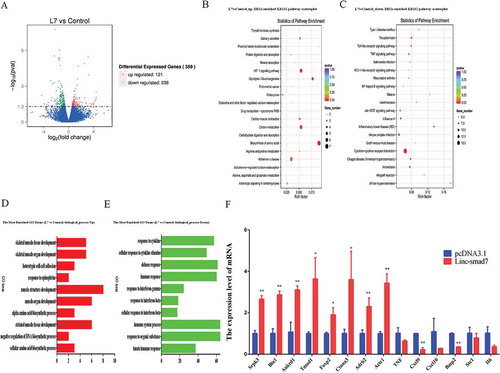
Linc-smad7 sponges miR-125b to regulate muscle development
LncRNAs can affect developmental processes by sponging regulatory miRNAs[Citation23]. By analyzing the intracellular occurrence of Linc-smad7, we observed that the lncRNA was present in both the nucleus and the cytoplasm (), which suggested the possibility that Linc-smad7 sponges miRNAs. Using TargetScan (http://www.targetscan.org/vert_71/) and RNAhybrid software (https://bibiserv.cebitec.uni-bielefeld.de/rnahybrid/) the seed regions of miR-125b were predicted to be complementary with Linc-smad7 (). We constructed wild-type and mutant versions of Smad7-UTR and Linc-smad7 using psi-Check2 vectors (see Materials and Methods) (). Then, RNA was immunoprecipitated using an antibody against Ago2, followed by detection of Linc-smad7 by RT-PCR (). In addition, we established that the expression of Linc-smad7 in the biotin-miR-125b group was about 10 times that of the control group ()). Using a dual-luciferase report assay, overexpression of miR-125b was associated with a decrease of luciferase activity of Linc-smad7 and smad7 wild type psi-Check2 vectors, but had no effect on the mutant version of the vector (). We therefore confirmed that miR-125b targeted Linc-smad7 and its downstream-located gene smad7. To further investigate if Linc-smad7 sponged miR-125b to regulate myoblast differentiation and skeletal muscle regeneration, smad7 and IGF2 protein levels were detected during myoblast differentiation after transfection with the Linc-smad7 overexpression plasmid () or its respective siRNA (). These results were consistent with our previous analyses of skeletal muscle regeneration (). Overexpression of miR-125b was associated with decreased smad7 and IGF2 protein levels ().
Figure 6. Linc-smad7 is a target of miR-125b during myogenesis. A. Expression level of Linc-smad7, LncRNA-smad7, Neat1, and actin in C2C12 myoblast cell nucleus and cytoplasm detected by agarose gel electrophoresis. B. Sequence information of relevant regions of miR-125b, Linc-smad7, and 3’UTR of smad7. The sequence in red represents the seed region of miR-125b and its target region. C. Complementary pairing between the sequences of miR-125b-5p and Linc-smad7. The right scheme shows the calculated ΔG value. D. Peak map of psi-check2 vector sequencing results of Linc-smad7 and smad7 3’UTR sequences. The red boxes represent the mutant bases. E. RIP assay was conducted using an antibody against Ago2. Linc-smad7 was detected by agarose gel electrophoresis. U1 snRNA was used as a control. F. qRT-PCR of C2C12 myoblasts transfected with biotin-miR-NC or biotin-miR-125b to detect Linc-smad7. G. Linc-smad7 wild or mutant type psi-check2 plasmid was transfected into HEK293T cells together with miR-125b mimic. Luciferase activities were measured 24 h after transfection. H. Smad7 wild or mutant type psi-check2 plasmids was transfected into HEK293T cells together with miR-125b mimic. Luciferase activities were measured 24 h after transfection. I,J. Cells transfected with Linc-smad7 expression vector. K,L. Cells transfected with siRNA. Detection of IGF2 and smad7 protein levels by Western blot. M,N. Detection of IGF2 and smad7 protein levels by Western blot after overexpression of miR-125b. Data are represented as mean ± SEM. *P < 0.05, **P < 0.01.
