ABSTRACT
Bisphenol A (BPA), an endocrine disrupting chemical (EDC), is a ubiquitous pollutant. As part of the Consortium Linking Academic and Regulatory Insights on BPA Toxicity (CLARITY-BPA), we sought to determine whether exposure of Sprague-Dawley rats to 2,500 μg/kg/day BPA (BPA) or 0.5 μg/kg/day ethinyl estradiol (EE) from gestational day 6 through postnatal day 21 induces behavior-relevant gene expression and DNA methylation changes in hippocampus and hypothalamus at adulthood. RNA and DNA were isolated from both regions. Expression of ten genes (Dnmt1, Dnmt3a, Dnmt3b, Esr1, Esr2, Avp, Ar, Oxt, Otr, and Bdnf) presumably altered by early-life BPA/EE exposure was examined. Three genes (Bdnf, Dnmt3b, and Esr1) were studied for DNA methylation changes in their putative 5ʹ promoter regions. Molecular changes in hippocampus were correlated to prior Barnes maze performance, including sniffing correct holes, distance traveled, and velocity. Exposure to BPA and/or EE disrupted patterns of sexually dimorphic gene expression/promoter DNA methylation observed in hippocampus and hypothalamus of controls. In the hippocampus of female offspring, BPA exposure resulted in hypermethylation of the putative 5ʹ promoter region of Bdnf, while EE exposure induced hypomethylation. Bdnf methylation was weakly associated with Bdnf expression in hippocampi of female rats. Hippocampal Bdnf expression in females showed a weak negative association with sniffing correct hole in Barnes maze. Hippocampal expression of Avp, Esr2, Oxt, and Otr was strongly associated with velocity of control rats in Barnes maze. Findings suggest BPA exposure induced non-EE-like gene expression and epigenetic changes in adult rat hippocampi, a region involved in spatial navigation.
Introduction
Many industrial chemicals are considered endocrine disrupting chemicals (EDCs) [Citation1], with bisphenol A (BPA) being one of the most prevalent [Citation2,Citation3]. In 2013, it was estimated to be produced at ~15 billion pounds annually [Citation4]. BPA has been detected in the urine of 93% of the US population [Citation5], suggesting widespread exposure of the human population to this chemical. The daily human exposure is estimated to be less than 1 μg BPA/kg body weight [Citation6]. The relative permanence and prevalence of BPA [Citation7] likely ensure continued exposure for many generations to come [Citation8].
Perinatal exposure to EDCs may detrimentally influence normal brain programming [Citation9–Citation11], as endogenous sex steroid hormones during this time serve as the primary drivers of brain sexual differentiation [Citation12–Citation14]. Developmental exposure of various rodent models to BPA has been reported to affect various behavioral domains, including socio-sexual, emotional, cognitive, spatial learning and memory, voluntary physical activity, parenting, and anxiety-like behaviors [Citation15–Citation26]. However, other studies suggest little to no effects of BPA on such behaviors [Citation27–Citation31].
To address these and other conflicting data in the area, the National Institute of Environmental Health Sciences (NIEHS)/National Toxicology Program (NTP)/Food and Drug Administration (FDA) Consortium Linking Academic and Regulatory Insights on BPA Toxicity (CLARITY-BPA) program was established [Citation32,Citation33]. As part of this project, which combines University and FDA/National Center for Toxicological Research (NCTR) scientists, several research groups across the country are addressing a wide-range of endpoints, including neurobehavioral assessment. In these studies, NCTR Sprague-Dawley rats were exposed daily during the developmental period to a wide dose range of BPA (2.5 µg/kg body weight (bw)/day, 25 µg/kg bw/day, or 2,500 µg/kg bw/day), a vehicle control (0.3% carboxymethylcellulose), or a reference estrogen (ethinyl estradiol, EE) control. Through this collaborative work, we previously reported that exposure of rats to BPA from gestation day (GD) 6 through postnatal day (PND) 21, but not EE, may disrupt aspects of spatial navigational learning and memory in females exposed to 2,500 μg BPA/kg bw/day [Citation26]. However, studies from another group suggested that BPA had minimal effects on anxiety-like behaviors or exploratory activity in the same animals [Citation34].
Spatial learning and memory is associated with hippocampal changes in plasticity, neurogenesis, neuronal cell volume and density, dendritic arborization, and long-term potentiation [Citation35,Citation36]. All of these processes are guided by sex steroid hormones [Citation37,Citation38]. Transcriptomic analyses of the hypothalamus from BPA- and EE-exposed California mice revealed that males and females developmentally exposed to BPA clustered separately from controls and EE-exposed individuals, with significantly greater gene expression changes in the males than females [Citation25]. In fact, BPA has been reported to induce effects independent of classic estrogen signaling [Citation8,Citation39].
Early exposure to BPA stimulates epigenetic and gene expression changes in the hippocampus and other brain sub-regions. Brain development and function is similar across taxa spanning from rodents and humans [Citation40,Citation41]. Previous rodent and zebrafish (Danio rerio) animal models and in vitro cell culture studies suggest BPA alters individual candidate genes in the hippocampus or hypothalamic regions or isolated neurons [Citation11,Citation25,Citation42–Citation47]. Some of the genes and their protein products implicated include estrogen receptor α (Esr1 and transcript variants), estrogen receptor β (Esr2), DNA methyltransferases (Dnmt1, 3a, 3b), androgen receptor (Ar), brain derived neural factor (Bdnf), vasopressin (Avp), and oxytocin receptor (Otr). As part of the CLARITY-BPA consortium, in utero exposure of NCTR Sprague-Dawley rats to BPA was shown to induce some gene expression changes in the hippocampus and hypothalamus at PND 1 [Citation48]. This study suggested that prenatal BPA exposure resulted in sex-specific effects on hypothalamic Esr1 and Esr2 and hippocampal and hypothalamic Oxt expression. However, it is not clear if such transcriptomic changes persist through adulthood. One mechanism by which BPA might alter gene expression patterns in the brain and other organs is through epigenetic reprogramming [Citation11,Citation47,Citation49], such as DNA methylation changes, that may last throughout life [Citation50,Citation51].
Based on these previous studies, we hypothesized that gestational and perinatal exposure to BPA would induce long-term DNA methylation and gene expression changes in the hypothalamus and hippocampus region, two brain regions suggested to be especially vulnerable to early exposure to BPA [Citation11,Citation44,Citation47]. Moreover, such biomolecular changes likely underpin the spatial learning and memory deficits identified in NCTR Sprague-Dawley female rats [Citation26]. In the current studies, candidate genes reported to be affected by BPA exposure in other rodent studies and that presumably mediate behaviors previously measured in these rats were examined in the hippocampus and hypothalamus of the same adult male and female rats tested in the behavioral studies [Citation26,Citation34].
The current study examined DNA methylation and gene expression pattern of genes, especially in the hippocampus, in male and female BPA- and EE-exposed Sprague-Dawley rats, which underwent previous behavioral testing in the Barnes maze. We hypothesized that exposure to BPA at the 2,500 µg/kg bw/day dose, where the most significant behavioral effects were detected in our previous study [Citation26], would induce long-term DNA methylation and gene expression changes in the hippocampus, which potentially underpin the spatial learning and memory deficits identified in the NCTR Sprague-Dawley female rats of the same cohort. As we were interested in examining these potential inter-relationships between bio-molecular and behavioral changes, we focused on the single dose of BPA that elicited phenotypic effects in the previous study [Citation26]. Accordingly, the two sets of results were then correlated to determine if epigenetic and gene expression changes in the hippocampus were associated with the previous behavioral performance. We also sought to examine gene expression and DNA methylation changes in the hypothalamus, as this brain region has been previously shown in Sprague-Dawley rats and other rodent models to be susceptible to early BPA exposure [Citation25,Citation48]. An ancillary objective was to determine whether BPA exposure induced unique DNA methylation and gene expression changes in these brain regions relative to EE, which would provide further evidence that this EDC can induce effects independent of the traditional estrogen signaling.
