ABSTRACT
Methylation levels at the hypoxia-inducible factor 3α gene (HIF3A) in blood have been linked to body mass index (BMI) in adults. Despite evidence implicating HIF3A in angiogenesis and metabolism, no studies have examined links between HIF3A methylation in early life and cardiovascular health. Here, we investigated the relationship between HIF3A methylation in blood at birth and 12 months of age with cardiovascular measures at 4 years. We also examined influences of prenatal exposures, birth outcomes, and genetic variation. Methylation of two HIF3A promoter regions in cord blood was measured using Sequenom EpiTYPER mass-spectrometry. The first promoter region was also measured in 12-month blood. Four-year cardiovascular measures included blood pressure, pulse wave velocity, and aortic and carotid intima-media thickness. Associations were tested using partial correlation tests and linear regression modelling. Methylation of the first HIF3A promoter in cord and 12-month blood was not associated with four-year measures. There was modest evidence of an association between DNA methylation at the second HIF3A promoter in cord blood and four-year systolic blood pressure (n = 353, r = 0.12, p = 0.03). In sex-stratified analysis, methylation of the second promoter was modestly associated with systolic and diastolic blood pressure (r = 0.16, p = 0.03 for both) in males only. In conclusion, HIF3A methylation at birth shows some evidence of an association with later blood pressure in childhood. Further work should determine whether this relationship persists into later childhood, and should assess potential functional links between HIF3A methylation and cardiovascular health more generally.
Background
The trajectory towards adult metabolic and cardiovascular health begins very in early life, with evidence for a variety of environmental exposures in childhood impacting disease risk in adulthood [Citation1]. Environmental exposures in early life can influence childhood cardiovascular health, which in turn has been linked to later risk of cardiovascular disease [Citation2,Citation3]. Elevated blood pressure in childhood is associated with increased risk of hypertension, metabolic syndrome [Citation4] and altered heart structure [Citation5] in adulthood. Further, intima-media thicknesses of the aortic and carotid vessels in childhood have been used as measures of preclinical atherosclerosis [Citation6,Citation7]. Evidence from animal models suggests these associations may be influenced by sex [Citation8], but evidence from humans is less compelling [Citation9]. Emerging data suggest that epigenetics plays a role in the ‘biological embedding’ of later life risk following early life exposures [Citation10], and attention has recently turned to identifying genes where methylation levels in early life may predict later cardiovascular health [Citation11].
Hypoxia-inducible factor 3α (HIF3A), encoded by the HIF3A gene, is part of a family of proteins that play a key role in angiogenesis, metabolism, and obesity [Citation12]. DNA methylation of one promoter of the HIF3A gene in adult blood has been reproducibly linked to body mass index (BMI) [Citation13], and more recently the link between HIF3A methylation at the same region and BMI has been investigated in childhood [Citation14,Citation15]. However, most paediatric studies have focussed on early life associations rather than longitudinal associations between methylation and later phenotypes. An exception is a study that measured DNA methylation at this region at birth, age 7 and 17 years and examined associations with BMI at 7 and 17 years of age [Citation15]. This study suggested that birth weight and BMI at 7 years were associated with later HIF3A methylation in blood at 7 and 17 years of age, respectively. The same study also reported evidence of a link between maternal pre-pregnancy BMI and HIF3A methylation levels at a second promoter region in cord blood. More recently, we found evidence that gestational diabetes (GDM), pre-eclampsia, infant sex, gestational age, and HIF3A genetic variation all independently associated with different HIF3A methylation levels at this second promoter region in cord blood [Citation16].
At present, there are no data on whether HIF3A methylation in early life is linked with cardiovascular phenotypes in children or adults. However, given the evidence for a link between pregnancy exposures we identified previously [Citation16] and cardiovascular health in offspring, specifically exposure to pre-eclampsia with elevated systolic blood pressure [Citation17] and risk of stroke [Citation18], and exposure to GDM with risk of cardiovascular-related hospitalizations [Citation19], we hypothesized that early life methylation of HIF3A is associated with later cardiovascular development in childhood.
