ABSTRACT
Although phenotypic abnormalities frequently appear in the placenta following somatic cell nuclear transfer (SCNT), mouse trophoblast stem cells (TSCs) established from SCNT embryos reportedly show no distinct abnormalities compared with those derived from normal fertilization. In this study, we reexamined SCNT–TSCs to identify their imprinting statuses. Placenta-specific maternally imprinted genes (Gab1, Slc38a4, and Sfmbt2) consistently showed biallelic expression in SCNT–TSCs, suggesting their loss of imprinting (LOI). The LOI of Gab1 was associated with decreased DNA methylation, and that of Sfmbt2 was associated with decreased DNA methylation and histone H3K27 trimethylation. The maternal allele of the intergenic differentially methylated region (IG–DMR) was aberrantly hypermethylated following SCNT, even though this region was prone to demethylation in TSCs when established in a serum-free chemically defined medium. These findings indicate that the development of cloned embryos is associated with imprinting abnormalities specifically in the trophoblast lineage from its initial stage, which may affect subsequent placental development.
Introduction
In mammalian embryogenesis, trophoblast lineage precursor cells first appear at the morula–blastocyst transition and commit to differentiation into functional placental cells after implantation. Whereas embryonic stem cells (ESCs) or epiblast stem cells are generated from the embryonic lineage, trophoblast stem cells (TSCs) are established from the trophoblast lineage cells in blastocysts or early postimplantation embryos [Citation1]. The establishment of mouse TSC lines was first reported in 1998 [Citation2]. Under appropriate culture conditions in the presence of fibroblast growth factor 4 (FGF4) and heparin, TSCs maintain their capacity to self-renew in vitro and differentiate into all trophoblastic cell types in vivo. Therefore, TSCs have been used extensively as experimental models to study the genetic and epigenetic mechanisms involved in trophoblast development and functions. TSC lines can also be established from cloned embryos generated by somatic cell nuclear transfer (SCNT) [Citation3]. A systematic analysis using several SCNT–TSC lines revealed that they were indistinguishable from those of natural fertilization-derived TSC lines in terms of gene expression profiles, DNA methylation patterns, and contribution to placental tissues after injection into blastocysts and transfer to recipient female mice. However, it has long been documented that SCNT-derived cloned embryos are prone to epigenetic and phenotypic abnormalities, which frequently appear in placental tissues [Citation4]. In mice, for example, hyperplastic development associated with enlargement of the trophospongium layer (junctional layer) occurs in all cloned placentas examined thus far, except for those with a specific genetic background [Citation5,Citation6]. Furthermore, extraembryonic tissues of SCNT-derived conceptuses also showed an abnormal phenotype shortly after implantation. They had a proliferative deficiency caused by precocious differentiation, leading to poor development of the ectoplacental cone and cytotrophoblast areas at implantation sites [Citation7,Citation8]. Based on these cumulative findings, we speculate that some unidentified epigenetic errors are inherent to extraembryonic lineages derived by SCNT throughout development. To test this, we examined whether SCNT–TSCs had any imprinting status aberrations, focusing on those of placenta-specific imprinted genes and the intergenic differentially methylated region (IG–DMR), which has been reported to be aberrant in placentas of cloned mice [Citation9].
Results and discussion
We first examined the expression alleles of three placenta-specific imprinted genes, Gab1, Slc38a4, and Sfmbt2, which showed biallelic expression in cloned placentas [Citation9]. The control in vitro fertilization (IVF)-derived TSCs showed normal paternal expressions of Slc38a4 and Sfmbt2 except for two lines (TS-5 and TS-6), which showed a minor maternal expression of Slc38a4 (, ). In contrast, Gab1 was biallelically expressed in most (three of four) control lines (). This was not because of contamination from feeder cells, as the embryonic fibroblasts did not express Gab1 (Figure S1). In contrast, all genes were expressed biallelically in SCNT–TSCs, except for the normal expression of Slc38a4 in one cumulus cell-derived line (ntTS-20) (, ). Consistent with the frequent biallelic expression of these imprinted genes in SCNT–TSCs, their expression levels were upregulated in these TSC lines (). There were significant differences in Slc38a4 and Sfmbt2, but not for Gab1, in the expression levels of SCNT–TSCs when compared with those of IVF–TSC lines. The minimum increase of the Gab1 expression might be due to its frequent biallelic expression in the IVF–TSC lines.
