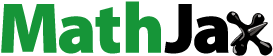
ABSTRACT
AXL is one of the TAM (TYRO3, AXL and MERTK) receptor tyrosine kinases and may be involved in airway inflammation. Little is known about how epigenetic changes in AXL may affect lung development during adolescence. We investigated the association between AXL DNA methylation at birth and lung function growth from 10 to 18 years of age in 923 subjects from the Children’s Health Study (CHS). DNA methylation from newborn bloodspots was measured at multiple CpG loci across the regulatory regions of AXL using Pyrosequencing. Linear spline mixed-effects models were fitted to assess the association between DNA methylation and 8-year lung function growth. Findings were evaluated for replication in a separate population of 237 CHS subjects using methylation data from the Illumina HumanMethylation450 (HM450) array when possible. A 5% higher average methylation level of the AXL promoter region at birth was associated with a 48.4 ml decrease in mean FEV1 growth from 10 to 18 years of age in the primary study population (95% CI: −100.2, 3.4), and a 53.9 ml decrease in mean FEV1 growth from 11 to 15 years of age in the replication population (95% CI: −104.3, −3.5). One CpG locus in the promoter region, cg10564498, was significantly associated with decreased growth in FEV1, FVC and MMEF from 10 to 18 years of age and the negative associations were observed in a similar age range in the replication population. These findings suggest a potential association between AXL promoter methylation at birth and lower lung function growth during adolescence.
Background
Optimal lung function is important for health, as reduced lung function has been associated with increased risks of coronary artery disease and respiratory disease in adults, and with the onset of asthma in adolescents [Citation1,Citation2]. Many factors, environmental, pathological and genetic, may influence lung function [Citation3–Citation6]. These factors may exert their influence through epigenetic mechanisms such as DNA methylation by altering the expression pattern and activity of genes involved in airway development [Citation7,Citation8]. Researchers have identified associations between lung function decline and DNA methylation of the transposable elements LINE-1 [Citation9], and of several candidate genes such as CRAT, F3, TLR2 and SERPINA1, mostly among older subjects [Citation10,Citation11]. DNA methylation at birth may be an early surrogate marker of chronic disease predisposition that is affected by prenatal environmental exposure [Citation12]. However, few studies have investigated the early-life epigenetic marks associated with lung function growth in healthy adolescents.
Methylation of AXL, a member of the TAM (TYRO3, AXL and MERTK) family receptor tyrosine kinases, was identified in our previous work as responsive to prenatal tobacco smoke exposure [Citation13,Citation14]. AXL has been implicated in various biological pathways including clearance of apoptotic cells, natural killer cell differentiation, and inhibition of proinflammatory cytokines induced by Toll-like receptors (TLR) [Citation15–Citation17]. Recent evidence suggests that AXL is expressed in both human and mouse airway/alveolar macrophages and is critical for effective phagocytosis. Reduced AXL expression may contribute to persistent airway inflammation through inefficient clearance of apoptotic cells from inflamed lungs [Citation18,Citation19]. Since defective phagocytosis and subsequent airway inflammation are closely related to accelerated lung function decline and chronic lung diseases [Citation3,Citation20], we hypothesized that increased methylation in AXL promoter region may be associated with reduced lung function growth even early in life, potentially through repressing its expression and promoting inflammation in the airways.
In this study, we investigated the association between methylation of multiple CpG sites across the regulatory regions of AXL at birth and lung function growth from 10 to 18 years of age. Methylation was first assessed using Pyrosequencing in newborn bloodspots from a subset of 923 subjects from the Children’s Health Study (CHS) [Citation21–Citation23]. We then sought to replicate the association in a separate population of 237 CHS subjects using methylation levels measured from the Infinium HumanMethylation450 BeadChip (HM450) array, and in whom lung function was assessed from 11 to 15 years of age.
Results
Characteristics of the study participants
The characteristics of the primary and replication populations are shown in . There were fewer boys than girls in both the primary study population (46.7% male subjects) and replication population (41.8% male subjects). The primary study population also had more white subjects (46.3% versus 32.1%), slightly higher parental education level, more asthmatics (21.5% versus 14.8%), more subjects exposed to prenatal tobacco smoke by design (22.9% versus 9.0%), and more subjects exposed to environmental tobacco smoke (20.2% versus 6.8%). There were very few smokers in both populations. Mean age at first pulmonary function testing was 10.8 and 11.4 years in the primary and replication populations, respectively. There were generally no significant differences for mean height and BMI at age 11, 13 and 15 years between the two populations.
Table 1. Characteristics of participants with lung function testing.
Genomic locations of the AXL CpG sites under investigation are shown in . Methylation levels at many of the CpG sites were significantly correlated (Table S1 and S2), with CpG sites closer to each other showing stronger correlations. The distribution of methylation at each CpG site in both populations is shown in Table S3. The patterns of lung function growth across the study period are shown in Table S4 and Figure S1.
Figure 1. Genomic locations of AXL CpG sites under investigation.