Results
Gene expression
Treatment differences
Targeted gene expression analysis by qPCR was performed in the hippocampus and hypothalamus of female and male rats with developmental exposure to 2,500 µg/kg bw/day BPA or 0.5 μg/kg bw/day ethinyl estradiol (EE). The panel of target genes, which included Dnmt3a, Dnmt3b, Dnmt1, Esr1, Esr2, Avp, Ar, Otr, Oxt, and Bdnf, have been reported to be regulated by BPA in the hippocampus and hypothalamus in several rodent and cell-based studies [Citation11,Citation25,Citation42–Citation47,Citation52–Citation59]. Expression of genes that were affected in females and/or males exposed to BPA or EE are shown in (hippocampus) and Supplemental Figures S1 and S2 (hypothalamus). Additional gene expression data are listed in (hippocampus) and Supplemental Table S1A and B (hypothalamus).
Table 1. (A) Other gene expression differences in the hippocampus of females across treatment groups. Data shown as mean, with lower and upper 95% confidence intervals shown in parenthesis. (B) Other gene expression differences in the hippocampus of males across treatment groups. Data shown as mean, with lower and upper 95% confidence intervals shown in parenthesis.
Figure 1. Gene expression differences in the hippocampus of female and male rats developmentally exposed to BPA or EE. (a) Avp expression in females. (b) Avp expression in males. (c) Otr expression in females. (d) Otr expression in males. P values are shown in the individual panels. Fold change refers to the gene expression level relative to that in the vehicle control group using the 2−ΔΔCt method. Statistical significance was determined using the ΔCt values. The graphs depict the mean ± upper and lower 95% confidence interval.
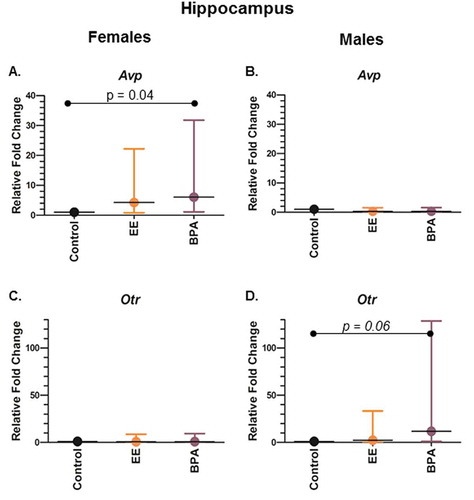
In the female hippocampus, Avp expression was significantly upregulated by 6-fold in the BPA group when compared to control (P = 0.041) ()). Changes in the expression level of the other 9 genes in the female hippocampus were insignificant when compared among groups (). In the female hypothalamus, Dnmt3b expression was significantly upregulated by 3.8-fold in the EE group when compared to control (P = 0.044) (Supplemental Figure S1A).
In the male hippocampus, there was a trend for Otr expression to be increased in the BPA group when compared to control (P = 0.060) ()). In the male hypothalamus, Dnmt3b was decreased by 12.5- and 5.6-fold, respectively, in BPA and EE group when compared to control (P = 0.011 and <0.001, respectively) (Supplemental Figure S1B). Dnmt1 expression was reduced by 5.6-fold in EE-exposed males when compared to controls (P = 0.010, Supplemental Figure S1D). Bdnf expression was downregulated in both BPA- and EE-treated males by 11.1- and 5.6-fold, respectively, (P = 0.001 and 0.014, respectively, Supplemental Figure S1F). Similarly, Esr1 expression was downregulated in BPA and EE-exposed males by 6.2- and 11-fold, respectively (P = 0.011 and 0.037, respectively, Supplemental Figure 2B). Yet, Esr2 expression was downregulated by 3.7-fold only in EE-exposed males (P = 0.028, Supplemental Figure 2C). Otr expression was reduced by 5-fold in BPA-exposed males (P = 0.015, Supplemental Figure 2F), and there was a trend for it to be decreased in EE-exposed (P = 0.054).
Figure 2. Effect of early-life exposure to EE and BPA on the methylation and expression of Bdnf in the adult rat hippocampus. (a) Schematic diagram showing the presence of a CG-rich region (green) at the promoter region of Bdnf, which is upstream of the transcription start site (TSS). Figure with annotation modified from UCSC Genome Browser. Region used for primer design (500 bp upstream and 100 bp downstream of the CG-rich region) is highlighted with a red line. (b) Primer design using MethPrimer. The blue highlighted region under the curve indicates the CG-rich region within the sequence used for primer design. A blue line indicates the target region for bisulfite sequencing. Methylation pattern of the putative 5ʹ promoter region of Bdnf in the hippocampus of (c) males and females, (d) females, and (e) males with early-life exposure to vehicle control (white), 0.5 μg EE/kg bw/day (EE; orange), and 2,500 μg BPA/kg bw/day (BPA; violet). Data expressed as mean ± SEM. Each circle represents the average % methylation of each corresponding CG site from 6–7 individual colonies (n = 5 males and n = 5 females per group). For methylation analysis, * P <0.05, *** P <0.001, and **** P <0.0001 vs. Vehicle by Two-way ANOVA and Tukey’s multiple test comparison. For gene expression analysis, *** P <0.001 vs. Vehicle by One-way ANOVA and Tukey test. Gene expression levels are expressed as gene expression level in treatment groups relative to the control group using the 2−ΔΔCt method.
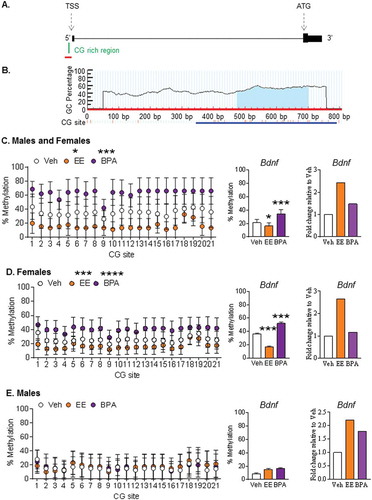
Sex differences within treatment
To determine whether the BPA or EE exposure induced sex differences in the expression of the target genes, the gene expression levels were compared between males and females within the same treatment group. In the hippocampus, Dnmt1, Avp, Ar, and Oxt expression significantly differed between control females and control males (P < 0.001 for Dnmt1, Avp, and Ar; P = 0.011 for Oxt) (). Dnmt1 was more abundant in control and BPA-treated females than their corresponding male counterparts (P < 0.001 for both), but this sex difference was absent in the EE-treated group. Avp was more expressed in control males than control females, but this relation was reversed in the BPA- and EE-exposed animals (P = 0.001 and 0.035, respectively). The expression of Oxt was higher in control females when compared to control males, while the opposite expression pattern was observed in the BPA group (P = 0.037). Developmental exposure to BPA or EE ablated the sexually dimorphic differences in Ar expression in the hippocampus observed in the control rats. In terms of estrogen receptors, Esr2 expression was lower in EE-treated females than EE-treated males (P = 0.038).
Table 2. Comparison of hippocampal gene expression differences between females and males within the same treatment group.
In the hypothalamus, all genes, except Avp and Oxt, showed sex-differences in the controls (P value range < 0.001 to 0.015), (Supplemental Table S2). However, developmental exposure to BPA or EE abolished many of these differences. For example, Esr1 expression was lower in control females than males (P = 0.002). Conversely, Esr1 expression was lower in males than females in the EE group (P = 0.015). The same sexually dimorphic pattern in Dnmt1 and Ar expression was observed in control and BPA groups (P < 0.001 and 0.030, respectively), with females in both groups having reduced expression of both transcripts relative to male counterparts.