Table 1. The imprinting statuses of TSC lines derived from IVF or SCNT.
Figure 1. Analysis of the allelic expression and DNA methylation of placenta-specific imprinted genes in TSCs. While IVF-derived TSCs showed normal paternal expression (black arrows), SCNT-derived TSCs showed biallelic expression (red arrows). Demethylation of the Gab1 DMR consistently occurs in SCNT–TSCs while that of the Slc38a4 DMR showed a cell line-dependent difference. Open circles indicate unmethylated and filled circles indicate methylated CpG dinucleotides. Numbers in parentheses indicate the percentages of methylated CpG sites.
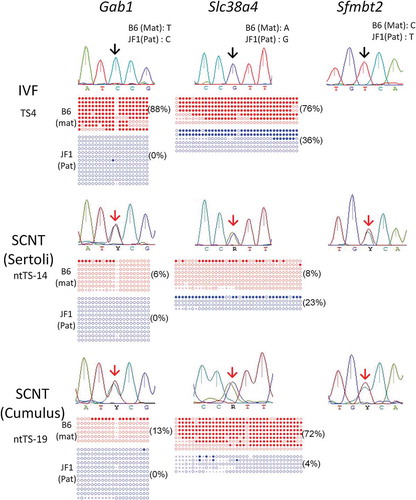
Figure 2. RT-qPCR analysis for Gab1, Slc38a4, and Sfmbt2. Four distinct IVF- and SCNT-derived TSC lines (TS-4, −5, −6, and −7; ntTS-13, −17, 18, and −19, respectively) were used. Each expression level was normalized against the level of beta-actin. Relative expression levels (mean ± standard deviation) from three independent experiments are shown. The average level of the IVF-derived lines was set as 1.0. *P < 0.01 (Student’s t-test).
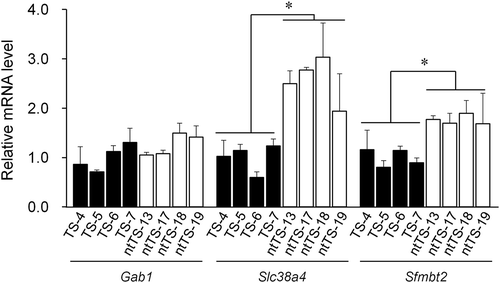
Next, we analyzed the DNA methylation patterns of these three genes by bisulfite sequencing [Citation9,Citation10]. Variation was high in the pattern of DNA methylation in these imprinted genes, with only a few showing a normal maternal methylation pattern (, ). However, the Gab1-DMR seemed more prone to hypomethylation irrespective of the embryo origin (IVF or SCNT) compared with the Slc38a4- and Sfmbt2-DMRs, consistent with its biallelic expression propensity. SCNT seemed to further promote this tendency because all four SCNT-derived lines showed hypomethylation less than 20% at the maternal allele of Gab1, while two of four IVF-lines maintained the methylation levels at more than 88.3% and 60.0%, respectively. Therefore, frequent biallelic expression of Gab1 in TSCs might be associated by its hypomethylated status. By contrast, for the Slc38a4 and Sfmbt2 genes, there was no clear correlation between its DMR methylation level and allelic expression pattern although Sfmbt2 of SCNT–TSCs frequently showed hypomethylated patterns ().