Solid black box: CpG sites in the promoter region (CpG1-CpG 6); dashed gray box: CpG sites in the gene-body region (CpG 7-CpG 11); dashed black box: CpG site in the 3ʹuntranslated region (CpG 12).Cg number in parenthesis: corresponding CpG locus in HM450 array.
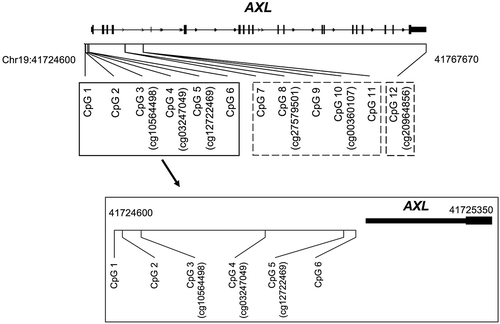
DNA methylation of AXL and lung function growth
We investigated whether AXL DNA methylation was associated with lung function growth in both the primary and replication populations. A 5% higher average promoter methylation level was associated with a 48.4 ml lower mean FEV1 growth from 10 to 18 years of age in the primary study population (95% CI: −100.2, 3.4; P = 0.07) (-Panel A and ), and significantly lower mean FEV1 growth from 11 to 15 years of age in the replication population (= -53.9; 95% CI: −104.3, −3.5; P = 0.04) (-Panel D and ). Similar negative associations with MMEF growth were observed in both populations. A 5% higher average promoter methylation level was associated with a 89.6 ml/sec lower mean 8-year MMEF growth (95% CI: −192.8, 13.7; P = 0.09) and the association with growth from 11 to 15 years was even stronger (
= -166.2; 95% CI: −278.4, −54.1; P = 0.004) (). We also investigated whether the relationship between AXL promoter methylation and lung function growth differed by sex (). Although there was no significant interaction, a stronger association was observed for boys than girls in both populations. For instance, in the primary study population, a 5% higher average promoter methylation was associated with a 69.4 ml decrease in mean 8-year FEV1 growth in boys (95% CI: −139.9, 1.1; P = 0.05) but only a 33.0 ml decrease in girls (95% CI: −94.1, 28.0; P = 0.29). In the replication population, a significant association between AXL promoter methylation and FEV1 growth from 11 to 15 years of age was also observed only in boys (
= -93.6; 95% CI: −166.1, −21.1; P = 0.01) but not in girls (
= -17.5; 95% CI: −87.8, 52.8; P = 0.62). There was no significant interaction between methylation and child’s ethnicity in both populations (Table S5).
Table 2. Association between lung function growth and AXL promoter methylation stratified by sex in the primary study population (N = 923) and replication population (N = 237)a.
Figure 2. Mean lung function growth versus the average methylation level of AXL promoter region.
AXL promoter methylation levels were first categorized into equal-sized groups based on deciles. The mean growth in FEV1, FVC and MMEF from 10 to 18 years of age in the primary study population (Panel A-C) and 11 to 15 years of age in the replication population (Panel D-F) are plotted against the corresponding levels of methylation in each decile. The corresponding coefficients and P-values are shown.FEV1, forced expiratory volume in 1 s; FVC, forced vital capacity; MMEF, maximal midexpiratory flow rate.
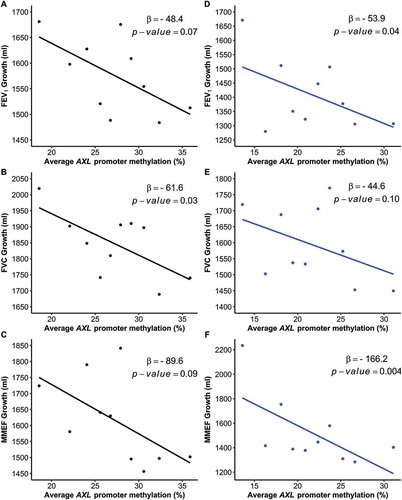
In the primary study population, a 5% higher average promoter methylation level was significantly associated with a 61.6 ml lower mean FVC growth from 10 to 18 years of age (95% CI: −117.5, −5.7; P = 0.03) (). The association between AXL promoter methylation and lower mean FVC growth was marginally significant in the replication population, yet still suggesting the same trend (= -44.6; 95% CI: −97.2, 8.1; P = 0.10).
Methylation at several individual CpG sites in the promoter region was also associated with lower lung function growth (Figure S2-Panel A). For example, methylation at CpG 3 was significantly associated with lower growth in FEV1, FVC and MMEF from 10 to 18 years of age in the primary study population (Table S6). Methylation at CpG 2, which is located near CpG 3, was also significantly associated with lower mean 8-year FVC growth, and lower attained FEV1 and FVC levels at 18 years of age. In the replication sample, we observed associations between CpG 3 methylation with lower growth in FEV1 and MMEF from 11 to 15 years of age (Table S7). No associations between lung function and methylation of specific CpG sites in the gene-body region or 3ʹ untranslated region were observed (Table S6).
Since asthma itself is associated with lower lung function growth in children [Citation24] and the prevalence of asthma in the primary study population was higher, we also conducted sensitivity analyzes in non-asthmatic subjects (Table S8). The observed associations were generally consistent with using all subjects.