DNA methylation
Based on the gene expression analyses and past-related studies, we sought to examine the DNA methylation patterns of Bdnf in the hippocampus and Dnmt3b and Esr1 in the hypothalamus. Since the availability of the rat hippocampal and hypothalamic samples were limited, an independent experiment using rat prostate cell lines was performed to verify whether the expression of Bdnf, Dnmt3b, and Esr1 are regulated by DNA methylation. In this in vitro study, NbE-1, a normal rat prostate epithelial cell line and AIT, a cancerous rat prostate epithelial cell line, were treated for eight days with 5-aza for gene expression analysis. As shown in Supplemental Figure 2A, treatment of 5-aza significantly increased the expression of Bdnf in NbE-1 cells in a dose-dependent manner. The level of Bdnf was very low to no expression in AIT cells, and the data were not shown. For Esr1, treatment of 5-aza significantly increased the expression of Esr1 by about 4-fold in AIT cells (Supplemental Figure S3B). The treatment did not affect Esr1 expression in NbE-1 cells. For Dnmt3b, treatment of 5-aza significantly increased the expression of Dnmt3b (>5-fold) in NbE-1 cells. The same treatment similarly increased the expression of Dnmt3b in AIT cells, although not significantly (Supplemental Figure S3C). Thus, our cell-based study supported that the expression of Bdnf, Esr, and Dnmt3b were likely to be regulated by DNA methylation.
To compare methylation patterns of the putative 5ʹ promoter region of Bdnf, Esr1, and Dnmt3b in the female and male hippocampus (for Bdnf) or hypothalamus (for Esr1 and Dnmt3b), bisulfite sequencing analysis was performed on these brain tissues. The putative 5ʹ promoter region of Bdnf was hypomethylated in the hippocampus of EE-exposed animals, but it was hypermethylated in the BPA-exposed animals, when compared to that in the vehicle control ()). When the data were sorted by sex, a similar pattern was observed in the females. Interestingly, the putative 5ʹ promoter region of Bdnf was hypomethylated in the EE-exposed females, but it was hypermethylated in the BPA-exposed females when compared to that in the vehicle-exposed females (P <0.0001 for both comparisons) ()). Conversely, there were no treatment differences in DNA methylation pattern of the putative 5ʹ promoter region of Bdnf in males ()).
For Dnmt3b, there were no differences in the overall methylation patterns for the CG-rich region in its putative 5ʹ promoter region when comparing both sexes combined or separately among treatment groups (Supplemental Figure S4C-E). Yet, there were site-specific changes when compared among treatment groups in the males (Supplemental Figure S4E). Similar to Dnmt3b, there were no differences in the overall methylation patterns for Esr1 when comparing among treatment groups in either and both sexes. Yet, significant site-specific differences were observed when compared among treatment groups for all animals, within females, and within males (Supplemental Figure 5C-E).
Correlation analyses
Using our gene expression and methylation data, we sought to determine whether the identified gene expression and DNA methylation changes associated with previously identified behavioral changes observed in the same group of rats tested for Barnes maze performance. Since the Barnes maze performance measures spatial learning and memory, correlation between transcript levels in the hippocampus, an area associated with spatial learning and memory [Citation35,Citation36,Citation60–Citation62], and the methylation pattern for Bdnf in this same brain region were examined. Additionally, correlation analyses were performed for the percentage methylation of the putative 5ʹ promoter region of Bdnf and the expression of Bdnf in the hippocampus. There was an overall weak negative association observed for Bdnf DNA methylation and gene expression (r = −0.35, P = 0.07) ()). When considering only the females, which displayed significant behavioral changes in our previous study, there was a trend for a negative association between Bdnf expression and rate of sniffing the correct hole in the Barnes maze (r = −0.48, P = 0.07) ()). This association was not observed when correlating Bdnf expression with velocity or distance travelled in Barnes maze, and was absent in males (data not shown).
Figure 3. Correlation analyses for hippocampal Bdnf gene expression. (a) Overall correlation between the expression level of Bdnf and % promoter methylation of Bdnf in rats with all data points included. (b) Overall correlation between the expression level of Bdnf and the rate of sniffing the correct hole in Barnes maze [Citation26] in female rats. Gene expression levels are expressed in log 2 values and as Ct value of Rpl19 relative to that of the target gene. Percentage methylation of the putative 5ʹ end region of Bdnf is in log value. P <0.05 was considered as statistically significant. Only samples with a detectable Ct value were used in the correlation analysis.
![Figure 3. Correlation analyses for hippocampal Bdnf gene expression. (a) Overall correlation between the expression level of Bdnf and % promoter methylation of Bdnf in rats with all data points included. (b) Overall correlation between the expression level of Bdnf and the rate of sniffing the correct hole in Barnes maze [Citation26] in female rats. Gene expression levels are expressed in log 2 values and as Ct value of Rpl19 relative to that of the target gene. Percentage methylation of the putative 5ʹ end region of Bdnf is in log value. P <0.05 was considered as statistically significant. Only samples with a detectable Ct value were used in the correlation analysis.](/cms/asset/a950bc05-89a9-4bd4-9475-b1a89fa82d3b/kepi_a_1497388_f0003_oc.jpg)
For the other transcripts in the hippocampus of vehicle only individuals, the expression levels of five genes (Avp, Oxt, Otr, Esr2, and Dnmt3b) were associated with velocity in the Barnes maze. Both expression of Avp and Oxt negatively associated with increased velocity (Avp: r = −0.84, P = 0.002; Oxt: r = −0.70, P = 0.02) (). In contrast, expression of Otr positively correlated with this behavioral category (r = 0.88, P = 0.05) (). A positive association with Esr2 expression and velocity was also detected, although not significant (r = 0.69, P = 0.06) (). Dnmt3b expression in the vehicle group was negatively associated with sniffing correct holes, but not velocity, in the Barnes maze (r = −0.76, P = 0.01; data not shown). Of note, all of these associations were absent in the EE- and BPA-exposed groups (data not shown).
Figure 4. Correlation between velocity in the Barnes maze and hippocampal expression levels of (a) Avp, (b) Esr2, (c) Oxt, and (d) Otr in control rats. Gene expression levels are expressed in log 2 values and as Ct value of Rpl19 relative to that of the target gene. Percentage methylation of the putative 5ʹ end region of Bdnf is in log value. Velocity is expressed as mean from seven observations and was previously reported in [Citation26]. P <0.05 was considered as statistically significant. Only samples with a detectable Ct value were used in the correlation analysis.
![Figure 4. Correlation between velocity in the Barnes maze and hippocampal expression levels of (a) Avp, (b) Esr2, (c) Oxt, and (d) Otr in control rats. Gene expression levels are expressed in log 2 values and as Ct value of Rpl19 relative to that of the target gene. Percentage methylation of the putative 5ʹ end region of Bdnf is in log value. Velocity is expressed as mean from seven observations and was previously reported in [Citation26]. P <0.05 was considered as statistically significant. Only samples with a detectable Ct value were used in the correlation analysis.](/cms/asset/96e317c1-090a-453d-af21-9ae01e2b45c1/kepi_a_1497388_f0004_oc.jpg)
Discussion
The primary goal of this current study was to examine whether perinatal exposure to BPA and EE could result in persistent gene expression and DNA methylation changes in the hippocampus and hypothalamus of Sprague-Dawley rats. An ancillary goal was to assess whether such biomolecular alterations in the hippocampus correlate with previously described spatial navigational learning and memory behaviors in these same male and female rats [Citation26]. BPA and EE exposure resulted in sex-dependent gene expression changes in the hippocampus and hypothalamus, as indicated in and Supplemental Table S2. Dnmt3b expression was upregulated in the hypothalamus of EE females, whereas Avp expression was increased in the hippocampus of BPA-exposed females. Dnmt3b expression was reduced in the hypothalamus of BPA- and EE-exposed males relative to controls. Dnmt1 was only downregulated in the hypothalamus of EE-exposed males. Esr1 was suppressed in the hypothalamus of BPA and EE-exposed males, whereas Esr2 was only downregulated in this brain region for EE-exposed males. Bdnf was decreased in the hypothalamus of EE- and BPA-exposed males.