It has been recently shown that a repressive histone mark, trimethylation at lysine 27 of histone H3 (H3K27me3), is specifically involved in the regulation of five maternally imprinted genes (Gab1, Phf17, Sfmbt2, Slc38a4, and Smoc1) in preimplantation embryos and the early trophoblast lineage [Citation11]. It is possible that this H3K27me3 regulation might be taken over by more stable DNA methylation during placental development. Indeed, in embryo day (E)13.5 placentas, the paternal expression of Gab1 and Slc38a4 is associated with DNA methylation, while that of Sfmtb2 is associated with H3K27me3 [Citation9]. Therefore, we analyzed the promoter region of the Sfmbt2 gene for H3K27me3 enrichment in IVF- and SCNT-derived TSC lines by chromatin immunoprecipitation, followed by quantitative polymerase chain reaction (ChIP–qPCR). There was a significant decrease in H3K27me3 level at the Sfmbt2 promoter in the SCNT–TSC lines (ntTS-13, −17, −18, and −19) compared with the IVF–TSC lines (TS-4, −5, −6, and −7), which indicated the loss of Sfmbt2 imprinting in SCNT–TSCs ()). We confirmed that H3K27me3 was normally enriched in IVF-derived TSC lines at the maternal allele of the Sfmbt2 promoter in IVF-derived TSCs by a sequence analysis based on the polymorphisms between C57BL/6 and JF1 mice ()). Additionally, we also analyzed the Slc38a4 gene for H3K27me3 enrichment and found a decreased tendency in the SCNT–TSC lines, but without a significant difference between the IVF – and SCNT–TSC lines (P = 0.097, Figure S2). Possibly, Slc38a4 in TSCs might be at the transition stage of imprinting regulation from H3K27me3- to DNA methylation-dependent mechanisms.
Figure 3. Loss of H3K27me3 at the maternal Sfmbt2 promoter in SCNT–TSCs. (a) ChIP–qPCR analysis at the Sfmbt2 promoter. Four distinct IVF- and SCNT-derived TSC lines (TS-4, −5, −6, and −7; ntTS-13, −17, 18, and −19, respectively) were used. Enrichment of H3K27me3 was significantly decreased in all SCNT–TSC lines. Recovery efficiency (mean ± standard deviation) is shown as enrichment relative to input from three independent experiments. *P < 0.01 (Student’s t-test). (b) Allelic enrichment of H3K27me3 at the Sfmbt2 promoter. Both IVF–TSC lines (TS-4 and −5) showed maternal enrichment of H3K27me3 (black arrows), whereas there were biallelic signals in the input (red arrow). Data were obtained from three independent experiments.
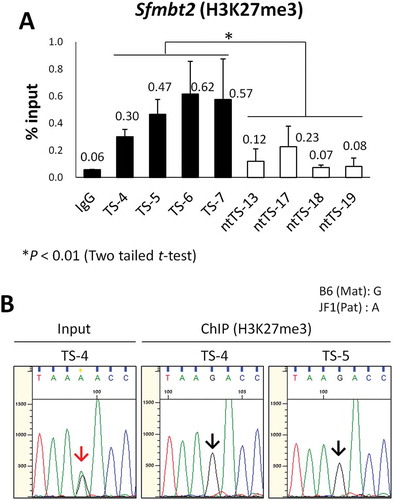
Collectively, our data suggest that SCNT–TSCs are prone to loss of imprinting (LOI) for the three placenta-specific imprinted genes analyzed. This is consistent with previous reports of SCNT-derived E13.5 placentas [Citation9], although SCNT–TSCs demonstrate more variable patterns in allelic gene expression and DMR methylation (particularly Slc38a4). It is likely that the LOI in SCNT-derived placentas and TSCs might be attributable to erasure of the parental-specific memory in the somatic lineages after implantation [Citation9,Citation11].