AXL promoter methylation and expression in lung
AXL was previously reported to be expressed at very low levels in blood [Citation25]. Thus, we evaluated the correlation between AXL promoter methylation and mRNA expression levels using histologically normal lung tissue samples based on HM450 array and RNA sequencing data from TCGA (Figure S3). Average promoter methylation showed negative correlation with expression (r = -0.40, P = 0.03). Although these data need to be interpreted with caution because they were generated in an older population enriched with smokers, they showed preliminary evidence that higher methylation might be associated with lower AXL expression level in the adult lung.
Lastly, we integrated chromHMM and RNA-seq data from the Roadmap Epigenomics Project to display a functional annotation of CpG sites in the promoter region in relevant tissues and cell types (Figure S2-Panel B). The CpG loci evaluated in the AXL promoter region are located adjacent to enhancers in both fetal and adult lung fibroblast cells (yellow in chromHMM tracks), but are located within transcriptionally-repressed region in cord blood primary T cells. The observed chromatin state patterns are similar in fetal and adult lung cells. As indicated from RNA-seq data, the CpG sites under investigation are located within or near the transcriptionally active region in both fetal and adult lung, and a consistent pattern is observed in adult blood progenitor cells, though at much lower levels. These data suggest that epigenetic alterations in this region of AXL may be associated with active patterns for transcription and chromatin state of this gene throughout life, supporting the use of blood as surrogate marker of AXL’s activity in fetal and adult lung.
Discussion
We showed that increased average methylation of the AXL promoter region at birth was associated with lower lung function growth during adolescence, as measured by the growth in FEV1, FVC and MMEF from the ages of 10 to 18 years. Similar associations were observed for the average promoter methylation and specifically, CpG 3 in this region in a separate population of 237 CHS subjects, despite differences in the age at which lung function was measured as well as differences in some baseline characteristics of the two populations.
AXL and other TAM receptors are broadly expressed by cells of the vascular, nervous, immune and reproductive systems [Citation26]. Although the role of AXL in the pathogenesis of cancers and cardiovascular events is well-characterized [Citation27,Citation28], little is known about its role in pulmonary homeostasis, which requires a balance between adequate responses to pathogens and control of inflammatory processes arising from accumulation of apoptotic cells and cellular products [Citation29]. Inefficient clearance of apoptotic cells in the airways may lead to accumulation of necrotic cell debris, subsequent uncontrolled inflammatory responses, and ineffective resolution of lung inflammation upon microbial and viral infection [Citation30]. Recent evidence from mouse models suggests that Axl is expressed in airway macrophages [Citation18] – the main cell populations responsible for phagocytosis in the respiratory tract [Citation30] – and is essential for macrophage function through clearance of apoptotic cells and cell debris and inhibiting influenza-induced inflammation [Citation18]. Similar findings were reported in human patients with moderate-to-severe asthma, suggesting a critical role of AXL in clearing apoptotic cells from the inflamed lung [Citation19].
This is the first paper investigating the role of epigenetic regulation of AXL in lung function growth during adolescence. Understanding predictors of lung function growth is important considering the maximal attained lung function predicts risk of subsequent cardiovascular and respiratory conditions during adult life [Citation31,Citation32]. Because we previously found that AXL methylation was associated with risk of asthma-related symptoms in childhood [Citation25], and asthma may affect the rate of lung function growth [Citation24], we performed sensitivity analyzes in subjects who were never diagnosed with asthma. Similar associations in non-asthmatics indicate that the epigenetic regulation of AXL on lung function growth may act independently of asthma status and probably through different biological pathways. The question of whether AXL regulates lung homeostasis mainly through the apoptosis pathways as opposed to also exerting negative control over pro-inflammatory cytokines and TLR signaling in the airway [Citation16,Citation26] remains unclear. Our results also support a stronger association between AXL promoter methylation and lower lung function growth among boys than girls. Despite the non-significant differences, the findings highlight the need for investigating whether there are sex-specific effects.
The AXL promoter region under investigation in this paper overlapped with the core promoter region (−556 to −182 upstream to translational start codon), which is a known Sp1/Sp3 transcription factor binding site. Methylation within or in close proximity to this core region is negatively associated with AXL gene expression in cultured colon cancer cells [Citation33]. Due to the low expression of AXL in blood [Citation34], we were not able to test whether AXL methylation correlated with mRNA expression in our population. Nonetheless, average promoter methylation of AXL was shown to correlate with lower mRNA level in normal lung tissue samples from the TCGA database, suggesting a potential link between higher promoter methylation and lower expression level of this gene. Moreover, in silico analyzes using data from the Roadmap Epigenomics Project suggest that this region may be associated with active transcription in the lung since it is located adjacent to enhancers in both fetal and adult lung fibroblast cells.