Gene expression changes in similar numbers of PND 1 Sprague-Dawley male and female rats were examined as part of an earlier CLARITY-BPA Consortium Study and identified sex-specific gene expression changes in the hippocampus and hypothalamus [Citation48]. While this previous study used both RNAseq to identify global transcriptomic changes and qPCR to validate candidate genes, interestingly, none of the gene expression changes identified in these two brain regions at PND 1 were detected in the current studies with adult brain samples. For example, Esr2 and Oxt expression was reported to be increased in the hippocampus of males exposed to 25,000 µg BPA/kg bw/day. In the hypothalamus, Esr1 and Esr2 expression were increased in females exposed to 25,000 µg BPA/kg bw/day, whereas Oxt was increased in this brain region for females exposed to BPA at doses of 2.5 and 250 µg/kg bw/day and males exposed to 25 µg BPA/kg bw/day [Citation48]. Thus, the current results suggest that some gene expression changes identified at PND 1 do not persist until adulthood. However, other gene expression changes identified in this earlier study were evident in both brain regions in adult males and females exposed to BPA or EE. These seemingly conflicting results are not surprising as the rodent brain continues to undergo considerable programming during the postnatal period. Strong conservation exists in brain development and function across species spanning from rodents to humans [Citation40,Citation41]. Yet, there are key embryological milestone differences for distinct brain regions between rodents and humans [Citation40,Citation41]. For several brain regions, including the cerebrum, hippocampus, hypothalamus, and amygdala, the early postnatal developmental period in most rodent species is approximately equivalent to the third trimester in human fetal development [Citation40,Citation41]. Correspondingly, the longer exposure period in this study relative to the previous study that only examined up through PND 1 ensured exposure throughout brain development in rats. Thus, our findings suggest that the developmental period of the brain may be a window of susceptibility to affect spatial navigational ability later in life.
To determine whether developmental exposure to BPA or EE results in DNA methylation changes, methylation pattern of Bdnf was examined in the hippocampus and that of Dnmt3b and Esr1 was examined in the hypothalamus. EE-exposure induced Bdnf promoter hypomethylation in females. Conversely, BPA exposure in females resulted in hypermethylation of this gene promoter. While there were no overall differences in DNA methylation for Dnmt3b and Esr1 in the hypothalamus in the BPA or EE treated groups, there were site-specific methylation changes that could be biologically relevant. Other studies have shown that BPA exposure can result in DNA methylation changes in the brain and other organs/cell lines [Citation49,Citation63–Citation70]. DNA methylation status of the Bdnf promoter has been proposed to be a potential biomarker of BPA exposure. Specifically, developmental exposure of BALB/c mice to BPA (200 μg/kg/day through the dam from GD 0–19) resulted in hypermethylation of the Bdnf promoter in the hippocampus of males, but not females [Citation47]. In our study, DNA hypermethylation of Bdnf was identified in the hippocampus of BPA-exposed females, but not males. However, Bdnf expression was reduced in the hypothalamus of BPA- and EE-exposed males. Similarly, early life exposure of a/a C57BL/6J mice 50 μg BPA/kg in the maternal diet through gestation and lactation increased overall methylation of Esr1 in tail tissue [Citation70]. Yet, this methylation change was not observed in our current study. Discordant results between this previous study and the current results might be due to the dose (200 μg/kg/day vs. 2,500 μg/kg/day), the window of exposure (gestational vs. gestational and perinatal), and rodent model (BALB/c mice vs. Sprague-Dawley rats) of BPA exposure, and the type of target tissues (tail vs. hippocampus) used for molecular analyses.
The last objective of the current study was to determine whether hippocampal gene expression and DNA methylation changes in Bdnf correlate with various behavioral parameters assessed in our previous study when rats were tested in the Barnes maze [Citation26]. These parameters included latency to locate the correct escape hole, sniffing correct and incorrect holes, distance traveled, and velocity. There was an overall weak negative association between DNA methylation and gene expression of Bdnf in the adult rat hippocampus. Bdnf expression in females also showed a weak negative correlation with sniffing the correct hole in the Barnes maze. Object recognition memory in male Sprague-Dawley rats is impaired when dams are treated during 9–20 days of gestation with varying doses of BPA (0.05 to 50 mg/kg/day), and these behavioral changes are associated with BPA-inhibition of phosphorylation of CREB and BDNF in the hippocampus [Citation71]. While the expression of other genes in the hippocampus, such as Avp, Oxt, Otr, and Dnmt3b, were associated with various measurements in this behavioral test, such associations were absent in the BPA- and EE-exposed groups. This finding may suggest that exposure to one of these EDCs disrupts the normal neural pathways that regulate select behaviors. We previously determined that developmental exposure to BPA or EE in painted turtles resulted in significant gene expression changes, especially those associated with mitochondrial and ribosomal protein functions, that correlated with prior performance when tested in an aquatic spatial maze [Citation72]. While such analyses cannot establish causation, they suggested a list of candidate genes that may be vulnerable to early life exposure to BPA or EE and contribute to behavioral changes later-in-life. Future investigations, which utilize transgenic technology (such as CRISPR/Cas9), may validate whether these candidate genes are indeed mediating the neuroendocrine disruptive effects of BPA or EE.
In conclusion, the current CLARITY-BPA Consortium studies reveal that early life exposure to BPA and EE can result in select gene expression changes in the hippocampus and hypothalamus of adult Sprague-Dawley rats. Additionally, exposure to BPA or EE abolished or reversed sexually dimorphic expression pattern differences detected in the hippocampus and hypothalamus of control rats. Exposure to both BPA and EE can also result in DNA methylation changes in the hippocampus and hypothalamus. These gene expression and DNA methylation changes in the hippocampus, especially those of Bdnf, showed some correlations with the performance of females in the Barnes maze. These gene expression and DNA methylation changes, especially for Bdnf, may provide useful biomarkers of EDC exposure in mammals.
Materials and methods
Animal husbandry and dosing
Comprehensive details on animal husbandry, treatment, and dosing procedures have been published [Citation73]. Therefore, only brief details are described here. All animal use and procedures were approved in advance by the NCTR Institutional Animal Care and Use Committee and were conducted in an Association for Assessment and Accreditation of Laboratory Animal Care (AALAC)-accredited facility. Experiments were performed in accordance with the ‘Guide for the Care and Use of Laboratory Animals’ [Citation74]. The animal rooms for breeding, gestation, and pre- weaning housing were maintained at 23 ± 3°C with a humidity level of 50 ± 20%, and a 12 hour:12 hour light/dark cycle with lights on at 6:00. After weaning on postnatal day (PND) 21, the light cycle was changed to a reverse cycle (light on at 11:00; off at 23:00). A low phytoestrogen diet (5K96 verified casein diet 10 IF, round pellets, γ-irradiated, Test Diets, Purina Mills, Richmond, IN), and Millipore-filtered water in glass water bottles were provided ad libitum. Housing cages were polysulfone with microisolator tops (Ancare Corp., Bellmore, NY) and contained hardwood chip bedding. Diet was tested for BPA, genistein, daidzein, zearalenone, and coumesterol, and drinking water, cage, and bedding extracts were tested for BPA. All lots of diet had BPA levels below 5 ppb and none of the other materials tests had levels of BPA detectable above the level of the average analytical blanks. Breeder male and female Sprague-Dawley (SD) rats (i.e., F0) from the NCTR breeding colony were placed in study housing conditions at weaning on PND 21.
Approximately two weeks prior to mating, females were assigned to one of three treatment groups [vehicle control, 2,500 μg/kg bw/day BPA (BPA), or 0.5 μg/kg bw/day ethinyl estradiol (EE)] based on body weight ranking to produce approximately equal mean body weights in each group. Males were randomly mated with females with the stipulation that no sibling or first cousin pairing was permitted. Breeding occurred in five ‘loads’ or ‘cohorts’, each spaced four weeks apart. Offspring from the last two breedings (i.e., loads 4 and 5) were used for the behavioral studies reported previously [Citation26,Citation34] and for the molecular assessments described here.