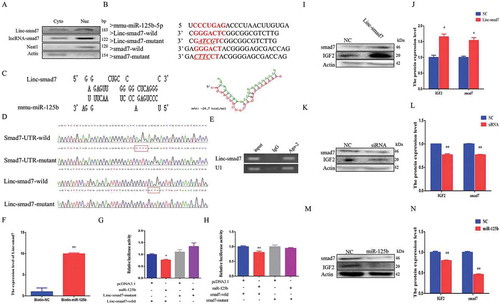
Discussion
In this study, we propose a novel function for Linc-smad7 in C2C12 differentiation and skeletal muscle regeneration. Our in vitro experiments suggest that Linc-smad7 expression increases during myoblast differentiation, and, serving as a ceRNA, promotes myogenesis by sponging miR-125b via targeting IGF2 and smad7 (). Moreover, our in vivo experiments also suggest that overexpression of Linc-smad7 promotes regeneration.
Figure 7. Model of Linc-smad7 regulatory mechanism on myogenesis. In this model, Linc-smad7 regulates myogenesis by functioning as a ceRNA that sequesters miR-125b, thereby relieving its repressive effect on IGF2 and smad7 to promote skeletal muscle development.
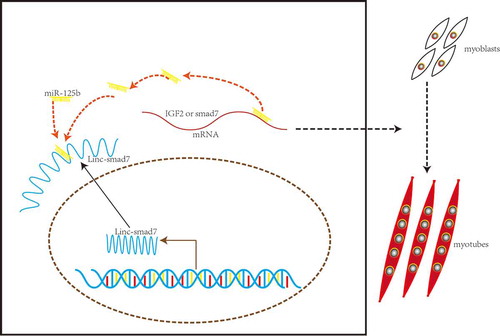
To provide further insights into the role played by Linc-smad7 during skeletal muscle regeneration, we assessed differential gene expression during myogenesis after Linc-smad7 overexpression using an RNA-seq approach[Citation10]. Upregulated genes mainly participate in biosynthesis of amino acids pathways and skeletal muscle tissue development, suggesting that Linc-smad7 may be involved in skeletal muscle regeneration. Muscle damage is often accompanied by an inflammatory response[Citation32]. Both KEGG pathway and GO terms analysis indicate that certain inflammatory factors, such as TNF[Citation33], were downregulated after overexpression of Linc-smad7. These finding suggest that Linc-smad7 effects on muscle repair function via a reduction of the inflammatory response.
Linc-smad7 was identified from previously published transcriptome data obtained during the proliferation and differentiation phases of C2C12 [Citation22]. It is noteworthy that this transcript is different from other types of lncRNA-smad7 [Citation27], and had not been studied before. A considerable number of studies have examined the regulatory functions and molecular mechanisms of lncRNAs, which are involved in various developmental processes [Citation17,Citation34]. However, the study of lncRNAs functioning as competing endogenous RNAs (ceRNAs) in myogenesis is still at its infancy, with only a handful characterized to date. For example, linc-MD1 sponges two regulatory miRNAs (miR-133 and miR-135) to enhance the expression of the transcription factors MAML1 and MEF2C during the process of muscle differentiation in mouse and human myoblasts[Citation35]. An alternative mechanism is exemplified by lncRNA H19, which promotes skeletal muscle differentiation and regeneration in mouse. This effect is mediated by the action of miR-675-3p and miR-675-5p, which are encoded in H19 exon 1[Citation36]. Previous work suggests that lncRNAs in cytoplasm are more likely to function as molecular sponges [Citation18,Citation37]. We found that Linc-smad7 was located in the cytoplasm and considered that it might serve as a ceRNA to regulate myogenesis. Our RNA binding immunoprecipitation assay using an antibody against Ago2 suggested that Linc-smad7 had the potential to combine with miRNAs. Luciferase screening and biotin-miR-125b capture results revealed that Linc-smad7 is a direct target of miR-125b. A dual luciferase report system was also used in previous studies to characterize the interaction between lncRNAs and miRNAs[Citation38–Citation40]. We found that Linc-smad7 and miR-125b (using myoblasts and a skeletal muscle regeneration model) produced an opposite effect in myoblast differentiation, suggesting that miRNA sponge effect by lncRNAs is a vital mechanism. IGF2 and smad7 were identified as targets of miR-125b in C2C12 cells. It is known that IGF2[Citation41] and smad7[Citation42,Citation43] promote myoblast differentiation. Therefore, we speculated that the effects of Linc-smad7 on myogenesis were due to miR-125b-mediated regulation of IGF2 and smad7. We propose that Linc-smad7 modulates IGF2 and smad7 expression by competing for miR-125b as a ceRNA to regulate myogenic differentiation. The ceRNA regulatory network (Linc-smad7/miR-125b/IGF2 or smad7) sheds light on the mechanisms of noncoding RNA regulation of muscle development. It is worth pointing out that Linc-smad7 was also detected in the nucleus and, thus, further studies are required to examine whether it regulates muscle development through other mechanisms as well.
