ABSTRACT
Although central to regulating the access to genetic information, most lysine methyltransferases remain poorly characterised relative to other family of enzymes. Herein, I report new substrates for the lysine methyltransferase SETD6. Based on the SETD6-catalysed site on the histone variant H2AZ, I identified similar sequences in the canonical histones H2A, H3, and H4 that are modified by SETD6 in vitro, and putative non-histone substrates. I herein expend the repertoire of substrates for methylation by SETD6.
Introduction
Histone H3 lysine residues were found to be methylated over fifty years ago [Citation1,Citation2]. However, it was not until 2000 that the first mammalian histone lysine methyltransferase was discovered [Citation3]. The latest human genome annotation predicts over 60 lysine methyltransferases (KMTs) based on sequence similarities with either a SET or a seven β-strand catalytic domain [Citation4]. However, most of these enzymes remain uncharacterized or poorly studied. Thus, important questions regarding the biological relevance and biochemical properties of these enzymes remain unanswered. Importantly, several histone KMTs also methylate non-histone substrates, such as the tumour suppressors p53 [Citation5–Citation7], ING2 [Citation8], and pRB [Citation9], as well as chromatin proteins such as DNMT1 [Citation10], HP1α/β/γ [Citation11], RUVBL1 [Citation12], and RUVBL2 [Citation13].
The methyltransferase SETD6 mono-methylates the NFκB subunit RelA at lysine 310 (K310me1) [Citation14], the histone variant H2AZ at lysine 7 (K7me1) [Citation15], and the kinases PAK4 [Citation16], and PLK1 [Citation17]. The expression of SETD6 is amplified in about 10% of cases of breast cancer according to a study using a patient xenograft model [Citation18] and is required for cellular proliferation in both ER+ and ER− breast cancer cell models [Citation19], suggesting an important role in driving breast cancer progression. Indeed, SETD6 was recently found to associate with the cytoskeleton protein VIM [Citation20], which is involved in epithelial to mesenchymal transition (EMT), cellular attachment, migration, and signalling, suggesting a role in metastasis.
Much like classical signal transduction events involve phospho-dependent protein-protein binding, chromatin signalling events implicate post-translational modifications in the regulation of macro-molecules interactions. For example, lysine methylation of histones lysine residues serves as landing pads for chromatin proteins, which are referred to as histone mark readers or simply readers, thereby nucleating enzymatic complexes that modify and remodel chromatin to regulate access to genetic information.
Herein, I demonstrate that recombinant SETD6 methylates canonical histones H2A, H3, and H4, as well as linker histones H1 and the non-histone protein ING2 in vitro, and identify several putative novel substrates, including chromatin proteins and other lysine methyltransferases.
Results
I previously identified 2 mono-methylation sites on the histone variant H2AZ catalysed by SETD6 [Citation15]. Interestingly, these modified sites, H2AZK4me1 and H2AZK7me1, are similar. Both modified lysine residues are preceded by a small amino acid (alanine or glycine) residue at position −2 and a glycine at position −1 (). Examination of canonical histone tails revealed similar sequences in histones H2A, H3, and H4, and highlighted a putative SETD6 methylation consensus motif A/G/RGKme1A/GG ().
Table 1. SETD6 consensus motif. Based on H2AZ methylation sites by SETD6, putative modification sites were identified in canonical histones H2A, H3, and H4.
To test whether SETD6 could methylate these other histones, I used a mixture of purified calf thymus histones as substrates. Interestingly, SETD6 was capable of modifying the linker H1 histones as well as the canonical histones H2A, H3, and H4 (). As a positive control, I used SET7, which is known to methylate H3 [Citation21], and H1 histones [Citation22]. As a negative control, GST alone was used and as expected GST had no detectable methyltransferase activity on histones ().
Figure 1. SETD6 methylates histones. Recombinant KMTs were used to modify histones isolated from calf thymus in the presence of 3H-SAM. Samples were analysed by SDS-PAGE, then either stained with Coomassie (top panel) or transferred to PVDF membrane and autoradiographed (bottom panel).