The strengths of this study include the analysis of DNA methylation using Pyrosequencing, a highly reproducible method that accurately quantifies DNA methylation [Citation35], and the replication of results in a separate population using a different assay. The measurement of DNA methylation utilized archived newborn bloodspots, which constitute a unique and underutilized resource of biospecimens that represent essentially every child born in the countries with newborn screening programs [Citation36]. The cost, ease of transportation and storage of bloodspot cards point to new opportunities for using the neonatal biobanks available worldwide to facilitate population-based studies on epigenetic modifications at birth [Citation37–Citation39]. We also utilized the longitudinal measurements of pulmonary function and covariates data, collected in a consistent manner throughout the study period, to study the lung function growth prospectively. The temporal separation of DNA methylation assessment (at birth) and lung function growth measurement (during adolescence) removes the possibility of reverse causation.
Some limitations are noteworthy. First, the replication population was a different wave of CHS recruited in a different decade but not an external population. Since our analyzes were based mainly on Hispanic and non-Hispanic whites as well as non-smokers, it remains to be investigated whether our findings can be extrapolated to other ethnicities or to active smokers. The methylation sites measured in the replication population did not capture the exact range of the promoter region and this may also limit the generalizability of our results. Secondly, as in any epidemiologic study, the observed effects could be biased by some unknown confounders associated with both DNA methylation levels and lung development. Since DNA methylation was only measured from newborn bloodspots in our study, we were not able to evaluate whether the methylation pattern in AXL still persists during adolescence, or whether there is any correlation between AXL methylation in blood and other more pathologically-relevant tissues such as lung or airway epithelial cells. Nonetheless, the epigenetic changes at birth may reflect a summary of in-utero environmental exposures that affect different tissues systematically. The subtle shifts in methylation occurring during fetal development reflected in newborn blood may serve as early biomarkers for the disruption of pathways involved in lung function growth later in life.
In conclusion, our results suggest a potential association between AXL promoter DNA methylation at birth and lower lung function growth during adolescence. Future studies on gene-specific methylation will improve our understanding of the relationship between epigenetic changes and lung development.
Methods
Study population
This study was conducted in participants from the Children’s Health Study, a longitudinal study of respiratory health of children in southern California [Citation21–Citation23]. Based on our ability to link CHS subjects with California birth records and to obtain a newborn bloodspot, a subset of 923 children was selected for an epigenetic study in which DNA methylation at multiple CpG loci on AXL was assessed using Pyrosequencing. Subjects were recruited either as fourth-grade students in 1996 (cohort D) or as kindergarten and first-grade students in 2003 (cohort E). The sample selected was enriched with subjects exposed to prenatal tobacco smoke. The replication population included 237 CHS subjects from cohort E who had previously participated in a substudy of childhood cardiovascular health [Citation40], for whom California birth records could be linked and at least 700ng of DNA was available from a dried newborn bloodspot. In these subjects bloodspot DNA methylation was measured with the HM450 array.
Written questionnaires were completed by children’s parents at study entry and were updated annually throughout the study thereafter. The questionnaires were used to obtain personal, parental and socio-demographic characteristics with respect to the child, which included the child’s age, sex, race, ethnic origin, level of parental education, and in-utero as well as post-natal tobacco smoke exposure.
The study was approved by the Institutional Review Board of the University of Southern California.
Pulmonary-function testing
Trained technicians measured subjects’ weight and height, and supervised performance of pulmonary-function maneuvers. Details of the testing protocol have been published previously [Citation22]. Three measures of pulmonary-function were analyzed for each child: forced vital capacity (FVC), forced expiratory volume in the first second (FEV1), and maximal midexpiratory flow rate (MMEF). Pulmonary-function testing was performed annually from approximately 10 to 18 years of age with the use of rolling-seal spirometers in cohort D. Cohort E subjects were tested for pulmonary-function every other year when they were approximately 11, 13 and 15 years of age with the use of pressure transducer-based spirometers. Information on asthma status and personal smoking status was collected at the time of each pulmonary-function testing.
DNA methylation
DNA methylation was measured in newborn bloodspots (NBS) obtained as part of the routine California Newborn Screening Program from the California Department of Public Health Genetic Disease Screening Program. The NBS were stored by the state of California at −20 degrees Celsius. A single complete newborn bloodspot for each requested participant was mailed to us and stored in our lab at −80 degrees Celsius upon receipt. Laboratory personnel performing DNA methylation analysis were blinded to study subject information. Using a disposable scalpel, 1/2 of the dried bloodspot in the Guthrie card was cut out and further divided into 4 pieces each equivalent to 1/8 spot. The pieces were transferred to two microcentrifuge tubes each containing two of the 1/8 spots. DNA was extracted using the QiaAmp DNA Micro kit (Cat # 56,304; Qiagen Inc, Valencia, CA). The procedure consisted of lysis of the sample followed by binding of the DNA in the lysate to the Minielute column. The column was then washed and the purified DNA was eluted with 60 l of buffer AE and stored at −80 degrees Celsius.