Here, we investigated if HIF3A promoter methylation (two regions, HIF3A.1 and HIF3A.2) in blood at birth and 12 months of age associated with BMI and/or measures of cardiovascular health at 4 years. We also considered whether specific prenatal exposures and birth outcomes, previously associated with HIF3A methylation and HIF3A genetic variation, might confound these relationships.
Methods
Study cohort – Barwon Infant Study
We used samples from the Barwon Infant Study (BIS), a population-based pre-birth cohort (n = 1074), with maternal clinical data from pregnancy, infant outcomes at birth, and cardiovascular measures at 4 years of age. The BIS protocol was approved by the Barwon Health Human Research Ethics Committee (HREC 10/24), and mothers provided written informed consent. The details on eligibility, recruitment, and retention have been described previously [Citation20].
Primary outcome – cardiovascular development at four years of age
Cardiovascular measures were taken during the participant’s 4-year review and included measurement of weight, height, blood pressure, heart rate, and pulse wave velocity, as well as the measurement of aortic and carotid intima-media thicknesses (aIMT and cIMT, respectively) following ultrasound imaging using the GE Vivid-I (GE Healthcare), with an intra-reader intra-class correlation (ICC) of 0.92 and inter-reader ICC of 0.90, as previously described [Citation21]. Measured weight and height-squared were used to calculate BMI. Brachial blood pressure, heart rate, and pulse wave velocity were averaged across three readings in a resting, supine position using SphygmoCor XCEL (AtCor Medical). The means for aIMT and cIMT were calculated from five images. Tests with mean aIMT were also adjusted for aortic diameter. Data availability for each measurement in this study is shown in . For analysis, tests including blood pressure measures were also adjusted for actual child age, height, and sex.
Table 1. Cohort characteristics for the full sample (any BIS infant with both any methylation data and any four-year measure), and the sex-stratified sample
Primary exposure – early life blood HIF3A methylation
DNA was extracted from cord and 12-month whole blood using the QIAamp DNA QIAcube HT Kit (QIAGEN, Hilden, Germany) according to manufacturer’s instructions and stored at −80°C. Bisulphite conversion of DNA was performed with the MagPrep Lightning Conversion Kit (Zymo Research, Irvine, CA, USA). DNA methylation in two promoter regions of HIF3A was measured using the locus-specific Sequenom EpiTYPER mass-spectrometry platform (Agena Bioscience) as described previously [Citation16]. Methylation at HIF3A.1 (hg38:chr19:46,298,243–46,298,580), previously linked to BMI in adults [Citation13], was measured in a subset of cord blood (n = 490) and 12-month whole blood samples (n = 538). Methylation at HIF3A.2 (hg38:chr19:46,303,864–46,304,196), associated with maternal pre-pregnancy BMI [Citation15], was measured in cord blood for all available samples (n = 938). The EpiTYPER platform utilizes a process of reverse transcription and cleavage of the assayed region to create fragments (referred to here as ‘CpG units’), each of which contains 1–4 CpG sites (the majority contain 1 CpG site). The resulting methylation level represents the average proportion of methylation across all CpG sites on each CpG unit. The CpG units measured in each region are listed in Supp. Table 1.
As methylation at each CpG unit within each region was strongly correlated [Citation16], the average methylation across each region was used as the main exposure measure, and individual CpG unit methylation was considered in sensitivity analysis. Participants with missing methylation data for any of the CpG units were excluded from the average methylation analysis.
To assess possible cellular heterogeneity in blood samples, flow cytometry (FACsCalibur, Becton Dickinson) was used to characterize the cellular composition of blood samples as described previously [Citation22]. The proportions of monocytes, granulocytes, and lymphocytes were considered in the sensitivity analysis.