While internal concentrations of BPA were not measured in the current studies, they were assessed previously in a comparable study that tested the effects of BPA in the same animal model, dosing regimen (i.e., daily oral gavage of dams from GD 6 through start of parturition, and direct dosing of pups from PND 1), and similar dosing formulations (0.3% CMC as vehicle; BPA and EE doses in this previous study approached or matched those included in the current CLARITY-BPA study) [Citation75]. Based on this prior study, the predicted maximum circulating levels of aglycone BPA and EE for rats tested in the current study would be: BPA: ~200 nM at PND 4 and ~20 nM at PND 21 and EE: ~300 pM at PND 4 and ~20 pM at PND 21. The lower levels of aglycone BPA in older animals are due to maturation of the metabolic capacity that resulted in more efficient conjugation of BPA and EE at PND 21 than PND 4 [Citation76]. Since the dosing was stopped at PND 21, the levels of BPA or EE at the time of these molecular assessments at adulthood would be undetectable.
Dams were considered pregnant when a sperm plug or a sperm-positive vaginal cytology was observed [mating day = gestational day (GD 0)]. Beginning on GD 6, dams were dosed by daily gavage with 0.3% carboxymethylcellulose (CMC or vehicle), 2.5 µg BPA/kg bw/day, 25 µg BPA/kg bw/day, 2,500 µg BPA/kg bw/day, or 0.5 µg EE/kg bw/day. These BPA doses were selected to provide low, middle, and upper levels of exposure and are below the no-observed-adverse-effect level (NOAEL) of 5 mg/kg bw/day as detailed in [Citation77,Citation78]. These doses are also within the dose range used in prior studies conducted at the NCTR with the same animal model [Citation26,Citation34,Citation55]. In particular, our previous study reported that behavioral differences were observed only among offspring with exposure to the dose of 2,500 µg BPA/kg bw/day [Citation26]. Thus, we focused on this BPA dose in the current study. The materials used for dose formulation were BPA [CAS # 80–05–7, TCI America, Portland, OR; catalogue # B0494, Lot # 111909/AOHOK (air-milled), ≥99.9 purity], EE (CAS # 57-63-6, Sigma-Aldrich, St. Louis, MO; catalogue # E4876, Lot # 071M1492V, >99% purity), and CMC (Sigma-Aldrich; catalogue # C5013, Lot # 041M0105V). Dams were dosed by daily gavage at a volume of 5 ml/kg bw using a modified Hamilton Microlab® ML511C programmable 115V pump (Hamilton Co., Reno, NV; Lewis et al., 2010). No treatment occurred on the day of parturition, typically GD 22. On the day after birth (i.e., PND 1), litters were randomly culled to 5/sex where possible and identified with a distinguishable paw tattoo. Only offspring from litters born between GD 20–26 and containing at least 3/sex, a minimum of 9 live pups, and with a difference of number of born male and females pups not greater than 5 were used in the current study. Beginning on PND 1 and continuing daily through weaning on PND 21, all offspring/litter were weighed and dosed by daily gavage with the same dose their dam had received. Twelve animals/sex/treatment group were assigned to the current study (1/sex/litter). Weaned animals were housed 2 to 3 per cage; cage-mates were from the same treatment, sex, and age, but were not siblings as only 1/sex/litter was used. When no cage-mate was available, a ‘companion’ animal was used. ‘Companion’ animals were from the same strain, sex, and age as the study animals, but were treatment naïve and were not tested.
Brain collection and processing
Upon completion of the behavior assessments, animals were slightly sedated with carbon dioxide and exsanguinated by cardiac puncture. Males were sacrificed on PND 101 (for ½ of each cohort) or PND 115 (for the remaining ½) and females were sacrificed in estrus between PND 101–107 (for ½ of each cohort) or PND 115–119 (for the remaining ½). Brains were removed from the cranium and rapidly frozen in dry ice, with great care to maintain their morphology. The frozen brains were stored at −80ºC until being shipped on dry ice to the Rosenfeld laboratory at the University of Missouri for further processing. The hippocampal and hypothalamic regions were micro-punched on dry ice similarly to PND 1 rat sections detailed in [Citation48] with the exceptions detailed below, and a rat brain atlas [Citation79] served as a guide for the two brain regions. For the hypothalamus, a Harris Micro-Punch 2mm in diameter and 1mm in depth (Catalogue # 15093, Ted Pella, Redding, CA, USA) was used to obtain 2 sequential punches that spanned the rostral to caudal medial regions of this brain region. For the hippocampus, a Harris Micro-Punch 1mm in diameter and 1mm in depth (Catalogue # 15091, Ted Pella) was used to obtain two punches that spanned the rostro-dorsal to caudo-ventral regions and thereby sample from both the CA1 and CA3 nuclei. All tissues were coded so that the data collection was done blinded to treatment level. Raw data were deposited into the NIEHS Chemical Effects in Biological Systems (CEBS) databank. The initial gene expression data were collected blinded to treatment group, but the identity of the samples was decoded to design the follow-up experiments, including the DNA methylation analysis. For these studies, males and females in the BPA 2,500, EE, and vehicle control groups were examined. The BPA 2,500 male and female groups were selected for this current study as it was previously determined that females in this group showed the most significant behavioral changes when tested in the Barnes maze [Citation26]. Five replicates for each brain region, sex, and group were tested in the current study, as this number of replicates was tested in the previous CLARITY-BPA rat study that showed gene expression differences the hippocampus and hypothalamus at PND 1 [Citation48] and in the hypothalamus of California mice (Peromyscus californicus) [Citation25].
Gene expression analysis
DNA and total RNA were isolated using the AllPrep DNA/RNA/miRNA Universal Kit (Catalogue #80224; Qiagen, Hilden, Germany). RNA was quantified using a spectrophotometer (Nanodrop 2000, ThermoFisher Scientific). DNA and RNA extracted from rat hypothalamus and hippocampus (thirty samples per brain sub-region, including five males and five females per dose group) was used for the gene expression analyses by quantitative real-time polymerase chain reaction (qPCR). Total RNA was reverse transcribed to complementary DNA (cDNA) with SuperScript® III Reverse Transcriptase (ThermoFisher Scientific, Waltham, MA, USA), which included a DNase treatment step. The QuantiTect SYBR® Green PCR kit (Qiagen) was used for the qPCR reactions, which were performed with a 7500 Realtime PCR system (ThermoFisher Scientific). The sequences of the primers used in the qPCR analyses are listed in Supplemental Table S3. The nucleotide sequence of the test genes was imported into the free Primer 3 (version 4.0.0, http://primer3.ut.ee/) software to create the primers listed in Supplemental Table S3. Primers were ordered from Integrated DNA Technologies (IDT, Coralville, IA). All primer sequences were validated beforehand to ensure that only a single product was obtained and the amplicon was the correct size. We also validated the primers based on obtained dissociation curves. For gene expression analysis, the same amount of cDNA (47.6 ng) was used per qPCR reaction and the expression levels of the target genes were normalized against that of an internal reference gene (Rpl19). Each sample was run in duplicate and a no template control was included in each assay; the latter showed no amplification in all cases. The cycling conditions were: 50⁰C(2 minutes) + 95⁰C(15 minutes) + [94⁰C (15 seconds)+ 56⁰C(35 seconds)+ 72⁰C(36 seconds)] X 40 cycles.
Treatment of NbE-1 and AIT cells with 5-aza-2-deoxycytidine
To validate that the expression of select genes are regulated by DNA methylation, a cell-based analysis was performed. Briefly, an immortalized normal rat prostatic epithelial cell line NbE-1 [Citation80] and a tumorigenic prostate epithelial cell line AIT [Citation81] were treated with 0.1% dimethylsulfoxide (DMSO; Sigma) as vehicle control, 0.5 µM or 1.0 µM 5-aza-2-deoxycytidine (5-aza; Tocris), a DNA methylation inhibitor, every two days for eight days as previously described [Citation50,Citation82]. RNA was extracted from the cell lysates using TRIzol reagent (Invitrogen-ThermoFisher Scientific). Total RNA (1 μg) was DNase treated (Promega) and reversed transcribed for qPCR analysis as described in [Citation66].