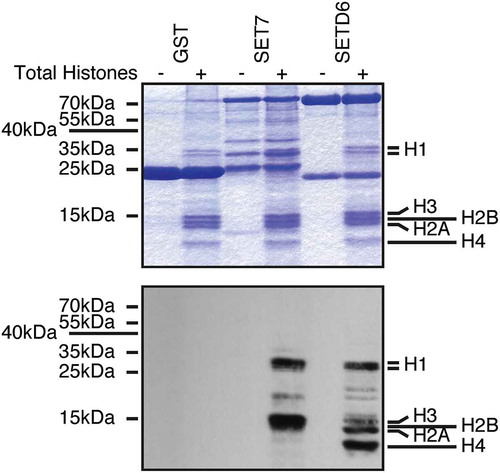
To confirm the SETD6-catalysed methylation sites on canonical histones, the first 50 amino acid residues of H2A, H3, and H4 were fused to the amino terminus of GST to leave the histone tail free at the amino terminus and generate H2A1-50-GST, H31-50-GST, and H41-50-GST. Then the predicted sites () were converted to arginine by site-directed mutagenesis. The affinity purified recombinant proteins were then used for in vitro KMT assays with SETD6. In agreement with previous experiments showing that SETD6 modifies canonical histones (), SETD6 methylated recombinant histone tails from H2A, H3, and H4 (). Importantly, mutation of the GK motifs reduced drastically the methylation of H2A and H3 by SETD6 (). However, single mutation of H4 at K5 or K12 only minimally reduced methylation by SETD6 (), suggesting that both K5 and K12 are modified.
Figure 2. SETD6 methylates GK motifs in canonical histones. (a) SETD6 was used to methylate the indicated recombinant histone tails. Samples were analysed by SDS-PAGE, then either stained with Coomassie (top panel) or transferred to PVDF membrane and autoradiographed (bottom panel). (b) KMT assays were performed with SETD6 on H4 peptides. (c) Sequence of H4 peptides used in panel B with each lysine numbered.
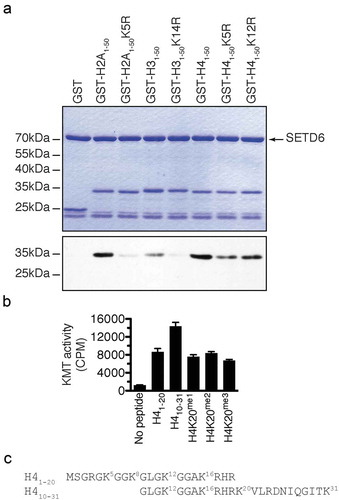
Since H4 methylation seemed stronger (), and to further investigate the methylation of H4 by SETD6, methyltransferase assays on H4 peptides H41-20 and H410-31 were performed and confirmed that SETD6 methylates H4 (). Interestingly, SETD6 methylated H410-31 better than the H41-20 peptide, while methylation at K20 (K20me1, K20me2, or K20me3) impaired this effect (), suggesting that SETD6 modifies H4K20 in addition to H4K12 or other site(s), such as H4K16 or H4K31 (). Alternatively, these results may suggest that there is a cross-talk between H4K20me and the SETD6-catalysed methylation site(s).
Several KMT, including SETD6, modify non-histone proteins. I thus searched for the GKDS motif in protein sequence repositories and identified several putative SETD6 substrates ( and S1), including the ATPase RUVBL1, which is modified by the H3K9 methyltransferases G9A and GLP [Citation12]. Importantly, some putative SETD6 substrates (AHNAK2, ERICH3, and MDN1) were found in the PhosphoSitePlus mass spectrometric database to be methylated at the predicted site [Citation23] (Table S1). A similar search using the H4K5 and H4K12 motif GKGG also yielded several putative substrates for SETD6, such as the chromatin remodeller BRG1 (GK1029GG) and the HBO1 acetyltransferase subunit JADE2 (GK637GG).
Table 2. Novel SETD6 putative substrates. The GKDS motif from H2AZ was used to identify novel substrates for SETD6.
Herein, I found that SETD6 methylates histones H2A, H3, and H4 on lysine residues within an A/G/RGKme1A/GG consensus motif.
Discussion
Proteomic studies have identified post-translational modifications on histones and non-histone proteins, but the enzymatic activities depositing these modifications remain largely unknown. There is a dire need to identify PTMs to understand how proteins are regulated, but more importantly to identify the enzymes catalysing these biochemical events. To this end, I have in the past designed an unbiased chemical-biology approach to tag novel KMT substrates [Citation24]. However, traditional biochemical studies are still required to investigate and validate novel post-translational modifications.
The H3K14me1 mark was reported to occur in both human and mouse [Citation25,Citation26], supporting the existence of the modification in cells. I have herein identified the first KMT capable of modifying this site in vitro. Further work will be required to validate the role of SETD6 in the catalysis of H3K14me1 in cells and the function of this mark.
Interestingly, H4K12me3 [Citation23], H4K16me3 [Citation23,Citation26], and H4K31me1 [Citation27] were detected by mass spectrometry. However, no other reference to these modifications appear in the current literature. Interestingly, H4K5 was recently found to be methylated by SMYD3 [Citation28].