Other factors: pregnancy heath, child genetics and birth outcomes
Infant birth weight (z-score, adjusted for gestational age and sex [Citation23]), sex and gestational age were considered as covariates. As there is evidence for maternal pre-pregnancy BMI, gestational diabetes and pre-eclampsia impacting both offspring HIF3A methylation [Citation15,Citation16,Citation24] and offspring cardiovascular health [Citation17,Citation19], these were considered as potential confounders. Pre-pregnancy BMI was calculated from self-reported weight, and gestational diabetes and pre-eclampsia were defined using standard clinical criteria [Citation25,Citation26]. Socioeconomic status, measured using Socio-Economic Indexes For Areas (SEIFA) [Citation27] and grouping mothers into tertiles, and maternal age were also considered as potential confounding factors.
Genome-wide genotyping and imputation were performed on all BIS infants as described previously [Citation16]. After quality control, genotypes were available for 261 common SNPs (minor allele frequency >0.01) in and near the HIF3A gene (hg38: chr19:46,278,743–46,361,743). A total of 14 tag SNPs, identified with the HaploView software (Broad Institute), were used as proxies for clusters of associated genetic variation (r2 > 0.1) in analysis. There is previous evidence for several of these SNPs associating with HIF3A methylation levels, particularly HIF3A.2 methylation [Citation16].
Statistical analysis
A flowchart of participant inclusion in this analysis, and the number of participants with any cardiovascular phenotype for each of the methylation measures, is shown in . The exact number of participants included in each test is shown in the corresponding results tables.
Pearson’s correlation coefficients were calculated for the pairwise correlations of all methylation measures (both average methylation across each region and individual CpG unit methylation). Preliminary analysis used partial correlation tests to identify potential associations of interest between HIF3A.1 and HIF3A.2 methylation in infancy and four-year weight and cardiovascular outcomes. All tests were adjusted for actual age in years at the four-year time point and Sequenom batch, as well as the actual age in months at the 12-month time point for 12-month methylation associations. To consider potential sex-specific associations, analyses were additionally stratified by sex. The associations of interest from in the initial analysis were then investigated further in linear regression models for adjustment of birth weight, 4-year BMI, and potential confounders (above). The final model included covariates which were demonstrated to alter the effect size of methylation (>10% change in coefficient) or improve the model fit (likelihood ratio test p < 0.05). Genotypes at each of the 14 tag SNPs were considered as covariates. P-values are presented unadjusted for multiple comparisons.
For sensitivity analysis, associations between methylation of individual CpG units in each region and 4-year cardiovascular measure were considered. In addition, cellular composition of blood samples (proportions of lymphocytes, monocytes, and granulocytes, adjusted for exposure to labour at birth (any/none)) and bisulphite conversion batch were also considered in the multivariable linear regression model to determine if they altered any findings.
Results
The distribution of cohort characteristics is shown in , and the distribution of methylation is shown in . There was a moderate negative correlation between HIF3A.1 and HIF3A.2 average methylation at birth (r = −0.17, p = 0.006, Supp. Tables 2 and 3). There was no evidence of an association between birth or 12-month average HIF3A.1 methylation and any of the four-year weight or cardiovascular measures (Supp. Table 4). There was modest evidence that HIF3A.2 methylation was positively associated with systolic blood pressure (r = 0.12, p = 0.03) in the correlation analysis (). When stratified by sex, there was some evidence for a relationship between HIF3A.2 methylation and both systolic (r = 0.16, p = 0.03) and diastolic (r = 0.16, p = 0.03) blood pressure in males, but not females (). In linear regression modelling, none of the prenatal maternal factors appeared to confound this relationship, and similarly, adjusting for birth outcomes or SNP genetic covariates did not improve the fit of the model or alter the effect size of HIF3A.2 methylation on systolic blood pressure, with the exception of birth weight (z-score), which modestly increased the methylation coefficient and improved the fit of the model. BMI at 4 years was associated with systolic blood pressure, but adjusting for BMI did not attenuate the association between methylation and systolic blood pressure ().