Eleven CpG loci (CpG 1–3, and 5–12) spanning the regulatory regions of AXL were selected for Pyrosequencing assays (); five (CpG 3, 5, 8, 10 and 12) were located at positions corresponding to positions in the HM450 array. Methylation of CpG 1 was previously reported to be associated with prenatal tobacco smoke exposure [Citation13,Citation14]. Pyrosequencing assays were conducted as previously described [Citation25]. PCR primers were designed by EpigenDx Inc. (http://www.epigendx.com) to cover the loci of interest. Methylation assays (assays ADS6525-FS, ADS8094-FS2, ADS6528-FS, ADS8097-FS, and ADS6570-FS) were performed by EpigenDx Inc. using the PSQ96HS system (Pyrosequencing, Qiagen) according to standard procedures as described in previous work [Citation41,Citation42]. 500 ng of genomic DNA extracted from each sample was bisulfite treated using the EZ DNA Methylation Kit™ (Zymo Research, Irvine, CA, USA) and was purified according to the manufacturer’s protocol. The methylation level was determined using QCpG software (Pyrosequencing, Qiagen) and was reported as percent of DNA methylation for each CpG locus.
For replication analyzes, two CpG loci (CpG 3 (cg10564498) and CpG 5 (cg12722469)) were selected for replication based on results with the primary study population. We additionally selected another HM450 array targeting locus in the promoter region (CpG 4 (cg03247049)) to further investigate this region (). For HM450 assays, 700 to 1000 ng of genomic DNA from each sample was treated with bisulfite using the EZ-96 DNA Methylation Kit™ (Zymo Research, Irvine, CA, USA), according to the manufacturer’s recommended protocol and eluted in 18 µl. The Infinium HM450 data was processed as previously reported [Citation25], compiled for each locus and was expressed as beta (β) values. Methylation data was extracted for CpG 3, 4 and 5.
Genotyping
Details of genotyping assays, data processing and assessment of admixture were previously described [Citation43]. Genotypes of SNPs in AXL and its surrounding region (1 kb upstream and downstream) were extracted from the CHS genome-wide genotypic data. 28 tagged SNPs were identified with a pair tag r2 > 0.8 in Haploview using all available CHS samples (N = 3845) and were included in the analyzes [Citation44]. Admixture was assessed using the program STRUCTURE from a set of ancestral informative markers that were scaled to represent the proportion of African American, Asian, Native American and White [Citation45].
In silico analyses in publicly available data
To evaluate the association between AXL promoter methylation and mRNA expression, we downloaded AXL methylation profiling data in 29 histologically normal tissue samples from cases with lung adenocarcinoma (LUAD) or lung squamous cell carcinoma (LUSC) from the TCGA dataset [Citation46]. All samples had both methylation profiling (HM450 array) and RNA-seq (Illumina HiSeq) data. The mean age was 65.9 years (SD: 12.39) and 75.9% of the subjects were males. 51.7% of the subjects were moderate to heavy smokers. Promoter methylation average was calculated from average methylation of CpG 3 (cg10564498), CpG 4 (cg03247049) and CpG 5 (cg12722469). Spearman correlation coefficient was calculated to evaluate the correlation between promoter methylation and mRNA level.
R packages coMet and snp.plotter were used to graphically display additional information about CpGs, including genomic location and functional annotation from the Roadmap Epigenomics Project [Citation47–Citation49].
Statistical analyses
The methylation status of AXL promoter was defined as the average of CpG 1, 2, 3, 5 and 6 in the primary study population and the average of CpG 3, 4 and 5 in the replication population. Although defined differently for the two populations, these CpG sites are located in the same genomic region () and the correlations between them in the same population were relatively high (Tables S1 and S2).
To evaluate the association between AXL methylation and lung function growth from 10 to 18 years of age in the primary study population, all available pulmonary-function measurements for each subject were used to estimate lung function growth curves. We used a previously-reported linear spline model to account for the nonlinear pattern of growth during adolescence, with knots placed at ages 12, 14 and 16 years [Citation50,Citation51]. The model was fitted for each outcome and CpG individually and adjusted for child’s age, sex, sex*age interaction, ethnicity, height, height squared, body-mass index (BMI), BMI squared, city of residence at study entry, history of asthma, parental history of asthma, and maternal smoking during pregnancy. Random effects were included to account for multiple measurements contributed by each subject. Additional adjustment for AXL genetic polymorphisms, methylation plate, admixture, cohort, parental education level, second-hand smoke exposure, wheezing symptoms, personal smoking status, field technician, and preterm birth did not change the effect estimates by more than 10% and were removed from final models. Visual inspection of residual values did not identify any departures from model assumptions. Estimates of association between methylation with 8-year lung function development (from 10 to 18 years of age) and with mean attained lung function at both 10 and 18 were obtained. Sensitivity analyzes were conducted to evaluate the association in non-asthmatic subjects, defined as subjects who were never diagnosed with asthma before the last pulmonary function testing visit. To assess whether associations between lung function growth and methylation are modified by sex, an interaction term between sex and methylation was included in the regression models, and Wald tests were used to compute interaction p-values. Interaction between methylation and child’s ethnicity was also evaluated.