We also examined the methylation status of the IG–DMR, which is also frequently aberrant in SCNT-derived embryos [Citation9]. The percentages of methylated CpGs at each parental allele are shown in and . We performed a two-way analysis of variance (ANOVA) to determine the effects of parental allele and type of embryo on DNA methylation levels. Both factors had significant effects and there was no interaction between these factors, which indicates that they exert their effects differentially and that the methylation levels were: maternal allele < paternal allele; IVF < SCNT (P < 0.005 and P < 0.001, respectively). Thus, the maternal IG–DMR was aberrantly hypermethylated in SCNT–TSCs, as previously reported for SCNT-derived placentas [Citation9]. However, hypomethylated patterns were unexpectedly found at the paternal allele in three of the four control TSC lines (TS-4, TS-5, and TS-6), ranging from 30.7% to 33.9%, although that of the SCNT–TSC lines remained hypermethylated (78.1% to 94.8%), probably in response to the SCNT procedure at this region (, ). We hypothesized that the reduction in DNA methylation at the paternal IG–DMR might have been caused by the use of serum-free conditioned medium (CDM) at their establishment [Citation12], because it contains knockout serum supplement (KSR), known to induce hypomethylation of the IG–DMR in induced pluripotent stem cells (iPSCs) [Citation13]. Thus, we next examined the IG–DMR of an additional series of TSCs (two IVF–TSC lines and three SCNT–TSC lines), which had been generated using the conventional TSC medium containing FGF4, heparin, and serum during the first 12–46 days of culture. They had been maintained in CDM thereafter as for the lines established with CDM (). In these TSCs, the methylation level of the paternal IG–DMR was maintained at more than 90% irrespective of embryo origin (IVF or SCNT) () and (c)). This suggests that the use of CDM at the initial stage of establishing TSCs might induce DNA hypomethylation. Consistent with this, we found that the methylation levels of the pericentric repeats (major satellite regions) in TSC lines generated using culture in CDM were significantly lower than those in TSC lines generated in conventional medium [41.0 ± 6.0% and 86.6 ± 5.4%, respectively (mean ± SEM); P < 0.01, by Student’s t-test] () and (c)). The hypomethylation tendency induced by CDM might contribute to the efficient TSC generation reported for this medium [Citation12]. These findings indicate that the DNA methylation status of TSCs might be determined by the culture medium used during their establishment.
Figure 4. DNA methylation status of the IG–DMR in TSC lines derived from IVF or SCNT. SCNT–TSC lines were significantly hypermethylated at the IG–DMR (P < 0.0005, two-way ANOVA). Open circles indicate unmethylated and filled circles indicate methylated CpG dinucleotides.
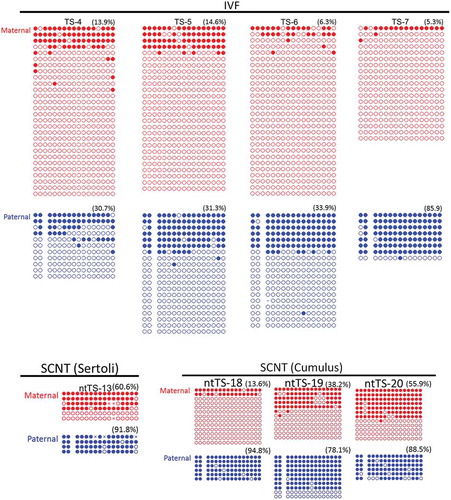
Figure 5. DNA methylation levels at the IG–DMR (paternal allele) and the major satellite region in TSC lines established using chemically defined medium (CDM) or conventional medium. (a) The protocol of TSC lines using chemically defined medium or conventional medium. The two protocols are different in the medium used at the initial stage of cell line establishment. (b) The DNA methylation levels were plotted according to data from bisulfite sequence analysis. Horizontal lines indicate mean levels. TSC lines established in chemically defined medium were prone to hypomethylation, although the IG–DMR in SCNT-derived TSC lines remained hypermethylated. *P < 0.05 (Student’s t-test). (b) Representative data from bisulfite sequence analysis. Data for the IG–DMR in TSCs established in chemically defined medium are shown in . Open circles indicate unmethylated and filled circles indicate methylated CpG dinucleotides.