In conclusion, Linc-smad7 was found to inhibit myoblast growth and promote myoblast differentiation and skeletal muscle regeneration by sponging miR-125b. Linc-smad7 might be an interesting candidate to use in future experiments to investigate the molecular mechanisms by which lncRNAs affect developmental processes.
Materials and methods
Animal research
Mice (C57BL/6 strain) were purchased from the Fourth Military Medical University (Xi’an, China). Animal care and study protocols were approved by the Animal Care Commission of the College of Veterinary Medicine, Northwest A&F University. Cardiotoxin (CTX; Whiga) was injected as a 50-µL CTX solution (10 µΜ) into the tibialis anterior muscle of ~ 5-week-old mice to induce skeletal muscle damage. To increase the expression of the lncRNA, we prepared Linc-smad7 pcDNA3.1 expression plasmid by preincubating 6.25 µg of pcDNA3.1 with EntransterTM-in vivo transfection reagent (Engreen Biosystem Co., Ltd.) in a final volume of 50 µl in 10% glucose solution. The mixture was injected into the tibialis anterior muscle of 3 mice per treatment group. Injection time was 12, 48, and 96 h after CTX treatment. Another 3 mice served as the control group and were injected a plasmid-free glucose solution. Interference of Linc-smad7 was done by transfecting siRNA into skeletal muscle. After CTX treatment (144 h) tibialis anterior muscles were harvested for RNA and protein extraction, and to obtain paraffin sections. Paraffin sections were used for HE staining and immunofluorescence staining according to manufacturer’s instructions. Detailed descriptions of our experimental protocols can be found in previous studies [Citation15,Citation20].
Cell culture and transfections
C2C12 myoblasts and 293T cells (ATCC) were cultured in Dulbecco’s modified Eagle’s medium (DMEM) with 10% FBS and 1% penicillin/streptomycin at 37°C under a 5% CO2 atmosphere. At confluence, the growth medium was replaced with 2% horse serum medium with 1% penicillin/streptomycin to initiate myoblast differentiation. Linc-smad7 expression plasmid (pcDNA3.1) was constructed using the ClonExpress II One Step Cloning Kit (Vazyme Biotech). Linc-smad7 siRNA (5ʹ-AAGAGUUGGAGUCCGCCAAACUAGG-3ʹ) was purchased from GenePharma. Linc-smad7-expressing plasmid or Linc-smad7 siRNA, miR-125b mimic (GenePharma) was transfected into C2C12 myoblasts using Lipofectamin 2000 (Invitrogen) following manufacturer’s instructions. PCR primer sequences are listed in Supplementary Table 1.
Cell cycle assay
C2C12 myoblasts were transfected with Linc-smad7 expression plasmid or Linc-smad7 siRNA and treated with cell cycle staining kit and analyzed by flow cytometry (Becton Dickinson, FACSCalibur). Data were analyzed using ModFit Software (Verity Software House).
Edu cell proliferation assay
Cell-Light™ EdU Apollo®567 in vitro Imaging Kit (Ribobio) was used to detect cell proliferation, following the manufacturer’s instructions. Positive cell rate (EdU-stained cells/total cells) was calculated using a fluorescence microscope (AMG EVOS).