A similar mixed-effect linear spline model was used in the replication population, with knots placed at ages 12 and 14 years and the same adjustment variables. Further adjustment for estimated cord blood cell type proportions showed minimal effects and were not included in the model. Due to the limited availability of lung function measurements, the model was constructed to yield estimates of the association between methylation and 4-year lung function development (from 11 to 15 years of age). Effect modification by sex was also tested.
All tests assumed a two-sided alternative hypothesis and were conducted using SAS statistical analysis software (version 9.4). An alpha level of 0.05 was used to determine statistical significance.
Competing financial interests
The authors declare that they have no competing interests.
Author contributions
CB conceived and designed the study. CB, JM, KS, LD, and RU supervised the project. LG analyzed the data and wrote the manuscript. All authors edited and approved the manuscript.
Data availability
Please contact author for data requests.
Supplemental Material
Download MS Word (931.3 KB)Acknowledgments
We would like to express our sincere gratitude to Steve Graham and Robin Cooley at the California Biobank Program and Genetic Disease Screening Program within the California Department of Public Health for their assistance and advice regarding newborn bloodspots. The biospecimens and/or data used in this study were obtained from the California Biobank Program, (SIS request number(s) 479)” Section 6555(b), 17 CCR. The California Department of Public Health is not responsible for the results or conclusions drawn by the authors of this publication.
This research was supported by NIEHS grants 4R01ES022216, K01ES017801 and P30ES007048.
Disclosure statement
No potential conflict of interest was reported by the authors.
Supplementary material
Supplementary material data can be accessed here.
Additional information
Funding
References
- Knuiman MW, James AL, Divitini ML, et al. Lung function, respiratory symptoms, and mortality: results from the busselton health study. Ann Epidemiol. 1999 Jul;9(5):297–306. PubMed PMID: 10976856; eng
- Islam T, Gauderman WJ, Berhane K, et al. Relationship between air pollution, lung function and asthma in adolescents. Thorax. 2007 Nov;62(11):957–963. PubMed PMID: 17517830; PubMed Central PMCID: PMCPMC2117135. eng
- Fogarty AW, Jones S, Britton JR, et al. Systemic inflammation and decline in lung function in a general population: a prospective study. Thorax. 2007 Jun;62(6):515–520. PubMed PMID: 17251312; PubMed Central PMCID: PMCPMC2117221. eng
- Gold DR, Wang X, Wypij D, et al. Effects of cigarette smoking on lung function in adolescent boys and girls. N Engl J Med. 1996 Sep 26;335(13):931–937. PubMed PMID: 8782500; eng
- McClearn GE, Svartengren M, Pedersen NL, et al. Genetic and environmental influences on pulmonary function in aging Swedish twins. J Gerontol. 1994 Nov;49(6):264–268. PubMed PMID: 7963289; eng
- Urman R, McConnell R, Islam T, et al. Associations of children’s lung function with ambient air pollution: joint effects of regional and near-roadway pollutants. Thorax. 2014 Jun;69(6):540–547. PubMed PMID: 24253832; PubMed Central PMCID: PMCPMC4191894. eng
- Joubert BR, Haberg SE, Nilsen RM, et al. 450K epigenome-wide scan identifies differential DNA methylation in newborns related to maternal smoking during pregnancy. Environ Health Perspect. 2012 Oct;120(10):1425–1431. PubMed PMID: 22851337; PubMed Central PMCID: PMCPMC3491949. eng
- Perera F, Tang WY, Herbstman J, et al. Relation of DNA methylation of 5ʹ-CpG island of ACSL3 to transplacental exposure to airborne polycyclic aromatic hydrocarbons and childhood asthma. PLoS One. 2009;4(2):e4488. PubMed PMID: 19221603; PubMed Central PMCID: PMCPMC2637989. eng
- Lange NE, Sordillo J, Tarantini L, et al. Alu and LINE-1 methylation and lung function in the normative ageing study. BMJ Open. 2012;2(5). DOI:10.1136/bmjopen-2012-001231. PubMed PMID: 23075571; PubMed Central PMCID: PMCPMC3488751. eng
- Qiu W, Baccarelli A, Carey VJ, et al. Variable DNA methylation is associated with chronic obstructive pulmonary disease and lung function. Am J Respir Crit Care Med. 2012 Feb 15;185(4):373–381. PubMed PMID: 22161163; PubMed Central PMCID: PMCPMC3297093. eng
- Lepeule J, Baccarelli A, Motta V, et al. Gene promoter methylation is associated with lung function in the elderly: the normative aging study. Epigenetics. 2012 Mar;7(3):261–269. PubMed PMID: 22430802; PubMed Central PMCID: PMCPMC3335949. eng
- Golbabapour S, Abdulla MA, Hajrezaei M. A concise review on epigenetic regulation: insight into molecular mechanisms. Int J Mol Sci. 2011;12(12):8661–8694. PubMed PMID: 22272098; PubMed Central PMCID: PMCPMC3257095. eng
- Breton CV, Byun HM, Wenten M, et al. Prenatal tobacco smoke exposure affects global and gene-specific DNA methylation. Am J Respir Crit Care Med. 2009 Sep 1;180(5):462–467. PubMed PMID: 19498054; PubMed Central PMCID: PMCPMC2742762. eng
- Breton CV, Salam MT, Gilliland FD. Heritability and role for the environment in DNA methylation in AXL receptor tyrosine kinase. Epigenetics. 2011 Jul;6(7):895–898. PubMed PMID: 21555911; PubMed Central PMCID: PMCPMC3230541. eng
- Park IK, Giovenzana C, Hughes TL, et al. The Axl/Gas6 pathway is required for optimal cytokine signaling during human natural killer cell development. Blood. 2009 Mar 12;113(11):2470–2477. [pii]. PubMed PMID: 18840707; PubMed Central PMCID: PMC2656272. eng.