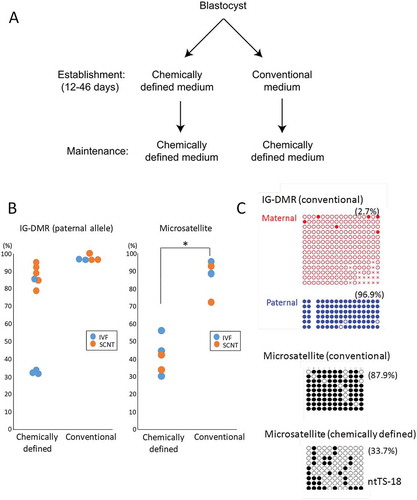
Mouse TSC lines are highly variable in many respects, such as colony morphology, differentiation ability, and gene expression patterns [Citation12,Citation14,Citation15]. This might have caused uncertainty about the normality or abnormality of SCNT–TSCs in previous studies [Citation3,Citation16,Citation17]. We also found that use of CDM for the establishment of TSC lines might compromise the allelic expression and DNA methylation of imprinted genes, indicating that the conventional TSC medium seems to be more suitable for these analyses. Here, we first confirmed that SCNT–TSCs carried epigenetic abnormalities in the imprinting statuses of at least three placenta-specific imprinted genes and the IG–DMR. The former gene groups showed LOI in terms of hypomethylated DMR or decreased H3K27me3 marks, which could be explained by the erasure of imprinting memory in the embryo proper (donor somatic cells) [Citation11]. Among the three placenta-specific imprinted genes analyzed, Gab1 and Sfmbt2 are known to promote placental development, as revealed by gene knockout studies [Citation18,Citation19]. Although there has been no functional study on Slc38a4 in mice, the human SLC38A4 gene encoding a neutral amino acid transporter expressed in the placenta is often associated with fetal macrosomia, which indicates its positive nutritional effect on the fetus, and possibly the placenta [Citation20]. Therefore, it is conceivable that increased expressions of these placenta-specific imprinted genes by LOI might cause enlargement of placentas in cloned fetuses from midgestation onward. In contrast, the reason(s) for the frequent association of hypermethylated IG–DMR in cloned placentas is still unclear. In mouse ESCs and iPSCs, this region is susceptible to hypermethylation [Citation13] and its normal hypomethylated state is maintained by polycomb repressive complex 2 (PRC2) that inhibits recruitment of Dnmt3 methyltransferases to this site [Citation21]. It would be interesting to examine whether this mechanism is dysregulated in cloned placentas. The IG–DMR regulates many imprinted genes within its region for their parental origin-specific expressions in the developing fetus and placenta. Indeed, upregulation of Dlk1, one of the IG–DMR-regulated genes, is often associated with death in early cloned fetuses, probably because of hypermethylation of the IG–DMR [Citation7,Citation9]. It is probable that the abnormal development of placentas in cloned mice is also affected by the hypermethylated IG–DMR.
Although it has been reported that SCNT–TSCs show no distinct epigenetic abnormalities compared with those derived from normal fertilization, our data clearly indicate that they are prone to specific imprinting abnormalities. Thus, SCNT may compromise the development of the trophoblast lineage at its earliest stage, which might affect the survivability of SCNT-derived cloned embryos. The frequent death of cloned embryos at the early postimplantation stage cannot be rescued even with strategies developed thus far such as correction of Xist expression [Citation22] and removal of donor-origin H3K9me3 [Citation23]. Therefore, our findings contribute important clues for improving the future efficiency of mouse cloning by SCNT.
Materials and methods
Animal experimentation
Experiments using mice were approved by the institutional animal care and use committee of RIKEN Tsukuba Branch and were performed in accordance with the committee’s guiding principles.