Table 2. Correlations between cord blood HIF3A.2 methylation and cardiovascular and weight measures at 4 years
Table 3. Correlations between cord blood HIF3A.2 methylation and cardiovascular and weight measures at 4 years, stratified by sex
Table 4. Final linear regression models with four-year blood pressure as outcome, unadjusted and adjusted models in both combined-sexes and sex-stratified analysis
Figure 2. Distribution of methylation of individual CpG units and the average methylation across HIF3A.1 in cord blood and 12-month blood and HIF3A.2 in cord blood. Error bars are mean ± standard deviation
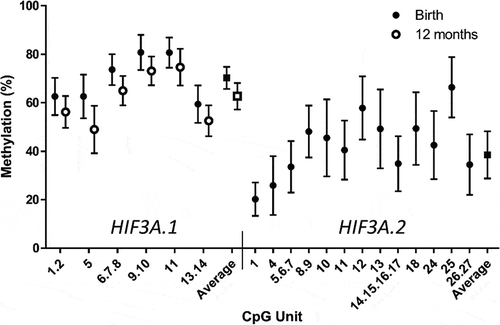
In sensitivity analyses, methylation of most, but not all, individual HIF3A.2 CpG units were positively associated with systolic blood pressure, while several individual HIF3A.2 CpG units were also positively associated with diastolic blood pressure (Supp. Table 5). There was no evidence for individual CpG units in HIF3A.2 or in HIF3A.1 at birth or 12 months associating with other 4-year cardiovascular measures (data not shown). Adjustment for bisulphite conversion batch, cellular composition of blood samples, and any exposure to labour did not alter the findings (data not shown).
Discussion
In this study, we investigated the potential for blood HIF3A methylation in early life to associate with four-year weight and cardiovascular measures. We found some evidence that higher HIF3A.2 methylation in cord blood correlates with higher systolic and diastolic blood pressure, primarily in males. This association persisted following adjustment for birth weight. To our knowledge, this is the first study to investigate the link between HIF3A methylation and cardiovascular health measures, and the first to report potential evidence of early life HIF3A methylation associating with health measures later in childhood. In light of previous findings for HIF3A.1, these findings suggest that methylation patterns at the two different HIF3A promoter regions may have differing relevance for cardiovascular and metabolic health outcomes.
We have found stronger evidence of HIF3A.2 methylation associating with blood pressure in males than females. While the potential relationship between DNA methylation and childhood blood pressure is currently uncharacterized, there are well-established sex differences in vascular and heart physiology and blood pressure regulation [Citation28]. Our findings may relate to this sexual dimorphism. However, it is important to note that the sex-stratified analysis is performed on a reduced sample size and as such, has greater potential to generate false positives, particularly when outlier values are included. Also, by including additional sex-stratified analysis the number of tests performed has increased, which should also be considered when interpreting the overall strength of evidence arising from association analyses.
There is considerable evidence that both systolic and diastolic blood pressure in childhood are predictive of cardiovascular risk in later life. In particular, elevated blood pressure in childhood is associated with increased risk of hypertension, metabolic syndrome [Citation4] and altered heart structure [Citation5] in adulthood. Based on our findings, it is unlikely that early life HIF3A.2 methylation has predictive utility in isolation, but could potentially improve prediction in combination with other predictive measures.
The effects of methylation on HIF3A gene expression are poorly characterized. There is evidence for HIF3A producing up to eight alternatively spliced transcripts across multiple promoter regions [Citation29]. Methylation of the HIF3A.1 promoter region has been reported to decrease total HIF3A expression in adipose tissue [Citation13], whereas no association between any HIF3A methylation probe and total expression was found in blood or fibroblasts [Citation30]. It is possible that HIF3A.2 methylation may relate to later blood pressure through regulation of specific HIF3A isoforms, rather than necessarily altering total expression levels, but current evidence linking promoter-specific methylation to HIF3A isoforms is limited. However, it has been shown that splice variants starting from the HIF3A.2 promoter region are more highly expressed in adult heart tissue compared to other organs, and also more highly expressed compared to splice variants starting from HIF3A.1 [Citation29]. As such, one or more splice variants starting from HIF3A.2 may potentially be involved in pathways regulating cardiac function or development. More studies investigating this aspect of HIF3A gene regulation are required in this regard.