- Rothlin CV, Ghosh S, Zuniga EI, et al. TAM receptors are pleiotropic inhibitors of the innate immune response. Cell. 2007 Dec 14;131(6):1124–1136. PubMed PMID: 18083102; eng
- Seitz HM, Camenisch TD, Lemke G, et al. Macrophages and dendritic cells use different Axl/Mertk/Tyro3 receptors in clearance of apoptotic cells. J Immunol. 2007 May 01;178(9):5635–5642. PubMed PMID: 17442946; eng
- Fujimori T, Grabiec AM, Kaur M, et al. The Axl receptor tyrosine kinase is a discriminator of macrophage function in the inflamed lung. Mucosal Immunol. 2015 Sep;8(5):1021–1030. PubMed PMID: 25603826; PubMed Central PMCID: PMCPMC4430298. eng
- Grabiec AM, Denny N, Doherty JA, et al. Diminished airway macrophage expression of the Axl receptor tyrosine kinase is associated with defective efferocytosis in asthma. J Allergy Clin Immunol. 2017 Apr;12(140):1144–1146.e4. PubMed PMID: 28412392; eng
- Demedts IK, Demoor T, Bracke KR, et al. Role of apoptosis in the pathogenesis of COPD and pulmonary emphysema. Respir Res. 2006 Mar;30(7):53. PubMed PMID: 16571143; PubMed Central PMCID: PMCPMC1501017. eng
- McConnell R, Berhane K, Yao L, et al. Traffic, susceptibility, and childhood asthma. Environ Health Perspect. 2006 May;114(5):766–772. PubMed PMID: 16675435; PubMed Central PMCID: PMCPMC1459934. eng
- Peters JM, Avol E, Gauderman WJ, et al. A study of twelve Southern California communities with differing levels and types of air pollution. II. Effects on pulmonary function. Am J Respir Crit Care Med. 1999 Mar;159(3):768–775. PubMed PMID: 10051249; eng
- Peters JM, Avol E, Navidi W, et al. A study of twelve Southern California communities with differing levels and types of air pollution. I. Prevalence of respiratory morbidity. Am J Respir Crit Care Med. 1999 Mar;159(3):760–767. PubMed PMID: 10051248; eng
- Berhane K, McConnell R, Gilliland F, et al. Sex-specific effects of asthma on pulmonary function in children. Am J Respir Crit Care Med. 2000 Nov;162(5):1723–1730. PubMed PMID: 11069803; eng
- Gao L, Millstein J, Siegmund KD, et al. Epigenetic regulation of AXL and risk of childhood asthma symptoms. Clin Epigenetics. 2017;9:121. PubMed PMID: 29177020; PubMed Central PMCID: PMCPMC5688797. eng
- Lemke G, Rothlin CV. Immunobiology of the TAM receptors. Nat Rev Immunol. 2008 May;8(5):327–336. PubMed PMID: 18421305; PubMed Central PMCID: PMCPMC2856445. eng
- Holland SJ, Pan A, Franci C, et al. R428, a selective small molecule inhibitor of Axl kinase, blocks tumor spread and prolongs survival in models of metastatic breast cancer. Cancer Res. 2010 Feb 15;70(4):1544–1554. PubMed PMID: 20145120; eng
- Healy AM, Schwartz JJ, Zhu X, et al. Gas 6 promotes Axl-mediated survival in pulmonary endothelial cells. Am J Physiol Lung Cell Mol Physiol. 2001 Jun;280(6):L1273–L81. PubMed PMID: 11350808; eng
- Hussell T, Bell TJ. Alveolar macrophages: plasticity in a tissue-specific context. Nat Rev Immunol. 2014 Feb;14(2):81–93. PubMed PMID: 24445666; eng
- Grabiec AM, Hussell T. The role of airway macrophages in apoptotic cell clearance following acute and chronic lung inflammation. Semin Immunopathol. 2016 Jul;38(4):409–423. PubMed PMID: 26957481; PubMed Central PMCID: PMCPMC4896990. eng
- Sin DD, Wu L, Man SF. The relationship between reduced lung function and cardiovascular mortality: a population-based study and a systematic review of the literature. Chest. 2005 Jun;127(6):1952–1959. PubMed PMID: 15947307; eng
- Tager IB, Segal MR, Speizer FE, et al. The natural history of forced expiratory volumes. effect of cigarette smoking and respiratory symptoms. Am Rev Respir Dis. 1988 Oct;138(4):837–849. PubMed PMID: 3202458; eng
- Mudduluru G, Allgayer H. The human receptor tyrosine kinase Axl gene–promoter characterization and regulation of constitutive expression by Sp1, Sp3 and CpG methylation. Biosci Rep. 2008 Jun;28(3):161–176. PubMed PMID: 18522535; eng