Establishment of TSC lines
TSC lines were established from blastocysts generated by IVF or SCNT. IVF was undertaken using oocytes from C57BL/6NCrSlc (Mus musculus domesticus) female mice (Japan Slc) and spermatozoa from JF1/Ms (M. m. molossinus) male mice (RIKEN BioResource Center) [Citation24]. SCNT experiments were performed using (C57BL/6NCrSlc × DBA/2CrSlc) F1 oocytes (Japan Slc) and (C57BL/6NCrSlc × JF1/Ms) F1 cumulus cells or immature Sertoli cells as described [Citation25]. For establishment of TSCs, developing blastocysts were transferred to CDM [Citation12] (described below) on layers of primary mouse embryonic fibroblasts treated with mitomycin-C (Sigma-Aldrich). After several passages, cell lines with typical TSC colonies were used.
TSC maintenance under defined conditions
TSCs were cultured in CDM as described previously [Citation12] with some modifications. CDM was generated by combining the same amount of neurobasal medium (Gibco) and Dulbecco’s modified Eagle’s medium/F12 (Ham’s) (1:1) (Gibco) supplied with N2 supplement (Gibco), B27 supplement (Gibco), penicillin–streptomycin solution (Gibco), GlutaMAX supplement (Gibco), 2-mercaptoethanol (Sigma-Aldrich; 150 μM final concentration), heparin (Sigma-Aldrich, 1 μg/mL final concentration), bovine serum albumin (Millipore, 0.05% final concentration), KnockOut serum replacement (Gibco, 1% final concentration), recombinant mouse basic FGF (Wako, 50 ng/ml final concentration), recombinant human activin A (R&D systems, 20 ng/ml final concentration), XAV939 (Calbiochem, 10 μM final concentration), and Y27632 (Wako, 5 μM final concentration). Medium was replaced daily or every 2 days. TripLE Select (Gibco) was used to disperse TSCs and the cells were then plated on dishes coated with 15 mg/ml human plasma fibronectin (Millipore).
Allelic expression analysis
We used established methods for allelic expression analysis [Citation9,Citation26]. Briefly, cDNA was reverse-transcribed from total RNAs using the SuperScriptTM III First-Strand Synthesis System (Thermo Fisher Scientific). Prepared cDNA samples were amplified by PCR and the products were sequenced using an ABI 3730xl DNA Analyzer (Applied Biosystems). The primer sets are shown in . Expressions of alleles were determined by comparing single nucleotide polymorphisms (SNPs) between sequences for mouse strains C57BL/6NCrSlc (Mus musculus domesticus; maternal allele) and JF1/Ms (M. m. molossinus; paternal allele).
Table 2. Primer sets used in this study.
Bisulfite sequence analysis
Each DNA sample was subjected to bisulfite modification using a solution of 10 M sodium bisulfite, denatured at 98°C for 1 min and incubated for 1 h at 70°C. Desulfonation and purification of bisulfite-treated DNA were performed using a bisulfite DNA purification kit (Zymo Research). Following PCR amplification, the products were cloned into pGEM T-Easy vectors (Promega) and individual clones were sequenced. The primer sets are shown in . For the IG–DMR, alleles were determined from different SNPs between sequences for mouse strains C57BL/6NCrSlc (Mus musculus domesticus; maternal allele) and JF1/Ms (M. m. molossinus; paternal allele).