Bisulfite sequencing analysis
Ten matched rat hypothalamus and hippocampus samples, including five males and five females per treatment group, were used for bisulfite sequencing analysis. These samples were collected from the same animals that were selected for gene expression analyses. In brief, three hundred ng of genomic DNA was bisulfite converted using EZ DNA methylation kit (ZymoResearch) according to the manufacturer’s protocol as described previously [Citation66]. Bisulfite polymerase chain reaction was performed using 3 μL of the bisulfite converted DNA, Platinum Taq DNA polymerase (Invitrogen), and gene specific primers in a 20 μL reaction volume based on the manufacturer’s protocol. Gene-specific primers for bisulfite sequencing were designed using MethPrimer (http://www.urogene.org/methprimer/) with reference to the predicted CpG island as indicated in UCSC Genome Browser (http://wwgenome/ucsc.edu/), and are tabulated in Supplemental Table S3. The size and location of the amplicon generated by each primer-pair in the specific gene (Bdnf, ; Dnmt3b, Supplemental Figure S4; and Esr1, Supplemental Figure S5) were illustrated (see Results section). The amplicons were resolved in an agarose gel, purified using GenElute Gel Extraction Kit (Sigma), and cloned into pGEM-T easy vector (Promega) according to the manufacturer’s protocol. Recombinant plasmids formed from a single One Shot Top 10 E coli colony (~5–7 colonies per sample) were selected and amplified using TempliPhi DNA amplification kit (GE Healthcare) and sequenced by Macrogen USA (Macrogen Maryland). The methylation status of each CpG site was analyzed using Quantification tool for Methylation Analysis (http://quma.cdb.riken.jp/).
Statistical analysis
Numerical outcome measures are expressed as mean ± SEM. Since some male/female pairs were siblings (Supplemental Table S4), a random effect was added in the statistical models to account for between sibling correlation or within cluster effect and ensure more precise and robust estimates of means and SEMs. Target gene expression was adjusted for internal control gene Rpl19. Expression levels in the treatment group relative to that in the vehicle control group were calculated using the 2−ΔΔCt method [Citation83]. For samples with the expression level of an individual gene being undetectable by qPCR, these samples were excluded in the gene-specific expression and correlation analyses. Out of 30 hypothalamic samples, one male sample was excluded from the EE group in the expression level analyses for Esr1. For the hippocampus, the following samples were excluded in the expression analyses for genes Esr2, Esr1, and Otr: (1) two females in the vehicle group for Esr2, (2) two females in the vehicle group and one male and one female in the BPA group for Esr1, and (3) three females and two males in the vehicle group, one female and three males in the EE group, and two males in the BPA group for Otr. Since the cycle threshold values for the endogenous Rpl19 of the excluded samples were comparable to the other samples, they were included in the expression analyses for the other genes (Dnmt1, Dnmt3a, Dnmt3b, Avp, Ar, Oxt, and Bdnf).
All numerical variables were inspected for central tendency, dispersion, skewness, and outliers before performing formal statistical analyses. Since all variables showed no concerns of severe skewness of the distributions, parametric statistical models where the variables were not transformed were employed. The box plots of the variables did show some mild outliers (i.e., the outliers were above or below 1.5IQR of upper and lower quartiles, but within 3IQR of the upper and lower quartiles, IQR = inter quartile range). Hence, for each variable, two analyses were conducted with and without removing outliers to test the sensitivity of the findings to the outliers. Since all results showed no changes of conclusion using either method, only the results from full data (hence with outliers) are presented in the current work.
For each numerical variable at each brain region, its association to the fixed effect of group (vehicle vs. BPA vs. EE) was assessed using a mixed effect model. Since some litters had more than one offspring represented in the current study, which is considered a random effect, these litters were used to account for within litter (or between sibling) correlation. In addition, sex was used to adjust for the association of interest in the model. From each mixed effect model, post hoc means of the numerical dependent variable were estimated and compared between groups. Each random effect model was also compared to an alternative model without considering the within litter correlation. The results from the alternative methods showed agreements to the mixed effect models in post hoc means and standard errors for each group, and thus, the results from the mixed effect models were used in the current study. All statistical tests were performed using SAS 9.4 software (SAS, Cary, NC). P values <0.05 were considered statistically significant. Bisulfite sequencing analyses was performed on ~5-7 individual colonies per sample, with five samples per sex per group. Two-way ANOVA and Tukey’s multiple comparison tests were used to compare among treatment groups. P <0.05 was considered as statistically significant.
Correlation analyses
By using Spearman correlation analyses, the gene expression (based on log 2 ΔCt values to permit inclusion of the vehicle control group in these assessments) and DNA methylation data (expressed in log) were correlated to each other and the previously reported spatial learning and memory results from the same rats [Citation26].
Disclaimer
The contents of this manuscript should not be interpreted as current or future policy of the FDA or NIEHS. The mention of any manufacturers or trade names is only for clarity and does not constitute endorsement.
Supplemental Material
Download MS Word (961.5 KB)Acknowledgments
The authors are grateful for Drs. K. Barry Delclos, Sherry A. Ferguson, and colleagues at National Center for Toxicological Research/Food and Drug Administration for their assistance in designing and collecting the brains as part of this CLARITY-BPA consortium project. The authors also acknowledge Dr. Thaddeus Schug and Dr. Retha Newbold of NIEHS for their assistance in coordinating and addressing any questions and concerns that arose during the project. The authors would also like to thank Ms. Natalie Ho for her assistance in designing Bdnf primers for bisulfite sequencing.
Disclosure statement
No potential conflict of interest was reported by the authors.
SUPPLEMENTAL DATA
Supplemental data for this article can be accessed here.
Additional information
Funding
References
- Diamanti-Kandarakis E, Bourguignon JP, Giudice LC, et al. Endocrine-disrupting chemicals: an Endocrine Society scientific statement. Endocr Rev 2009; 30:293–342.
- Galloway T, Cipelli R, Guralnick J, et al. Daily bisphenol A excretion and associations with sex hormone concentrations: results from the InCHIANTI adult population study. Environ Health Perspect 2010; 118:1603–1608.
- He Y, Miao M, Herrinton LJ, et al. Bisphenol A levels in blood and urine in a Chinese population and the personal factors affecting the levels. Environ Res 2009; 109:629–633.
- GrandViewResearch. Global bisphenol A (BPA) market by appliation (appliances, automotive, consumer, construction, electrical & electronics) expected to reach USD 20.03 billion by 2020. 2014. Available from: http://www.digitaljournal.com/pr/2009287.
- Calafat AM, Ye X, Wong LY, et al. Exposure of the U.S. population to bisphenol A and 4-tertiary-octylphenol: 2003-2004. Environ Health Perspect 2008; 116:39–44.
- Toxicological and Health Aspects of Bisphenol A. Report of Joint FAO/WHO Expert Meeting. Report of Stakeholder Meeting on Bisphenol A. November 2010. Ottawa, Canada. Available from: http://apps.who.int/iris/bitstream/10665/44624/1/97892141564274_eng.pdf.
- Environment Canada. Screening Assessment for the Challenge Phenol, 4,4ʹ -(1-methylethylidene)bis-(Bisphenol A) Chemical Abstracts Service Registry Number 80-05-7. Ministers of the Environment and of Health, ed., 2008:1–107. Ottawa, Canada.
- Vandenberg LN, Maffini MV, Sonnenschein C, et al. Bisphenol-A and the great divide: a review of controversies in the field of endocrine disruption. Endocr Rev 2009; 30:75–95.
- Palanza P, Morellini F, Parmigiani S, et al. Prenatal exposure to endocrine disrupting chemicals: effects on behavioral development. Neurosci Biobehav Rev 1999; 23:1011–1027.