This study is the first to investigate the association between early HIF3A methylation and measures of cardiovascular health in childhood, and one of the few to address methylation across multiple HIF3A promoter regions. We have also considered a range of potential confounders, including abnormal metabolic prenatal exposures, birth outcomes, and genetic variation. A limitation is missing data for individual CpG units, reducing the number of infants with complete methylation data, and missing data on some of the four-year cardiovascular measures reducing our sample size in some analyses, and consequently decreasing our power to detect more subtle effect sizes. There may also be additional unknown and unmeasured confounders. Replication of our findings in other longitudinal populations is warranted, with concomitant cardiovascular measures. Such measures at age 7 in BIS are currently underway, which will be valuable for testing the observed relationships later in childhood.
In conclusion, we provide some evidence for an association of cord blood methylation at a specific HIF3A promoter region with measures of four-year cardiovascular health independently of child anthropometry at birth and 4 years of age, with stronger evidence for a relationship in males. Our findings suggest the importance of considering promoter-specific HIF3A methylation status in broader association studies. Further evidence from paediatric and adult cohorts is required to characterize the extent to which earlier HIF3A methylation might be associated with later cardiovascular health throughout the life course and also to understand the potential underlying functional mechanisms.
Barwon Infant Study Investigator Team
The members of the Barwon Infant Study Investigator Team are the following: Peter Vuillermin and Fiona Collier, Barwon Health, Deakin University, the Murdoch Children’s Research Institute; Anne-Louise Ponsonby, John Carlin, Katie Allen, Mimi Tang, Richard Saffery, Sarath Ranganathan, and David Burgner, the Murdoch Children’s Research Institute, University of Melbourne; Terry Dwyer, the Murdoch Children’s Research Institute and the George Institute for Global Health; and Peter Sly, University of Queensland, Queensland Children’s Medical Research Institute.
Supplemental Material
Download Zip (60.6 KB)Acknowledgments
We thank QIMR Berghofer Medical Research Institute for their role in coordinating the genotyping of BIS samples.
Disclosure statement
No potential conflict of interest was reported by the authors.
Supplemental material
Supplemental data for this article can be accessed here.
Additional information
Funding
References
- Gluckman PD, Hanson MA. The developmental origins of the metabolic syndrome. Trends Endocrinol Metab. 2004;15(4):183–187.
- Kelly RK, Thomson R, Smith KJ, et al. Factors affecting tracking of blood pressure from childhood to adulthood: the childhood determinants of adult health study. J Pediatr. 2015;167(6):1422–1428. e2.
- Theodore RF, Broadbent J, Nagin D, et al. Childhood to early-midlife systolic blood pressure trajectories: early-life predictors, effect modifiers, and adult cardiovascular outcomes. Hypertension. 2015;66(6):1108–1115.
- Sun SS, Grave GD, Siervogel RM, et al. Systolic blood pressure in childhood predicts hypertension and metabolic syndrome later in life. Pediatrics. 2007;119(2):237–246.
- Lai -C-C, Sun D, Cen R, et al. Impact of long-term burden of excessive adiposity and elevated blood pressure from childhood on adulthood left ventricular remodeling patterns: the Bogalusa heart study. J Am Coll Cardiol. 2014;64(15):1580–1587.
- Hong YM. Atherosclerotic cardiovascular disease beginning in childhood. Korean Circ J. 2010;40(1):1–9.
- McCloskey K, Vuillermin P, Ponsonby A-L, et al. Aortic intima-media thickness measured by trans-abdominal ultrasound as an early life marker of subclinical atherosclerosis. Acta Paediatr. 2014;103(2):124–130.
- Intapad S, Ojeda NB, Dasinger JH, et al. Sex differences in the developmental origins of cardiovascular disease. Physiology. 2014;29(2):122–132.
- Dasinger JH, Alexander BT. Gender differences in developmental programming of cardiovascular diseases. Clin Sci. 2016;130(5):337–348.
- Novakovic B, Saffery R. The importance of the intrauterine environment in shaping the human neonatal epigenome. Epigenomics. 2013;5(1):1–4.