ChIP–qPCR analysis
Fixation and chromatin shearing were performed using truChIP Chromatin Shearing Reagent Kits (Covaris) according to the manufacturer’s instructions. Briefly, 2 × 106 TSCs were washed and fixed by 0.5% formaldehyde for 5 min. Chromatin shearing was performed using a Covaris S220 instrument (Covaris) for 15 cycles with its default parameters. The supernatant containing 2.3 μg of DNA was incubated with 1 μg of anti-H3K27me3 (MBL, MABI0323) or Rabbit IgG (Abcam, ab46540) antibodies prebounded to Dynabeads Protein G (Invitrogen) in ChIP dilution buffer [0.01% sodium dodecyl sulfate (SDS), 1.1% w/v Triton X-100, 1.2 mM ethylenediaminetetraacetic acid (EDTA), 0.25 μg/μl bovine serum albumin (BSA) and 167 mM NaCl] at 4°C overnight with rotation. The immunocomplexes were washed with low salt buffer (20 mM Tris-HCl, 0.1% SDS, 1% w/v Triton X-100, 2 mM EDTA and 150 mM NaCl) and high salt buffer (20 mM Tris-HCl, 0.1% SDS, 1% w/v Triton X-100, 2 mM EDTA and 500 mM NaCl), then eluted by elution buffer (10 mM Tris-HCl, 1% SDS, 5 mM EDTA and 300 mM NaCl) at 65°C for 30 min. Crosslinking was reversed by incubation at 65°C for 6 h, after which RNAs and proteins were degraded by adding RNase A (Ambion) at 37°C for 30 min and Proteinase K (Zymo Research) at 55°C for 2 h, respectively. DNA was purified using QIAquick PCR purification kits (Qiagen). The amount of ChIPed-DNA at Sfmbt2 locus [Citation9] was quantified with QuantStudio7 Flex systems (Applied Biosystems) using the PowerUp SYBR Green Master Mix (Applied Biosystems) according to the manufacturer’s instructions. The primers used are shown in .
RT–qPCR analysis
Total RNA was extracted using RNeasy Mini kits (Qiagen) according to the manufacturer’s instructions. For reverse transcription, a SuperScriptTM III First-Strand Synthesis System for RT–PCR and oligo (dT)20 was used. Expression levels were quantified using a QuantStudio 7 Flex Real-Time PCR System with PowerUp SYBR Green Master Mix and normalized against the level of beta-actin. Primer sequences are shown in .
Statistical analysis
DNA methylation levels of IG–DMR were analyzed by two-way (parental allele × embryo type) ANOVA following arcsine transformation of the percentages of methylated CpG sites. Other statistical methods are indicated in the results, as appropriate.
Supplemental Material
Download MS Power Point (1.1 MB)Acknowledgments
The JF1/Msf strain (RBRC00639) was provided by the RIKEN BioResource Center, with the support of the National BioResource Project of MEXT.
Disclosure statement
No potential conflict of interest was reported by the authors.
Supplemental Material
Supplemental data for this article can be accessed here.
Additional information
Funding
References
- Roberts RM, Fisher SJ. Trophoblast stem cells. Biol Reprod. 2011;84:412–421. PMID:21106963.
- Tanaka S, Kunath T, Hadjantonakis AK, et al. Promotion of trophoblast stem cell proliferation by FGF4. Science. 1998;282:2072–2075, PMID:9851926.
- Oda M, Tanaka S, Yamazaki Y, et al. Establishment of trophoblast stem cell lines from somatic cell nuclear-transferred embryos. Proc Natl Acad Sci USA. 2009;106:16293–16297. PMID:19706390.
- Yang X, Smith SL, Tian XC, et al. Nuclear reprogramming of cloned embryos and its implications for therapeutic cloning. Nat Genet. 2007;39:295–302. PMID:17325680.
- Inoue K, Ogura A. In quest of genomic treasure. J Reprod Dev. 2015;61:489–493. PMID:26400375.
- Wakayama T, Yanagimachi R. Cloning of male mice from adult tail-tip cells. Nat Genet. 1999;22:127–128. PMID:10369248.
- Hirasawa R, Matoba S, Inoue K, et al. Somatic donor cell type correlates with embryonic, but not extra-embryonic, gene expression in postimplantation cloned embryos. PLoS One. 2013;8:e76422. PMID:24146866.
- Wakisaka-Saito N, Kohda T, Inoue K, et al. Chorioallantoic placenta defects in cloned mice. Biochem Biophys Res Commun. 2006;349:106–114. PMID:16938271.
- Okae H, Matoba S, Nagashima T, et al. RNA sequencing-based identification of aberrant imprinting in cloned mice. Hum Mol Genet. 2014;23:992–1001. PMID:24105465.