- Jasarevic E, Geary D, Rosenfeld C Sexually selected traits: a fundamental framework for studies on behavioral epigenetics. ILAR J 2012; 53:253–269.
- Kundakovic M, Gudsnuk K, Franks B, et al. Sex-specific epigenetic disruption and behavioral changes following low-dose in utero bisphenol A exposure. Proc Natl Acad Sci U S A 2013; 110:9956–9961.
- Arnold AP, Breedlove SM Organizational and activational effects of sex steroids on brain and behavior: a reanalysis. Horm Behav 1985; 19:469–498.
- Adkins-Regan E Hormones and animal social behavior. Princeton (NJ): Princeton University Press, 2005.
- McCarthy MM Estradiol and the developing brain. Physiol Rev 2008; 88:91–124.
- Mueller SC, Temple V, Oh E, et al. Early androgen exposure modulates spatial cognition in congenital adrenal hyperplasia (CAH). Psychoneuroendocrinology 2008; 33:973–980.
- Puts DA, McDaniel MA, Jordan CL, et al. Spatial ability and prenatal androgens: meta-analyses of congenital adrenal hyperplasia and digit ratio (2D:4D) studies. Arch Sex Behav 2008; 37:100–111.
- Berenbaum S, Hines M Early androgens are related to childhood sex-typed toy preferences. Psychol Sci 1992; 3:203–206.
- Geary DC Male, female: the evolution of human sex differences. Chapter 10. 291–294. Washington (DC): American Psychological Association, 2010.
- Rosenfeld CS Bisphenol A and phthalate endocrine disruption of parental and social behaviors. Front Neurosci 2015; 9:1–15.
- Jasarevic E, Sieli PT, Twellman EE, et al. Disruption of adult expression of sexually selected traits by developmental exposure to bisphenol A. Proc Natl Acad Sci U S A 2011; 108:11715–11720.
- Williams SA, Jasarevic E, Vandas GM, et al. Effects of developmental bisphenol A exposure on reproductive-related behaviors in California mice (Peromyscus californicus): a monogamous animal model. PLoS ONE 2013; 8:e55698.
- Johnson SA, Painter MS, Javurek AB, et al. Sex-dependent effects of developmental exposure to bisphenol A and ethinyl estradiol on metabolic parameters and voluntary physical activity. J Dev Orig Health Dis 2015; 6:539–552.
- Johnson SA, Javurek AB, Painter MS, et al. Disruption of parenting behaviors in California Mice, a monogamous rodent species, by endocrine disrupting chemicals. PLoS One 2015; 10:e0126284.
- Jasarevic E, Williams SA, Vandas GM, et al. Sex and dose-dependent effects of developmental exposure to bisphenol A on anxiety and spatial learning in deer mice (Peromyscus maniculatus bairdii) offspring. Horm Behav 2013; 63:180–189.
- Johnson SA, Spollen WG, Manshack LK, et al. Hypothalamic transcriptomic alterations in male and female California mice (Peromyscus californicus) developmentally exposed to bisphenol A or ethinyl estradiol. Physiol rep 2017;5:pii: e13133.
- Johnson SA, Javurek AB, Painter MS, et al., Effects of developmental exposure to bisphenol A on spatial navigational learning and memory in rats: a CLARITY-BPA study. Horm Behav 2016; 80:139–148.
- Kuwahara R, Kawaguchi S, Kohara Y, et al. Bisphenol A does not affect memory performance in adult male rats. Cell Mol Neurobiol 2014; 34:333–342.
- Neese SL, Bandara SB, Schantz SL Working memory in bisphenol-A treated middle-aged ovariectomized rats. Neurotoxicol Teratol 2013; 35:46–53.
- Sadowski RN, Park P, Neese SL, et al. Effects of perinatal bisphenol A exposure during early development on radial arm maze behavior in adult male and female rats. Neurotoxicol Teratol 2014; 42:17–24.
- Ferguson SA, Law CD, Abshire JS Developmental treatment with bisphenol A causes few alterations on measures of postweaning activity and learning. Neurotoxicol Teratol 2012; 34:598–606.
- Ryan BC, Vandenbergh JG Developmental exposure to environmental estrogens alters anxiety and spatial memory in female mice. Horm Behav 2006; 50:85–93.
- Schug TT, Heindel JJ, Camacho L, et al. A new approach to synergize academic and guideline-compliant research: the CLARITY-BPA research program. Reprod Toxicol 2013; 40:35–40.
- Birnbaum LS, Bucher JR, Collman GW, et al. Consortium-based science: the NIEHS’s multipronged, collaborative approach to assessing the health effects of bisphenol A. Environ Health Perspect 2012; 120:1640–1644.
- Rebuli ME, Camacho L, Adonay ME, et al. Impact of Low-Dose Oral Exposure to Bisphenol A (BPA) on Juvenile and Adult Rat Exploratory and Anxiety Behavior: a CLARITY-BPA Consortium Study. Toxicol Sci 2015; 148:341–354.
- Galea LAM, McEwen BS Sex and seasonal differences in the rate of cell proliferation in the dentate gyrus of adult wild meadow voles. Neuroscience 1999; 89:955–964.
- Pyter LM, Reader BF, Nelson RJ Short photoperiods impair spatial learning and alter hippocampal dendritic morphology in adult male white-footed mice (Peromyscus leucopus). J Neurosci 2005; 25:4521–4526.
- Duarte-Guterman P, Yagi S, Chow C, et al. Hippocampal learning, memory, and neurogenesis: effects of sex and estrogens across the lifespan in adults. Horm Behav 2015; 74:37–52.
- Tuscher JJ, Fortress AM, Kim J, et al. Regulation of object recognition and object placement by ovarian sex steroid hormones. Behav Brain Res 2015; 285:140–157.
- Vandenberg LN, Colborn T, Hayes TB, et al., Hormones and endocrine-disrupting chemicals: low-dose effects and nonmonotonic dose responses. Endocr Rev 2012; 33:378–455.
- Howdeshell KL A model of the development of the brain as a construct of the thyroid system. Environ Health Perspect 2002; 110 Suppl 3:337–348.
- Rice D, Barone S Jr. Critical periods of vulnerability for the developing nervous system: evidence from humans and animal models. Environ Health Perspect 2000; 108 Suppl 3:511–533.
- Chen F, Zhou L, Bai Y, et al. Sex differences in the adult HPA axis and affective behaviors are altered by perinatal exposure to a low dose of bisphenol A. Brain Res 2014; 1571:12–24.
- Cao J, Joyner L, Mickens JA, et al. Sex-specific Esr2 mRNA expression in the rat hypothalamus and amygdala is altered by neonatal bisphenol A exposure. Reproduction 2014; 147:537–554.
- Ceccarelli I, Della Seta D, Fiorenzani P, et al. Estrogenic chemicals at puberty change ERalpha in the hypothalamus of male and female rats. Neurotoxicol Teratol 2007; 29:108–115.
- Fukushima A, Funabashi T, Kawaguchi M, et al. Bisphenol A induces transforming growth factor-beta3 mRNA in the preoptic area: a cDNA expression array and Northern blot study. Neurosci Lett 2007; 411:81–85.
- Wolstenholme JT, Taylor JA, Shetty SR, et al. Gestational exposure to low dose bisphenol A alters social behavior in juvenile mice. PLoS One 2011; 6:e25448.
- Kundakovic M, Gudsnuk K, Herbstman JB, et al. DNA methylation of BDNF as a biomarker of early-life adversity. Proc Natl Acad Sci U S A 2015; 112:6807–6813.
- Arambula SE, Belcher SM, Planchart A, et al. Impact of Low Dose Oral Exposure to Bisphenol A (BPA) on the Neonatal Rat Hypothalamic and Hippocampal Transcriptome: a CLARITY-BPA Consortium Study. Endocrinology 157:3856–3872.