Gene expression analyses
Total RNA of cells and tissues was extracted using Trizol Reagent (TaKaRa). Reverse transcription was conducted with about 1 μg of total RNA using the PrimeScript™ RT Reagent Kit, which includes a gDNA eraser (TaKaRa). Gene expression levels were detected by RT-PCR using the SYBR Green Kit (Genestar) on a CFX96 RT-PCR Detection System (Bio-Rad). Primer sequences for qRT-PCR are listed in Supplementary Table 1.
Nuclear and cytoplasmic RNA fractionation
Nuclear/cytoplasmic RNA fractionation was accomplished in C2C12 myoblasts using Nucleoprotein Extraction Kit (Sangon Biotech). Nuclear and cytoplasmic RNA was extracted and transcribed into cDNA for further analysis. The locations of Linc-smad7, Actin (cytoplasmic marker gene), and Neat1 (a nuclear expression lncRNA) [Citation20] were analyzed by PCR. Primer sequences for PCR are listed in Supplementary Table 1.
Protein extraction and western blot
Cells were lysed with RIPA buffer (Solarbio) to extract total proteins. Protein concentration was determined using a BCA Kit (Beyotime). The proteins were separated in 12% SDS-polyacrylamide gel electrophoresis and transferred to polyvinylidene fluoride membranes. The membranes were exposed with ECL Plus (Solarbio) and quantified with the ChemiDoc XRS+ system (Bio-Rad Laboratories). The primary antibody and secondary antibody are listed in Supplementary Table 2.
Rna-sequencing
RNA-seq was used to characterize transcriptomes of the CTX-injected muscle after Linc-smad7 overexpression treatment (L7) and the control group (3 biological replicates per group). A total amount of 3 µg RNA per sample was used for library construction. We generated sequencing libraries using the NEBNext® Ultra™ RNA Library Prep Kit for Illumina® (NEB) following the manufacturer’s recommendations and added index codes to attribute sequences to each sample (Novogene). Differentially expressed genes (Padjusted < 0.05) between the two groups were screened using the DESeq package in R. KEGG pathway enrichment analysis was performed to identify the most important biochemical, metabolic, and signal transduction pathways involved (using KOBAS 2.0). Genes were considered differentially expressed between the two groups if Pcorrected < 0.05. Gene Ontology (GO) enrichment analysis of differentially expressed genes was performed using the GOseq package of R, which corrects for biases due to different gene lengths.). Genes were considered differentially expressed between the two groups if Pcorrected < 0.05.
RNA-binding immunoprecipitation assay
RNA-binding protein immunoprecipitation (RIP) was used to identify specific RNA molecules associated with the Ago2 protein. We followed the manufacturer’s instructions for the EZ-Magna RIP Kit (Millipore). Briefly, we lysed cells in RIP lysis buffer and then immunoprecipitated the lysate with antibody against RNA binding protein Ago2 (Abcam) using protein A/G magnetic beads.
Biotin-labeled miR-125b capture
C2C12 cells were lysed after transfection with biotin-labeled miR-125b (Ribobio), using biotin-labeled miRNA mimic as negative control. The supernatant was added to 30 µl beads (Dynabeads MyOne Streptavidin C1; Invitrogen) to absorb biotin-labeled RNA. Detailed steps can be found in a previous study [Citation21]. TRIzol Reagent was used to extract RNA from the beads that remained after magnetic adsorption. Gene expression of Linc-smad7 was analyzed by RT-qPCR.
Dual luciferase reporter assay
Wild type and mutant partial length sequences (containing miR-125b seed sequence target sites) of Linc-smad7 and smad7 3′UTR were cloned into the psi-Check2 vector with T4 DNA ligase (TakaRa), and confirmed by sequencing (Sangon Biotech) . These plasmids and miR-125b mimic were co-transfected into 293T cells separately. Twenty-four hours after transfection, cells were lysed and luciferase report analysis performed using Dual-Luciferase® Reporter Assay System (Promega).