- Kuzmin A, Han Z, Golding MC, et al. The PcG gene Sfmbt2 is paternally expressed in extraembryonic tissues. Gene Expr Patterns. 2008;8:107–116. PMID:18024232.
- Inoue A, Jiang L, Lu FL, et al. Maternal H3K27me3 controls DNA methylation-independent imprinting. Nature. 2017;547:419–424. PMID:WOS:000406358300028.
- Ohinata Y, Tsukiyama T. Establishment of trophoblast stem cells under defined culture conditions in mice. PLoS One. 2014;9:e107308. PMID:25203285.
- Stadtfeld M, Apostolou E, Ferrari F, et al. Ascorbic acid prevents loss of Dlk1-Dio3 imprinting and facilitates generation of all-iPS cell mice from terminally differentiated B cells. Nat Genet. 2012;44:398–405. PMID:WOS:000302130600013.
- Motomura K, Inoue K, Ogura A. Selection of accurate reference genes in mouse trophoblast stem cells for reverse transcription-quantitative polymerase chain reaction. J Reprod Dev. 2016;62:311–315. PMID:26853688.
- Motomura K, Oikawa M, Hirose M, et al. Cellular dynamics of mouse trophoblast stem cells: identification of a persistent stem cell type. Biol Reprod. 2016;94:122. PMID:27122635.
- Rielland M, Brochard V, Lacroix MC, et al. Early alteration of the self-renewal/differentiation threshold in trophoblast stem cells derived from mouse embryos after nuclear transfer. Dev Biol. 2009;334:325–334. PMID:19631638.
- Rielland M, Hue I, Renard JP, et al. Trophoblast stem cell derivation, cross-species comparison and use of nuclear transfer: new tools to study trophoblast growth and differentiation. Dev Biol. 2008;322:1–10. PMID:18680738.
- Itoh M, Yoshida Y, Nishida K, et al. Role of Gab1 in heart, placenta, and skin development and growth factor- and cytokine-induced extracellular signal-regulated kinase mitogen-activated protein kinase activation. Mol Cell Biol. 2000;20:3695–3704. PMID:WOS:000086698100037.
- Miri K, Latham K, Panning B, et al. The imprinted polycomb group gene Sfmbt2 is required for trophoblast maintenance and placenta development. Development. 2013;140:4480–4489. PMID:WOS:000326681900004.
- Li Z, Lai GR, Deng LJ, et al. Association of SLC38A4 and system A with abnormal fetal birth weight. Exp Ther Med. 2012;3:309–313. PMID:WOS:000299363900026.
- Das PP, Hendrix DA, Apostolou E, et al. PRC2 is required to maintain expression of the maternal Gtl2-Rian-Mirg locus by preventing de novo DNA methylation in mouse embryonic stem cells. Cell Rep. 2015;12:1456–1470. PMID:WOS:000360574200010.
- Inoue K, Kohda T, Sugimoto M, et al. Impeding Xist expression from the active X chromosome improves mouse somatic cell nuclear transfer. Science. 2010;330:496–499. PMID:WOS:000283329100037.
- Matoba S, Liu YT, Lu FL, et al. Embryonic development following somatic cell nuclear transfer impeded by persisting histone methylation. Cell. 2014;159:884–895. PMID:WOS:000344522000019.
- Hasegawa A, Mochida K, Tomishima T, et al. Microdroplet in vitro fertilization can reduce the number of spermatozoa necessary for fertilizing oocytes. J Reprod Dev. 2014;60:187–193. PMID:WOS:000338271700003.
- Kishigami S, Wakayama S, Thuan NV, et al. Production of cloned mice by somatic cell nuclear transfer. Nat Protoc. 2006;1:125–138. PMID:WOS:000251002200021.
- Kamimura S, Hatanaka Y, Hirasawa R, et al. Establishment of paternal genomic imprinting in mouse prospermatogonia analyzed by nuc-lear transfer. Biol Reprod. 2014;91:120. PMID:WOS:000209831900006.