- Carithers LJ, Moore HM. The genotype-tissue expression (GTEx) project. Biopreserv Biobank. 2015 Oct;13(5):307–308. PubMed PMID: 26484569; PubMed Central PMCID: PMCPMC4692118. eng
- Yang AS, Estécio MR, Doshi K, et al. A simple method for estimating global DNA methylation using bisulfite PCR of repetitive DNA elements. Nucleic Acids Res. 2004 Feb 18;32(3):e38. PubMed PMID: 14973332; PubMed Central PMCID: PMCPMC373427. eng
- Khoo SK, Dykema K, Vadlapatla NM, et al. Acquiring genome-wide gene expression profiles in Guthrie card blood spots using microarrays. Pathol Int. 2011 Jan;61(1):1–6. PubMed PMID: 21166936; eng
- Hollegaard MV, Grauholm J, Norgaard-Pedersen B, et al. DNA methylome profiling using neonatal dried blood spot samples: a proof-of-principle study. Mol Genet Metab. 2013 Apr;108(4):225–231. PubMed PMID: 23422032; eng
- Aberg KA, Xie LY, Nerella S, et al. High quality methylome-wide investigations through next-generation sequencing of DNA from a single archived dry blood spot. Epigenetics. 2013 May;8(5):542–547. PubMed PMID: 23644822; PubMed Central PMCID: PMCPMC3741224. eng
- Hollegaard MV, Grove J, Grauholm J, et al. Robustness of genome-wide scanning using archived dried blood spot samples as a DNA source. BMC Genet. 2011 Jul;4(12):58. PubMed PMID: 21726430; PubMed Central PMCID: PMCPMC3142526. eng
- Dratva J, Breton CV, Hodis HN, et al. Birth weight and carotid artery intima-media thickness. J Pediatr. 2013 May;162(5):906–11.e1–2. PubMed PMID: 23260106; PubMed Central PMCID: PMCPMC4030536. eng
- Tost J, Dunker J, Gut IG. Analysis and quantification of multiple methylation variable positions in CpG islands by Pyrosequencing. Bio Tech. 2003 Jul;35(1):152–156. PubMed PMID: 12866415; eng
- Brakensiek K, Wingen LU, Langer F, et al. Quantitative high-resolution CpG island mapping with Pyrosequencing reveals disease-specific methylation patterns of the CDKN2B gene in myelodysplastic syndrome and myeloid leukemia. Clin Chem. 2007 Jan;53(1):17–23. PubMed PMID: 17095538; eng
- Breton CV, Yao J, Millstein J, et al. Prenatal air pollution exposures, DNA methyl transferase genotypes, and associations with newborn LINE1 and alu methylation and childhood blood pressure and carotid intima-media thickness in the children’s health study. Environ Health Perspect. 2016 Dec;124(12):1905–1912. PubMed PMID: 27219456; eng
- Barrett JC, Fry B, Maller J, et al. Haploview: analysis and visualization of LD and haplotype maps. Bioinformatics. 2005 Jan 15;21(2):263–265. PubMed PMID: 15297300; Eng
- Pritchard JK, Stephens M, Donnelly P. Inference of population structure using multilocus genotype data. Genetics. 2000 Jun;155(2):945–959. PubMed PMID: 10835412; PubMed Central PMCID: PMCPMC1461096. Eng
- The Cancer Genome Atlas 2009 [ cited 2017 Mar 20]. Available from: https://cancergenome.nih.gov/
- Luna A, Nicodemus KK. snp.plotter: an R-based SNP/haplotype association and linkage disequilibrium plotting package. Bioinformatics. 2007 Mar 15;23(6):774–776. PubMed PMID: 17234637; eng
- Martin TC, Yet I, Tsai PC, et al. coMET: visualisation of regional epigenome-wide association scan results and DNA co-methylation patterns. BMC Bioinf. 2015 Apr;28(16):131. PubMed PMID: 25928765; PubMed Central PMCID: PMCPMC4422463. eng
- Bernstein BE, Stamatoyannopoulos JA, Costello JF, et al. The NIH roadmap epigenomics mapping consortium. Nat Biotechnol. 2010 Oct;28(10):1045–1048. PubMed PMID: 20944595; PubMed Central PMCID: PMCPMC3607281. eng
- Gauderman WJ, Vora H, McConnell R, et al. Effect of exposure to traffic on lung development from 10 to 18 years of age: a cohort study. Lancet (London, England). 2007 Feb 17;369(9561):571–577. PubMed PMID: 17307103; eng
- Gilliland F, Avol E, McConnell R, et al. The effects of policy-driven air quality improvements on children’s respiratory health. (Research Report I90). Boston, MA: Health Effects Institute 2017.