Immunofluorescence staining of myotubes
The number of nuclei in myosin-positive myoblast was determined by immunofluorescence staining. In short, C2C12 cells were washed three times with PBS buffer, fixed with 4% paraformaldehyde in PBS buffer for 30 min, and permeabilized by treatment with 0.5% Triton X-100 for 10 min. Cells were blocked with 5% BSA for 30 min, incubated with anti-myosin antibody at 37°C for 2 hours, and then incubated with Cy3-conjugated secondary antibody for 1 hour at room temperature. Nuclei were stained with DAPI for 10 min. All images were taken on an inverted fluorescence microscope (AMG EVOS). The primary and secondary antibodies are listed in Supplementary Table 2.
Statistical analysis
Generally, all data are presented as mean ± SEM. P values for the statistical comparison of control vs. treatment group were calculated using two-tailed Student’s t-tests.
Supplemental Material
Download Zip (434.3 KB)Acknowledgments
This work was supported by the National Natural Science Foundation of China [Grant No. 31772574], the Program of National Beef Cattle and Yak Industrial Technology System [CARS-38], Special Fund of Xinyang Normal University (No.2017001).
Disclosure statement
No potential conflict of interest was reported by the authors.
Supplementary information
Supplementary information is available at https://www.ncbi.nlm.nih.gov/geo/query/acc.cgi?acc=GSE106365
Additional information
Funding
References
- Buckingham M. Gene regulatory networks and cell lineages that underlie the formation of skeletal muscle. Proc Natl Acad Sci U S A. 2017 Jun 6;114(23):5830–5837. PubMed PMID: 28584083; PubMed Central PMCID: PMC5468682.
- Buckingham M, Bajard L, Chang T, et al. The formation of skeletal muscle: from somite to limb. J Anat. 2003;202(1):59–68.
- Buckingham M. Myogenic progenitor cells and skeletal myogenesis in vertebrates. Curr Opin Genet Dev. 2006 Oct;16(5):525–532. PubMed PMID: 16930987.
- Bismuth K, Relaix F. Genetic regulation of skeletal muscle development. Exp Cell Res. 2010 Nov 1;316(18):3081–3086. PubMed PMID: 20828559.
- Bentzinger CF, Wang Y, Rudnicki MA. Building muscle: molecular regulation of myogenesis. Cold Spring Harb Perspect Biol. 2012 Feb;4(2). PubMed PMID: 22300977; PubMed Central PMCID: PMC3281568.
- Buckingham M, Rigby P. Gene regulatory networks and transcriptional mechanisms that control myogenesis. Dev Cell. 2014 Feb 10;28(3):225–238. PubMed PMID: 24525185.
- Conerly ML, Yao Z, Zhong JW, et al. Distinct activities of Myf5 and MyoD indicate separate roles in skeletal muscle lineage specification and differentiation. Dev Cell. 2016 Feb 22;36(4):375–385.. PubMed PMID: 26906734; PubMed Central PMCID: PMC4769793.
- Brunetti AGI. Role of Myogenin in Myoblast differentiation and its regulation by fibroblast growth factor. J Biol Chem. 1990;265(11):5960–5963.
- Moretti I, Ciciliot S, Dyar KA, et al. MRF4 negatively regulates adult skeletal muscle growth by repressing MEF2 activity. Nat Commun. 2016;7:12397. PubMed PMID: 27484840.
- Estrella NL, Desjardins CA, Nocco SE, et al. MEF2 transcription factors regulate distinct gene programs in mammalian skeletal muscle differentiation. J Biol Chem. 2015 Jan 9;290(2):1256–1268. PubMed PMID: 25416778; PubMed Central PMCID: PMC4294490.
- Collins CA, Olsen I, Zammit PS, et al. Stem cell function, self-renewal, and behavioral heterogeneity of cells from the adult muscle satellite cell niche. Cell. 2005 Jul 29;122(2):289–301. PubMed PMID: 16051152.
- Dumont NA, Bentzinger C, Sincennes MC, et al. Satellite cells and skeletal muscle regeneration. Compr Physiol. 2015 Jul 1;5(3):1027–1059. PubMed PMID: 26140708.