- Yaoi T, Itoh K, Nakamura K, et al. Genome-wide analysis of epigenomic alterations in fetal mouse forebrain after exposure to low doses of bisphenol A. Biochem Biophys Res Commun 2008; 376:563–567.
- Ho SM, Tang WY, Belmonte de Frausto J, et al. Developmental exposure to estradiol and bisphenol A increases susceptibility to prostate carcinogenesis and epigenetically regulates phosphodiesterase type 4 variant 4. Cancer Res 2006; 66:5624–5632.
- Tang WY, Newbold R, Mardilovich K, et al. Persistent hypomethylation in the promoter of nucleosomal binding protein 1 (Nsbp1) correlates with overexpression of Nsbp1 in mouse uteri neonatally exposed to diethylstilbestrol or genistein. Endocrinology 2008; 149:5922–5931.
- Cano-Nicolau J, Vaillant C, Pellegrini E, et al. Estrogenic Effects of Several BPA Analogs in the Developing Zebrafish Brain. Front Neurosci 2016; 10:112.
- Warita K, Mitsuhashi T, Hoshi N, et al. A unique pattern of bisphenol a effects on nerve growth factor gene expression in embryonic mouse hypothalamic cell line N-44. Arh Hig Rada Toksikol 2014; 65:293–299.
- Warita K, Mitsuhashi T, Ohta K, et al. Gene expression of epigenetic regulatory factors related to primary silencing mechanism is less susceptible to lower doses of bisphenol A in embryonic hypothalamic cells. J Toxicol Sci 2013; 38:285–289.
- Cao J, Rebuli ME, Rogers J, et al. Prenatal bisphenol A exposure alters sex-specific estrogen receptor expression in the neonatal rat hypothalamus and amygdala. Toxicol Sci 2013; 133:157–173.
- Cao J, Mickens JA, McCaffrey KA, et al. Neonatal Bisphenol A exposure alters sexually dimorphic gene expression in the postnatal rat hypothalamus. Neurotoxicology 2012; 33:23–36.
- Monje L, Varayoud J, Luque EH, et al. Neonatal exposure to bisphenol A modifies the abundance of estrogen receptor alpha transcripts with alternative 5ʹ-untranslated regions in the female rat preoptic area. J Endocrinol 2007; 194:201–212.
- Funabashi T, Kawaguchi M, Kimura F The endocrine disrupters butyl benzyl phthalate and bisphenol A increase the expression of progesterone receptor messenger ribonucleic acid in the preoptic area of adult ovariectomized rats. Neuroendocrinology 2001; 74:77–81.
- Goldsby JA, Wolstenholme JT, Rissman EF Multi- and transgenerational consequences of Bisphenol A on sexually dimorphic cell populations in mouse brain. Endocrinology 2017;158:21–30.
- Jacobs LF, Gaulin SJ, Sherry DF, et al. Evolution of spatial cognition: sex-specific patterns of spatial behavior predict hippocampal size. Proc Natl Acad Sci U S A 1990; 87:6349–6352.
- Pyter LM, Trainor BC, Nelson RJ Testosterone and photoperiod interact to affect spatial learning and memory in adult male white-footed mice (Peromyscus leucopus). Eur J Neurosci 2006; 23:3056–3062.
- Walton JC, Chen Z, Weil ZM, et al. Photoperiod-mediated impairment of long-term potention and learning and memory in male white-footed mice. Neuroscience 2011; 175:127–132.
- Kitraki E, Nalvarte I, Alavian-Ghavanini A, et al. Developmental exposure to bisphenol A alters expression and DNA methylation of Fkbp5, an important regulator of the stress response. Mol Cell Endocrinol 2015; 417:191–199.
- Kumar D, Thakur MK Effect of perinatal exposure to Bisphenol-A on DNA methylation and histone acetylation in cerebral cortex and hippocampus of postnatal male mice. J Toxicol Sci 2017; 42:281–289.
- Doshi T, Mehta SS, Dighe V, et al. Hypermethylation of estrogen receptor promoter region in adult testis of rats exposed neonatally to bisphenol A. Toxicology 2011; 289:74–82.
- Cheong A, Zhang X, Cheung YY, et al. DNA methylome changes by estradiol benzoate and bisphenol A links early-life environmental exposures to prostate cancer risk. Epigenetics 2016; 11:674–689.
- Prins GS, Ye SH, Birch L, et al. Prostate Cancer Risk and DNA Methylation Signatures in Aging Rats following Developmental BPA Exposure: a Dose-Response Analysis. Environ Health Perspect 2017; 125:077007.
- Wong RL, Wang Q, Trevino LS, et al. Identification of secretaglobin Scgb2a1 as a target for developmental reprogramming by BPA in the rat prostate. Epigenetics 2015; 10:127–134.
- Ho SM, Cheong A, Lam HM, et al. Exposure of Human Prostaspheres to Bisphenol A Epigenetically Regulates SNORD Family Noncoding RNAs via Histone Modification. Endocrinology 2015; 156:3984–3995.
- Kochmanski J, Marchlewicz EH, Savidge M, et al. Longitudinal effects of developmental bisphenol A and variable diet exposures on epigenetic drift in mice. Reprod Toxicol 2017; 68:154–163.
- Wang C, Li Z, Han H, et al. Impairment of object recognition memory by maternal bisphenol A exposure is associated with inhibition of Akt and ERK/CREB/BDNF pathway in the male offspring hippocampus. Toxicology 2016; 341-343:56–64.
- Manshack LK, Conard CM, Bryan SJ, et al. Transcriptomic alterations in the brain of painted turtles (Chrysemys picta) developmentally exposed to bisphenol A or ethinyl estradiol. Physiol Genomics 2017; 49:201–215.
- Heindel JJ, Newbold RR, Bucher JR, et al. NIEHS/FDA CLARITY-BPA Research Program Update. Reprod Toxicol 2015;58:33–44.
- National Research Council. Guide for the Care and Use of Laboratory Animals. Washington (DC): The National Academies Press; 2011.
- Churchwell MI, Camacho L, Vanlandingham MM, et al. Comparison of life-stage-dependent internal dosimetry for bisphenol A, ethinyl estradiol, a reference estrogen, and endogenous estradiol to test an estrogenic mode of action in Sprague Dawley rats. Toxicol Sci 2014; 139:4–20.
- Doerge DR, Twaddle NC, Vanlandingham M, et al. Pharmacokinetics of bisphenol A in neonatal and adult Sprague-Dawley rats. Toxicol Appl Pharmacol 2010; 247:158–165.
- Tyl RW, Myers CB, Marr MC, et al. Two-generation reproductive toxicity study of dietary bisphenol A in CD-1 (Swiss) mice. Toxicol Sci 2008; 104:362–384.
- Tyl RW, Myers CB, Marr MC, et al. Three-Generation reproductive toxicity study of dietary bisphenol A in CD Sprague-Dawley rats. Toxicol Sci 2002; 68:121–146.
- Paxinos G The Rat Brain in Stereotaxic Coordinates, 7th ed. Cambridge (MA): Academic Press; 2013.
- Chang SM, Chung LW Interaction between prostatic fibroblast and epithelial cells in culture: role of androgen. Endocrinology 1989; 125:2719–2727.
- Ho SM, Leav I, Damassa D, et al. Testosterone-mediated increase in 5 alpha-dihydrotestosterone content, nuclear androgen receptor levels, and cell division in an androgen-independent prostate carcinoma of Noble rats. Cancer Res 1988; 48:609–614.
- Tang WY, Morey LM, Cheung YY, et al. Neonatal exposure to estradiol/bisphenol A alters promoter methylation and expression of Nsbp1 and Hpcal1 genes and transcriptional programs of Dnmt3a/b and Mbd2/4 in the rat prostate gland throughout life. Endocrinology 2012; 153:42–55.
- Rao X, Huang X, Zhou Z, et al. An improvement of the 2^(-delta delta CT) method for quantitative real-time polymerase chain reaction data analysis. Biostat Bioinforma Biomath 2013; 3:71–85.