Although mono-methylation events on the linker histone H1 H1F0 was reported at K12, K59, K82, K102, K108, and K155 [Citation23,Citation29], these sites do not share similarities with the SETD6 consensus GK motif, suggesting that SETD6 modifies non-GKDS sequences. Indeed, SETD6 methylates RelA at the non-GKDS site FK310SI [Citation14]. In addition, SETD6 can methylate the histone mark reader ING2 in vitro (Figure S1), PAK4 [Citation16], and PLK1 [Citation17], all of which do not contain any GKDS-like motif.
Structurally, the GK motif on AHR is not within a particular functional domain, while the putative methylation sites on CDKN2C and KDM5A are found at the very end of their unstructured carboxy terminus, while the GK motif of SMYD4 is found near the amino terminus. These observations lead to conclude that the GK motifs found on the putative SETD6 non-histone substrates could be available for modification.
Together, the in vitro data provided here identifies SETD6 as a likely candidate for the methylation of reported events on H2AK5 [Citation30], H3K14 [Citation25,Citation26], H4K5 [Citation28,Citation31], H4K12, H4K16, and H4K31. In addition, based on sequence similarities I have identified several putative non-histone protein substrates for SETD6.
Methods
Plasmids
The modified pGEX plasmid with an engineered multi-cloning site at the N-terminus of the GST coding sequence was described previously [Citation15]. The cDNA of histones H2A, H3, and H4 was amplified by PCR from reverse transcribed total RNA and inserted in frame with GST using restriction endonucleases and T4 DNA ligase (NEB).
Recombinant protein expression and purification
Essentially, BL21 DE3 competent bacteria (Stratagene) were transformed with pGEX plasmids. Single colonies were picked and grown in 2YT media. Expression of GST-fusion proteins was induced with 0.01 mM IPTG for 2.5 hours at 37°C, cells were collected and lysed in buffer (50 mM Tris-Cl pH 7.5, 150 mM NaCl, 0.05% NP-40). Recombinant protein were batch purified using glutathione-sepharose beads. GST-SETD6 was purified similarly, but from Sf9 insect cells as described [Citation15].
In vitro KMT and flashplate KMT assays
Lysine methylation assays were performed in reaction buffer (50 mM Tris-Cl pH 8.0, 10% glycerol, 20 mM KCl, 5 mM MgCl2) supplemented with 3H-SAM as described [Citation8], using calf thymus histones (Worthington), recombinant histone tails (see above), or biotinylated histone H4 peptides.
Motif search
The GKDS sequence was used in a motif search using PHI-BLAST against the Non-redundant protein sequences (nr) database, restricted to Homo sapiens (taxid:9606).
Correction Statement
This article has been republished with minor changes. These changes do not impact the academic content of the article.
Supplemental Material
Download PDF (40.4 KB)Supplemental Material
Download TIFF Image (4.9 MB)Acknowledgments
OB was supported by the Newcastle’s Biomedical Fellowship Programme, which is in part funded through the Wellcome Trust’s Institutional Strategic Support Fund, and by the Breast Cancer Campaign charity grant number 2013MaySP005.
Disclosure statement
No potential conflict of interest was reported by the author.
Supplementary material
Supplemental data for this article can be accessed here.
Additional information
Funding
References
- Allfrey V, Faulkner R, Mirsky A. Acetylation and methylation of histones and their possible role in the regulation of RNA synthesis. Proc Natl Acad Sci USA. 1964;51(5):786–794.
- Murray K. The occurrence of e-N-methyl lysine in histones. Biochemistry. 1964;3:10–15.
- Rea S, Eisenhaber F, O’Carroll D, et al. Regulation of chromatin structure by site-specific histone H3 methyltransferases. Nature. 2000;406(6796):593–599.
- Binda O. On your histone mark, SET, methylate!. Epigenetics. 2013;8(5):457–463.
- Chuikov S, Kurash JK, Wilson JR, et al. Regulation of p53 activity through lysine methylation. Nature. 2004;432(7015):353–360.
- Huang J, Perez-Burgos L, Placek BJ, et al. Repression of p53 activity by Smyd2-mediated methylation. Nature. 2006;444(7119):629–632.
- Shi X, Kachirskaia I, Yamaguchi H, et al. Modulation of p53 function by SET8-mediated methylation at lysine 382. Mol Cell. 2007;27(4):636–646.
- Binda O, LeRoy G, Bua DJ, et al. Trimethylation of histone H3 lysine 4 impairs methylation of histone H3 lysine 9: regulation of lysine methyltransferases by physical interaction with their substrates. Epigenetics. 2010;5(8):767–775.