- Bentzinger CF, Wang Y, Dumont NA, et al. Cellular dynamics in the muscle satellite cell niche. EMBO Reports. 2013 Dec;14(12):1062–1072.. PubMed PMID: 24232182; PubMed Central PMCID: PMC3849491.
- Ge Y, Sun Y, Chen J. IGF-II is regulated by microRNA-125b in skeletal myogenesis. J Cell Biol. 2011 Jan 10;192(1):69–81. PubMed PMID: 21200031; PubMed Central PMCID: PMC3019547.
- Wang L, Zhao Y, Bao X, et al. LncRNA Dum interacts with Dnmts to regulate Dppa2 expression during myogenic differentiation and muscle regeneration. Cell Res. 2015 Mar;25(3):335–350.. PubMed PMID: 25686699; PubMed Central PMCID: PMC4349245.
- Wilusz JE, Sunwoo H, Spector DL. Long noncoding RNAs: functional surprises from the RNA world. Genes Dev. 2009 Jul 1;23(13):1494–1504.. PubMed PMID: 19571179; PubMed Central PMCID: PMC3152381.
- Kopp F, Mendell JT. Functional classification and experimental dissection of long noncoding RNAs. Cell. 2018 Jan 25;172(3):393–407.. PubMed PMID: 29373828.
- Neguembor MV, Jothi M, Gabellini D. Long noncoding RNAs, emerging players in muscle differentiation and disease. Skelet Muscle. 2014;4:8.
- Mueller AC, Cichewicz M, Dey BK, et al. MUNC, a long noncoding RNA that facilitates the function of MyoD in skeletal myogenesis. Mol Cell Biol. 2015 Feb;35(3):498–513.. PubMed PMID: 25403490; PubMed Central PMCID: PMC4285423.
- Yu X, Zhang Y, Li T, et al. Long non-coding RNA Linc-RAM enhances myogenic differentiation by interacting with MyoD. Nat Commun. 2017 Jan 16;8:14016.
- Zhu M, Liu J, Xiao J, et al. Lnc-mg is a long non-coding RNA that promotes myogenesis. Nat Commun. 2017 Mar 10;8:14718.
- Gong C, Li Z, Ramanujan K, et al. A long non-coding RNA, LncMyoD, regulates skeletal muscle differentiation by blocking IMP2-mediated mRNA translation. Dev Cell. 2015 Jul 27;34(2):181–191. PubMed PMID: 26143994.
- Cesana M, Cacchiarelli D, Legnini I, et al. A long noncoding RNA controls muscle differentiation by functioning as a competing endogenous RNA. Cell. 2011;147(4):947.
- Zhou L, Sun K, Zhao Y, et al. Linc-YY1 promotes myogenic differentiation and muscle regeneration through an interaction with the transcription factor YY1. Nat Commun. 2015;6:10026. PubMed PMID: 26658965.
- Wang GQ, Wang Y, Xiong Y, et al. Sirt1 AS lncRNA interacts with its mRNA to inhibit muscle formation by attenuating function of miR-34a. Sci Rep. 2016 Feb 23;6:21865. PubMed PMID: 26902620; PubMed Central PMCID: PMC4763196.
- Ballarino M, D’Andrea D, Grassi L, et al. Novel long noncoding RNAs (lncRNAs) in myogenesis: a miR-31 overlapping lncRNA transcript controls myoblast differentiation. Mol Cell Biol. 2015;354:728–736. PubMed Central PMCID: PMCPMC4301723
- Arase M, Horiguchi K, Ehata S, et al. Transforming growth factor-beta-induced lncRNA-Smad7 inhibits apoptosis of mouse breast cancer JygMC(A) cells. Cancer Sci. 2014 Aug;105(8):974–982. PubMed PMID: 24863656; PubMed Central PMCID: PMC4317863.
- Seale P, Sabourin L, Girgis-Gabardo A, et al. Pax7 is required for the specification of myogenic satellite cells. Cell. 2000;102(6):777–786.