- Gluckman PD, Hanson MA, Cooper C, et al. Effect of in utero and early-life conditions on adult health and disease. N Engl J Med. 2008;359(1):61–73.
- Dengler VL, Galbraith MD, Espinosa JM. Transcriptional regulation by hypoxia inducible factors. Crit Rev Biochem Mol Biol. 2014;49(1):1–15.
- Dick KJ, Nelson CP, Tsaprouni L, et al. DNA methylation and body-mass index: a genome-wide analysis. Lancet. 2014;383(9933):1990–1998.
- Pan H, Lin X, Wu Y, et al. HIF3A association with adiposity: the story begins before birth. Epigenomics. 2015;7(6):937–950.
- Richmond RC, Sharp GC, Ward ME, et al. DNA methylation and BMI: investigating identified methylation sites at HIF3A in a causal framework. Diabetes. 2016;65(5):1231–1244.
- Mansell T, Ponsonby A-L, Januar V, et al. Early-life determinants of hypoxia-inducible factor 3A gene (HIF3A) methylation: a birth cohort study. Clin Epigenetics. 2019;11(1):96.
- Davis EF, Lazdam M, Lewandowski AJ, et al. Cardiovascular risk factors in children and young adults born to preeclamptic pregnancies: a systematic review. Pediatrics. 2012;129(6):e1552-e1561. e1552-e1561.
- Kajantie E, Eriksson JG, Osmond C, et al. Pre-eclampsia is associated with increased risk of stroke in the adult offspring: the Helsinki birth cohort study. Stroke. 2009;40(4):1176–1180.
- Leybovitz-Haleluya N, Wainstock T, Landau D, et al. Maternal gestational diabetes mellitus and the risk of subsequent pediatric cardiovascular diseases of the offspring: a population-based cohort study with up to 18 years of follow up. Acta Diabetol. 2018;55(10):1037–1042.
- Vuillermin P, Saffery R, Allen KJ, et al. Cohort Profile: the Barwon infant study. Int J Epidemiol. 2015;44(4):1148–1160.
- McCloskey K, Ponsonby A-L, Carlin JB, et al. Reproducibility of aortic intima-media thickness in infants using edge-detection software and manual caliper measurements. Cardiovasc Ultrasound. 2014;12(1):18.
- Collier FM, Tang MLK, Martino D, et al. The ontogeny of naïve and regulatory CD4+ T-cell subsets during the first postnatal year: a cohort study. Clin Transl Immunology. 2015;4(3):e34.
- Cole TJ, Williams AF, Wright CM. Revised birth centiles for weight, length and head circumference in the UK-WHO growth charts. Ann Hum Biol. 2011;38(1):7–11.
- Haertle L, El Hajj N, Dittrich M, et al. Epigenetic signatures of gestational diabetes mellitus on cord blood methylation. Clin Epigenetics. 2017;9(1):28.
- Nankervis A, McIntyre HD, Moses RG, et al. Testing for gestational diabetes mellitus in Australia. Diabetes Care. 2013;36(5):e64.
- Tranquilli A, Dekker G, Magee L, et al. The classification, diagnosis and management of the hypertensive disorders of pregnancy: a revised statement from the ISSHP. Pregnancy Hypertens. 2014;4(2):97.
- Pink B. Socio-economic indexes for areas (SEIFA) 2011. Canberra: Australian Bureau of Statistics; 2013.
- Mendelsohn ME, Karas RH. Molecular and cellular basis of cardiovascular gender differences. Science. 2005;308(5728):1583–1587.
- Pasanen A, Heikkilä M, Rautavuoma K, et al. Hypoxia-inducible factor (HIF)-3alpha is subject to extensive alternative splicing in human tissues and cancer cells and is regulated by HIF-1 but not HIF-2. Int J Biochem Cell Biol. 2010;42(7):1189–1200.
- Gutierrez-Arcelus M, Lappalainen T, Montgomery S, et al. Passive and active DNA methylation and the interplay with genetic variation in gene regulation. elife. 2013;2:e00523.