- Saddic LA, West LE, Aslanian A, et al. Methylation of the retinoblastoma tumor suppressor by SMYD2. J Biol Chem. 2010;285(48):37733–37740.
- Estève P-O, Chin HG, Benner J, et al. Regulation of DNMT1 stability through SET7-mediated lysine methylation in mammalian cells. Proc Natl Acad Sci USA. 2009;106(13):5076–5081.
- LeRoy G, Weston JT, Zee BM, et al. Heterochromatin protein 1 is extensively decorated with histone code-like post-translational modifications. Mol Cell Proteomics. 2009;8(11):2432–2442.
- Lee JS, Kim Y, Bhin J, et al. Hypoxia-induced methylation of a pontin chromatin remodeling factor. Proc Natl Acad Sci USA. 2011;108(33):13510–13515.
- Lee JS, Kim Y, Kim IS, et al. Negative regulation of hypoxic responses via induced reptin methylation. Mol Cell. 2010;39(1):71–85.
- Levy D, Kuo AJ, Chang Y, et al. Lysine methylation of the NF-κB subunit RelA by SETD6 couples activity of the histone methyltransferase GLP at chromatin to tonic repression of NF-κB signaling. Nat Immunol. 2011;12(1):29–36.
- Binda O, Sevilla A, LeRoy G, et al. SETD6 monomethylates H2AZ on lysine 7 and is required for the maintenance of embryonic stem cell self-renewal. Epigenetics. 2013;8(2):177–183.
- Vershinin Z, Feldman M, Chen A, et al. PAK4 methylation by SETD6 promotes the activation of the Wnt/beta-catenin pathway. J Biol Chem. 2016;291(13):6786–6795.
- Feldman M, Vershinin Z, Goliand I, et al. The methyltransferase SETD6 regulates mitotic progression through PLK1 methylation. Proc Natl Acad Sci USA. 2019;116(4):1235–1240.
- Eirew P, Steif A, Khattra J, et al. Dynamics of genomic clones in breast cancer patient xenografts at single-cell resolution. Nature. 2015;518(7539):422–426.
- O’Neill DJ, Williamson SC, Alkharaif D, et al. SETD6 controls the expression of estrogen-responsive genes and proliferation of breast carcinoma cells. Epigenetics. 2014;9(7):942–950.
- Cohn O, Chen A, Feldman M, et al. Proteomic analysis of SETD6 interacting proteins. Data in brief. 2016;6:799–802.
- Wang H, Cao R, Xia L, et al. Purification and functional characterization of a histone H3-lysine 4-specific methyltransferase. Mol Cell. 2001;8(6):1207–1217.
- Kassner I, Barandun M, Fey M, et al. Crosstalk between SET7/9-dependent methylation and ARTD1-mediated ADP-ribosylation of histone H1.4. Epigenetics Chromatin. 2013;6(1):1.
- Hornbeck PV, Kornhauser JM, Tkachev S, et al. PhosphoSitePlus: a comprehensive resource for investigating the structure and function of experimentally determined post-translational modifications in man and mouse. Nucleic Acids Res. 2012;40(Database issue):D261–270.
- Binda O, Boyce M, Rush JS, et al. A chemical method for labeling lysine methyltransferase substrates. ChemBioChem. 2011;12(2):330–334.
- Bonenfant D, Towbin H, Coulot M, et al. Analysis of dynamic changes in post-translational modifications of human histones during cell cycle by mass spectrometry. Mol Cell Proteomics. 2007;6(11):1917–1932.
- Tweedie-Cullen RY, Reck JM, Mansuy IM. Comprehensive mapping of post-translational modifications on synaptic, nuclear, and histone proteins in the adult mouse brain. J Proteome Res. 2009;8(11):4966–4982.
- Lee YJ, Rice RH, Lee YM. Proteome analysis of human hair shaft: from protein identification to posttranslational modification. Mol Cell Proteomics. 2006;5(5):789–800.
- Van Aller GS, Reynoird N, Barbash O, et al. Smyd3 regulates cancer cell phenotypes and catalyzes histone H4 lysine 5 methylation. Epigenetics. 2012;7(4):340–343.
- Lu A, Zougman A, Pudelko M, et al. Mapping of lysine monomethylation of linker histones in human breast and its cancer. J Proteome Res. 2009;8(9):4207–4215.
- Wu Z, Cheng Z, Sun M, et al. A chemical proteomics approach for global analysis of lysine monomethylome profiling. Mol Cell Proteomics. 2015;14(2):329–339.
- Green EM, Mas G, Young NL, et al. Methylation of H4 lysines 5, 8 and 12 by yeast Set5 calibrates chromatin stress responses. Nat Struct Mol Biol. 2012;19(3):361–363.