- Xu Y, Yu W, Xiong Y, et al. Molecular characterization and expression patterns of serine/arginine-rich specific kinase 3 (SPRK3) in porcine skeletal muscle. Mol Biol Rep. 2011 Jun;38(5):2903–2909. PubMed PMID: 20127522.
- Boutilier JK, Taylor R, Ram R, et al. Variable cardiac alpha-actin (Actc1) expression in early adult skeletal muscle correlates with promoter methylation. Biochim Biophys Acta. 2017 Oct;1860(10):1025–1036. PubMed PMID: 28847732.
- Nakashima A, Katagiri T, Tamura M. Cross-talk between Wnt and bone morphogenetic protein 2 (BMP-2) signaling in differentiation pathway of C2C12 myoblasts. J Biol Chem. 2005 Nov 11;280(45):37660–37668.. PubMed PMID: 16150699.
- Coletti D, Yang E, Marazzi G, et al. TNFα inhibits skeletal myogenesis through a PW1-dependent pathway by recruitment of caspase pathways. EMBO J. 2002;21(4):631–642.
- Liao P, Zhou J, Ji LL, et al. Eccentric contraction induces inflammatory responses in rat skeletal muscle: role of tumor necrosis factor-alpha. Am J Physiol Regul Integr Comp Physiol. 2010;298(3):R599–607.
- Fatica A, Bozzoni I. Long non-coding RNAs: new players in cell differentiation and development. Nat Reviews Genet. 2014 Jan;15(1):7–21. PubMed PMID: 24296535.
- Derrien T, Guigó R, Johnson R. The long non-coding RNAs: a new (P)layer in the “Dark Matter”. Front Genet. 2011;2:107. PubMed PMID: 22303401; PubMed Central PMCID: PMC3266617.
- Dey BK, Pfeifer K, Dutta A. The H19 long noncoding RNA gives rise to microRNAs miR-675-3p and miR-675-5p to promote skeletal muscle differentiation and regeneration. Genes Dev. 2014 Mar 1;28(5):491–501. PubMed PMID: 24532688; PubMed Central PMCID: PMC3950346.
- Wang Y, Xu Z, Jiang J, et al. Endogenous miRNA sponge lincRNA-RoR regulates Oct4, Nanog, and Sox2 in human embryonic stem cell self-renewal. Dev Cell. 2013 Apr 15;25(1):69–80. PubMed PMID: 23541921.
- Li M, Sun X, Cai H, et al. Long non-coding RNA ADNCR suppresses adipogenic differentiation by targeting miR-204. Biochim Biophys Acta. 2016 Jul;1859(7):871–882. PubMed PMID: 27156885.
- Han P, Li J, Zhang BM, et al. The lncRNA CRNDE promotes colorectal cancer cell proliferation and chemoresistance via miR-181a-5p-mediated regulation of Wnt/beta-catenin signaling. Mol Cancer. 2017 Jan 13;16(1):9. 10.1186/s12943-017-0583-1. PubMed PMID: 28086904; PubMed Central PMCID: PMC5237133.
- Wang K, Liu CY, Zhou LY, et al. APF lncRNA regulates autophagy and myocardial infarction by targeting miR-188-3p. Nat Commun. 2015 Apr 10;6:6779. PubMed PMID: 25858075.
- Florini JR, Magri K, Ewton DZ, et al. “Spontaneous” differentiation of skeletal myoblasts is dependent upon autocrine secretion of insulin-like growth factor-II. J Biol Chem. 1991;266(24):15917–15923.
- Kollias HD, Perry R, Miyake T, et al. Smad7 promotes and enhances skeletal muscle differentiation. Mol Cell Biol. 2006 Aug;26(16):6248–6260. PubMed PMID: 16880533; PubMed Central PMCID: PMC1592807.
- Miyake T, Alli N, McDermott JC. Nuclear function of Smad7 promotes myogenesis. Mol Cell Biol. 2010 Feb;30(3):722–735. PubMed PMID: 19995910; PubMed Central PMCID: PMC2812228.